ABSTRACT
Verticillium dahliae, the causal agent of Verticillium wilt of strawberry, is a major soilborne pathogen in California strawberry production. Recent shifts in disease management have necessitated alternative methods for disease control. Two field experiments were conducted to examine the efficacy of sequential fumigant applications for crop termination and bed fumigation to reduce Verticillium dahliae inoculum density in a naturally infested field. Fumigant efficacy was evaluated for crop termination and for reducing pathogen survival in host tissue. Crop termination was conducted using metam potassium and metam sodium. Bed fumigation was conducted using both metam products and chloropicrin. For plots that were subject to both crop termination and bed fumigation, inoculum levels were somewhat reduced (3–6 CFU/g of soil) but not to a level below the disease threshold for strawberry (2 CFU/g of soil). Plots subjected to crop termination with metam potassium and bed fumigation with chloropicrin resulted in the lowest V. dahliae inoculum density. Injury due to crop termination ranged from 53% to 77% at 7 days after fumigation (DAF) and 73–89% at 14 DAF. The odds ratio of V. dahliae survival in the terminated crop were lower than in crops not terminated and V. dahliae survival was less likely at 14, compared to 7 DAF. In the crop terminated with metam potassium, the odds ratio of pathogen survival in the petiole versus the crown was 0.9–1.4. This research shows that crop termination could constitute management strategies for Verticillium wilt of strawberry.
Introduction
Strawberry (Fragaria × ananassa, Duch.) is an important crop in California, with 15,782 ha harvested in 2021 resulting in a farm gate value of $3.02 billion (California Department of Food and Agriculture, Citation2021). In 2020, California strawberry production accounted for more than 85% of national strawberry production, with most production occurring in fumigated soils due to the threat of soilborne pathogens (Njoroge et al., Citation2009). V. dahliae affects many high-value cropping systems in the world, such as lettuce, strawberry, and tomato (Bhat and Subbarao, Citation1999; Lopez-Escudero and Blanco-Lopez, Citation2001). V. dahliae can survive as microsclerotia and persist for up to 14 years in soil without a host (Wilhelm, Citation1958). V. dahliae microsclerotia are more persistent in sandy loam soils that are common to California strawberry production compared to other soil types such as clay loam (Wilhelm, Citation1966, Citation1998). These dark-colored and resistant structures can also survive in colonized tissues and remain in the soil upon crop decomposition (Subbarao et al., Citation2007). Upon contact with strawberry roots, V. dahliae microsclerotia germinate and colonize the xylem, resulting in plant collapse and death (Beckman and Roberts, Citation1995). Strawberry is extremely sensitive and vulnerable to infection by V. dahliae, with levels as low as 2 CFU/g of soil being sufficient for plant infection (Harris and Yang, Citation1996). Due to the wide host range of V. dahliae, the common crop rotation strategy between strawberry and lettuce enables the pathogen to persist and increase over time (Mihail, Citation1992). Control of Verticillium wilt of strawberry is important for the continued economic viability of the California strawberry industry due to its impact on plant health, yield, and revenues (Zavatta et al., Citation2021). Considering the disease cycle, reduction of the pathogen in infected current season plants and in soil is crucial to attain adequate control of the pathogen.
Predictably, diseases caused by soilborne pathogens, such as Verticillium wilt, have increased and are more difficult to control after the methyl bromide (Mb) phaseout (Klose et al., Citation2007). 1,3-dichloropropene (1,3-D), chloropicrin (Pic), dazomet (Dz), metam sodium (Ms; sodium N-methyldithiocarbamate), and metam potassium (Mp; potassium N-methyldithiocarbamate) have been utilized in place of Mb (Brannen and Cline, Citation2021). Alternative fumigants have shortcomings of incomplete control due to their intrinsic chemical properties that limit their movement through the soil (both lateral and vertical) and into lignified tissues (Baggio et al., Citation2018; Chamorro et al., Citation2016; Porter et al., Citation2004). With this, management strategies to reduce inoculum in the soil and in plant tissues and debris necessitate investigation, especially since high-valued strawberries are often cropped consecutively or have limited crop rotation timelines (Mihail, Citation1992).
Crop termination has been utilized by strawberry growers at end-of-season to kill weeds and facilitate plastic removal (Palhano et al., Citation2018). The utilization of paraquat in this method does not provide control of soilborne pathogens like V. dahliae due to its lack of fungicidal properties (Noling, Citation2006). However, the use of soil fumigants such as Mp and Ms provide broad spectrum control of pathogens while also killing the infected crop (Khatri et al., Citation2020). In strawberry, although crop termination alone has been investigated, the use of crop termination combined with bed fumigation to control V. dahliae is yet to be examined.
The utilization of metam products like Mp in a crop termination application was observed to adequately kill infected strawberry plants and reduce Macrophomina phaseolina inoculum (Khatri et al., Citation2020). Owing to the high-water solubility of Mp, it is well-suited for drip-irrigation (Chamorro et al., Citation2016). When examining the efficacy of Mp to reduce soilborne pathogens, Chamorro et al. demonstrated that an application of Mp had statistically similar pathogen reduction rates to Mb in reducing M. phaseolina survivability when applied via drip irrigation (Citation2016).
Methyl isothiocyanate (MITC) is the active ingredient of Mp and Ms in soil. Ms has been used since the 1950s due to its rapid degradation into MITC (Kreutzer, Citation1963); the use of Ms has been limited due to the lack of mobility in soil compared to that of Mb (Ajwa et al., Citation2002; Tomlin, Citation1994).
Preplant soil fumigation, specifically bed fumigation, has been widely used by strawberry growers and in annual production systems, becoming a common disease management practice. Almost all of conventional strawberry production worldwide occurs in fumigated soils (Ajwa et al., Citation2002) and annual applications have contributed to the control of soil-borne pathogens in California (Guthman, Citation2019; Subbarao et al., Citation2007). Pic has been used since the 1920s and is still in use, providing excellent control of fungal pathogens (Duniway, Citation2002). Crop termination can be used to reduce the pathogen inoculum going into the soil whereas bed fumigation could reduce inoculum level in soil.
Therefore, the main goal of this study was to evaluate the effectiveness of sequential applications of crop termination and bed fumigation on reducing inoculum of V. dahliae in soil and decreasing pathogen survival in the crop. The objectives were (1) to assess the efficacy of crop termination alone, bed fumigation alone, and the sequential applications of these methods to reduce V. dahliae soil inoculum levels, (2) to compare the efficacy of different fumigant chemicals, (3) to visually assess the degree of crop injury due to crop termination, and (4) to assess the survival of V. dahliae in the terminated crop.
Materials and Methods
Experimental Location and Design
Two field trials were established at California Polytechnic State University, San Luis Obispo, field 25, block 6 (35°30’ N, 120°67’ W). Trial 1 occurred during the 2020–2022 growing seasons. Trial 2 occurred during the 2021–2023 growing seasons. Soil in the trial sites, characterized as Salinas silty clay loam (38% clay, 36% sand, 26% silt; California Soil Resource Lab, Citation2022), had a pH of 7.2, electrical conductivity of 2.2 dS/m, cation exchange capacity of 20.5 meq/100 g, and an organic matter composition of 2.9% (A & L Western Agricultural Laboratories, Modesto, CA). Prior to the beginning of trial 1, lettuce (cv. Black Seeded Simpson) was broadcasted in June 2020, maintained with overhead sprinkler irrigation and disked in September 2020 to increase pathogen inoculum. Lettuce crop was symptomatic and showed over 75% mortality.
The experimental sites for both trials were 0.1 ha each and located within the same block, separated by 20 m. The strawberry crop for both trials was established in 12 industry standard beds. Each bed measured 1.1 m between row centers and 0.3 m high. Bed lengths were 90 m and 54 m for trials 1 and 2, respectively. Beds were formed on 14 October 2020 and 16 October 2021 for trials 1 and 2, respectively. Beds were covered with polyethylene mulch, which was 2.1 m wide, 1.1 mil thick, and completely black (Ginegar, Ozgard Black T-Plus, Israel). Bare-root strawberry transplants of public cultivar Seascape (Lassen Canyon Nursery, Redding, CA), which is highly susceptible to Verticillium wilt, were planted on 2 November 2020 and 2 November 2021 for trials 1 and 2, respectively, in four rows of plants per bed, separated by 0.2 m. Prior to transplant, the strawberry transplants were stored at 1°C for approximately 2 weeks and were not subjected to supplemental chill by the nursery. Three lines of 0.5 mm drip-tape (low-flow, 1.2 L/min/30.4 m at 55 kPa, with 20 cm spacing on emitters; Tri-Cal®, Hollister, CA) spaced 0.4 m apart and at a depth of 15 mm were used. For trial 1, a total of 930 plants were established in each bed. For trial 2, a total of 560 plants were established in each bed. Irrigation, fertilization, and the management of other pests were conducted according to standard industry practices.
The experiment was a split-plot design with the main plot being the fumigant and the subplot being the application method (Supplementary Figure S1 and Supplementary Figure S2). To apply fumigants and application method treatments, a drip irrigation system was used. Due to logistical constraints, the same fumigant had to be applied on the same bed. By-pass tubes were used to apply specific application method to different plots of the same bed. For trial 1, each bed consisted of three plots (30 m per plot) with 310 plants per plot. For trial 2, each bed consisted of three plots (18 m per plot) with 185 plants per plot. Each plot was characterized as either receiving only crop termination, only bed fumigation, or both treatments. Three non-treated control beds (90 m in length for trial 1, 930 plants; 54 m in length for trial 2, 560 plants) were included.
Crop Termination and Bed Fumigation
For crop termination, fumigants Mp (AMVAC, Newport Beach, CA) and Ms (AMVAC, Newport Beach, CA) were utilized whereas for bed fumigation Mp, Ms, and Pic (Tri-Clor EC, TriCal, Hollister, CA) were utilized. There were more plots terminated with Mp than Ms since plots assigned Pic could not be terminated with this chemistry as it is not registered for use as a crop termination fumigant in the state of California and thus were assigned Mp. The description of the applications and treatments are provided in . Following the crop termination treatment, plastic and drip tape from the experimental beds were removed by hand. In trial 1, the upper 13 cm of the soil profile in each bed was tilled by hand to incorporate plant debris. In trial 2, a hydraulic rototiller (Barreto 918 H, Barreto Manufacturing Inc., La Grande, OR) was employed to reduce manual labor, and plant debris was incorporated into the soil profile at the same depth as trial 1. Drip tape and plastic (total impermeable film, Ginegar, Ozgard Black T-Plus, Israel) were then laid by hand prior to the bed fumigation treatment.
Table 1. Applications and treatments applied to soil by drip irrigation during the 2020–22, and 2021–23 growing seasons in San Luis Obispo, CA.
Crop Termination
For trial 1, Mp and Ms were applied 238 days after transplanting on 28 June 2021 when the crop experienced 5% mortality and 45% symptom expression. Mp was injected via drip irrigation using existing three drip tapes at 439.6 L/ha over a 50 min injection period. Ms was injected at 523.8 L/ha over a 30 min injection period.
For trial 2, Mp and Ms were applied 241 days after transplanting on 1 July 2022 when the crop experienced 2% mortality and 20% symptom expression. Mp and Ms were applied as mentioned previously.
Bed Fumigation
At the beginning of the 2021–22 season for trial 1, bed fumigation was applied on 18 October 2021, 112 days after termination and 15 days before transplanting of the next strawberry crop. Mp was injected via drip irrigation using three drip tapes at 580.0 L/ha over a 90 min injection period. Ms was injected at 701.6 L/ha over a 90 min injection period. Pic was injected at 203.0 L/ha over a 40 min injection period.
At the beginning of the 2022–23 season for trial 2, bed fumigation was applied on 12 August 2022, 42 days after termination and 125 days before transplanting of the next strawberry crop. Mp was injected via drip irrigation using three drip tapes at 580.0 L/ha over a 60 min injection period. Ms was injected at 701.6 L/ha over a 60 min injection period. Pic was injected at 203.0 L/ha over a 30 min injection period.
Quantification of V. dahliae Density
Soil inoculum density of V. dahliae was determined seven days before transplanting, after the crop termination application, and after the bed fumigation application. A total of ten soil samples were collected per bed in a zig-zag pattern at 0.3 m depth using a 0.03 m soil-core, with samples separated by 3 m. Soil samples were pooled, air-dried for two weeks, pulverized with a mortar and pestle, and 0.1 g of soil in ten replicates were plated on a semi-selective medium, Sorenson’s NP-10 (Sorensen et al., Citation1991), with use of an Andersen sampler (Andersen, Citation1958). Colony forming units (CFU) were counted using a dissecting microscope at 10X (Olympus, Tokyo, Japan) magnification after two weeks of dark incubation in ambient temperature (13–24°C). Following the crop termination and bed fumigation treatments, soil was sampled 10 days after crop termination and 14 days after bed fumigation from each subplot and within the middle 15 m of the plot along the outer (lines I and IV) and inner (lines II and III) drip tapes. Soil collected from the outer and inner lines were pooled separately prior to enumeration. Non-treated control beds were also sampled from the middle 15 m of the bed. Soil inoculum density of V. dahliae was determined as mentioned previously.
V. dahliae Molecular Identification
A total of 15 isolates of putative V. dahliae were selected for species identification with molecular techniques. Genomic DNA extraction was performed on the isolates following the protocol of Bok and Keller (Citation2012). The ITS region was PCR amplified with primers ITS1 and ITS4 (White et al., Citation1990) following thermal cycles: an initial denaturation at 95°C for 3 min, followed by 36 cycles of 95°C for 30 s, 54°C for 45 s, and 72°C for 45 s, and a final extension at 72°C for 5 minutes. PCR products were then purified with ExoSAP-IT Express (Applied Biosystems) and sequenced at MCLAB (San Francisco, CA). Sequences were aligned using ClustalW in BioEdit v7.0.5.3 (Hall, Citation1999) and the ITS region of the 15 isolates appeared to be identical. The sequence was searched with BLAST in NCBI for species identification. Based on BLASTn searching results, the ITS region of the 15 isolates obtained in this study was identical with isolates identified as V. dahliae in GenBank. The ITS Region of one isolate was deposited in GenBank and assigned the accession number OP131837.
Determination of Plant Injury and Pathogen Survival After Crop Termination
Following crop termination, plant injury was visually assessed 7 and 14 days after fumigation (DAF). A total of 100 plants from the middle 15 m of each subplot were visually assessed on a 0–4 scale: 0 = no mortality, no wilting, no necrotic plant parts; 1 = 25% mortality, minimal wilting, half of plant parts not necrotic; 2 = 50% mortality, complete wilting, half of plant parts necrotic; 3 = 75% mortality, complete wilting, plant parts necrotic besides apical meristem; and 4 = 100% mortality, complete wilting, all plant parts necrotic. Further, V. dahliae survival in the terminated crop was examined at 7 and 14 DAF. Petiole and crown tissue of ten plants from the middle 15 m of each subplot were sampled, surface disinfected, and plated on NP-10 semi-selective medium, incubated in darkness at ambient temperature (13–24°C) for 10–14 days, and observed under a dissecting microscope for the presence of V. dahliae microsclerotia.
Data Analyses
Statistical analyses were performed in R 4.2.2 (R Core Team, Vienna, Austria). Data were analyzed separately for trial 1 and trial 2.
Effect of Crop Termination On V. dahliae Soil Population Density
Normality of the V. dahliae soil population density data was assessed using the Shapiro Wilk test in R with α = 0.05. Normality was not confirmed for both trial 1 and trial 2 data; thus, the nonparametric Kruskal–Wallis test was used to compare mean V. dahliae population densities. If a significant difference was found with α = 0.05, a post-hoc Wilcoxin signed-rank test with continuity correction was performed to identify differences. Investigation into differences between crop termination chemistries on V. dahliae soil population densities and between the inner and outer lines were performed separately.
Effect of Both Crop Termination and Bed Fumigation On V. dahliae Soil Population Density
Following the bed fumigation application, the soil CFU data were analyzed in a generalized linear mixed model with a negative binomial distribution and a log link function using the lme4 package in R (Bates et al., Citation2015), due to overdispersion of the data. Prior to evaluation, CFU count data were averaged over lines (lines I/IV and II/III). A nested treatment structure between the fumigant and fumigation application type were used as fixed effects; block and block by fumigant were included as random effects to account for the split-plot experimental design. Least square means were used with the emmeans package in R (Searle et al., Citation2012) to determine significant differences between both the application type and fumigant and the fumigant itself. A Tukey p-adjustment was utilized to control for type I error rates due to the multiple comparisons. A separate analysis was conducted to evaluate possible differences in pathogen density between the inner and outer lines.
Evaluation of Crop Injury Due to Crop Termination Fumigation
Data was analyzed to determine if a significant difference in visual injury was found (1) between the two fumigant types; and (2) at 7 and 14 DAF. Normality of the injury data was assessed using the Shapiro Wilk test in R with α = 0.05 and was not confirmed for both trials 1 and 2 data. Thus, the nonparametric Kruskal-Wallis test was used to compare the mean injury levels. If a significant difference was found with α = 0.05, a post-hoc Wilcoxin signed-rank test was performed with a Benjamini-Hochberg adjusted P-value in avoidance of false discovery.
Determination Of V. dahliae Survival in Crop Tissue After Crop Termination Fumigation
The pathogen survival data were analyzed in a multilevel logistic regression model using lme4 in R (Bates et al., Citation2015) to establish the likelihood of (1) pathogen survival (binary variables with 0 for surviving and 1 for not surviving) in terminated crop at both 7 and 14 DAF; (2) pathogen survival in the crown tissue of terminated crop; (3) pathogen survival in the petiole tissue of terminated crop; and (4) pathogen survival in the crown and petiole tissue of the terminated crop treated with Mp or Ms.
Results and Discussion
Plant Injury Due to Crop Termination
For trial 1, the overall average plant injury due to the uptake of the fumigants for 7 and 14 days after fumigation (DAF) was 77% and 89%, respectively. For crop terminated with Mp, the average plant injury at 7 DAF and 14 DAF was 77% and 87%, respectively. For crop terminated with Ms, the average plant injury at 7 DAF and 14 DAF was 77% and 90%, respectively. There was no significant difference in the efficacy of Mp and Ms on crop injury at 7 DAF (X2 = 0.24, df = 1, P = .62). After 14 days, the most effective fumigant was Mp in terms of inducing higher injury to the crop (X2 = 8.3, df = 1, P = .0039).
For trial 2, the overall average plant injury for 7 and 14 days after fumigation (DAF) was 53% and 73%, respectively. For crop terminated with Mp, the average plant injury at 7 DAF and 14 DAF was 56% and 75%, respectively. For crop terminated with Ms, the average plant injury at 7 DAF and 14 DAF was 50% and 70%, respectively. Termination with Mp resulted in a significantly higher crop injury at 7 DAF (X2 = 17.0, df = 1, P ≤.001) compared to Ms. Consistent with trial 1, after 14 days, the most effective fumigant was Mp in terms of inducing higher injury to the crop (X2 = 16.4, df = 1, P ≤.001).
For both trials, significant differences in visual plant injury assessments were found at 14 DAF. At 14 DAF, crop terminated with Mp had significantly higher plant injury levels compared to crop terminated with Ms. It is known that as plants are subject to the fumigant and lack of irrigation over time, injury is suspected to be higher at 14 DAF. Khatri et al. (Citation2020) demonstrated 100% crop injury at 14 DAF with high fumigant rates of Mp (195–975 L/ha) and > 90% crop injury at 14 DAF with lower fumigant rates (65–325 L/ha). For our trials, Mp was applied at 439.6 L/ha and Ms was applied at 523.8 L/ha, demonstrating that fumigant rates between 400 and 600 L/ha were adequate to provide between 70% and 90% crop injury at 14 DAF in both years of the trial. Although economic analyses have not been conducted, identifying sufficient rates of fumigant inputs in future research is an important consideration for growers; if less fumigant is needed to provide crop injury and successful pathogen eradication, this could result in less of an economic burden to growers as well as the environment.
Pathogen Survival in Terminated Crop
The mean survival of V. dahliae in the crown and petiole tissue after crop termination for both trials are summarized in . With all other predictors held constant, the odds of V. dahliae survival in trial 1 were four times less in crops terminated than crops not terminated (). In the non-terminated crop, there was no significant difference between the likelihood of V. dahliae survival in crown versus petiole (β = 0.20, Z = 0.34, P = .73). In crop terminated with Mp, there was a significant difference between likelihood of V. dahliae survival in crown and petiole, with the pathogen more likely to survive in the petiole (β = 1.4, Z = 0.52, P ≤.001). In crop terminated with Ms, the same results apply; V. dahliae was more likely to survive in the petiole than the crown (β = 1.7, Z = 0.61, P < .001). Over time, there was a significant difference in V. dahliae survival at 7 DAF and 14 DAF, with 14 DAF having a significantly lower level of V. dahliae survival (β = 2.0, Z = 0.49, P ≤.001). There was no significant difference between the efficacy of the two fumigants over time (β = 0.57, Z = 0.41, P = .17). There was a significant difference in V. dahliae survival in crop tissue over time, with the pathogen more likely to survive in the petiole than the crown at 14 DAF (β = 1.2, Z = 2.6, P = .0078).
Figure 1. The odds ratio of Verticillium dahliae survival in terminated crop for trial 1. With all other predictors held constant, the odds ratio represents the likelihood of pathogen survival after a particular exposure (crop termination). Negative binomial generalized linear model regression (α = 0.05). n = 3.
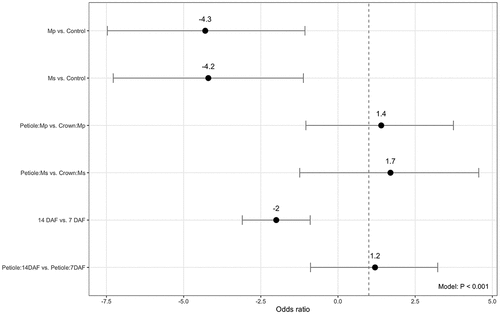
Table 2. Trials 1 and 2 post crop termination pathogen survival in host tissue 7 and 14 days after fumigation (DAF). % positive indicates samples showing Verticillium dahliae microsclerotia on a semi-selective medium, NP-10. ± represent standard error of the mean. n = 3.
Our findings in trial 2 corroborated the results from trial 1 for overall V. dahliae survival. With all other predictors held constant, the odds of V. dahliae survival in trial 2 were less in crops terminated than crops not terminated (). In the non-terminated crop, there was no significant difference between the likelihood of V. dahliae survival in crown versus petiole (β = 0.19, Z = 0.46, P = .64). In crop terminated with Mp, there was a significant difference between likelihood of V. dahliae survival in crown and petiole, with the pathogen more likely to survive in the petiole (β = 0.98, Z = 2.2, P = .031). In crop terminated with Ms, there was no significant difference between the likelihood of V. dahliae survival in crown and petiole (β = 0.77, Z = 1.7, P = .098). Over time, there was no significant difference in V. dahliae survival at 7 DAF and 14 DAF (β = 0.60, Z = 1.5, P = 0.14). There was not a significant difference in V. dahliae survival in crop tissue over time, whether that be in the petiole or the crown (β = −0.24, Z = −0.71, P = .48).
Figure 2. The odds ratio of Verticillium dahliae survival in terminated crop for trial 2. With all other predictors held constant, the odds ratio represents the likelihood of pathogen survival after a particular exposure (crop termination). Negative binomial generalized linear model regression (α = 0.05). n = 3.

Although visual assessments of crop injury are adequate for evaluating the effects of fumigants in a crop termination application, whether the pathogen survived inside the terminated crop tissue needs to be examined. Our study suggests that the odds of pathogen survival at 14 DAF is lower compared to 7 DAF. This is an important consideration, for growers need to be cognizant of re-plant intervals and the allowance for the fumigants to terminate the crop accordingly. The survival of V. dahliae in the terminated crop tissue visually correlates to assessments of plant injury, both at 7 DAF and 14 DAF. We find that pathogen survival is less likely at 14 DAF, and that the pathogen is more likely to survive in the petioles of crops terminated with Mp in comparison to the crowns. There was no consistency between trials in the odds of pathogen survival in plants terminated with Ms. As the plants actively uptake the fumigants, we assume that the crop tissue would be destroyed and translocation of the fumigants to other parts of the plant such as the petioles and leaves may be hindered. Therefore, survival of V. dahliae in the petiole may lead to an increase in inoculum for the next season. These findings further illustrate that waiting 14 days after termination lowers the chances of V. dahliae survival and may lead to lower amounts of inoculum being incorporated upon decomposition.
It is known that Verticillium wilt symptoms develop rather quickly within infected strawberry plants, as the pathogen produces conidia that move upwards in the xylem of the plant tissue after one or more cortical infections and as the plant declines, V. dahliae can proliferate more extensively in the water conducting tissue and produce microsclerotia in the decomposing crop residue (Gordon and Subbarao, Citation2007). Since the fumigants applied in a crop termination setting rely on translocation through water conducting tissues, crops with more severe infections or increased symptoms could have less movement of the fumigants through the plant tissues. Future research should be conducted to determine the correlation between Verticillium wilt incidence, severity and the efficacy of crop termination. The timing of crop termination is important for growers due to monetary concerns, as growers are unlikely to kill the crop prematurely for the sake of pathogen control. Along the California Central Coast, peak harvest occurs in May to July (Bolda et al., Citation2021) and the timing of crop termination with peak harvest is a concern. Since growers prefer to wait until season end for crop termination, this waiting period could increase inoculum, reducing the efficacy of pathogen control by crop termination and providing the pathogen increased momentum for successive seasons.
The lack of full control of V. dahliae could be attributed to the degree to which the crop was infested at the time of crop termination. It is hypothesized that terminating the crop at lower symptom expression rates could lead to more plant injury and less pathogen survival in plant tissue; although our study shows that lower symptom expression rates in trial 2 did not lead to less chances of pathogen survival after termination. With this, pathogen pressure was less in trial 2 versus trial 1 so we are unable to conclude that terminating the crop earlier in symptom expression could lead to less chances of V. dahliae survival.
Pathogen Density in Soil After Crop Termination
The overall pre-plant soil inoculum density of V. dahliae for trial 1 was 7.0 ± 2.4 CFU/g. Following crop termination, soil inoculum density significantly decreased in beds treated versus non-treated control beds (X2 = 20.2, df = 2, P ≤.001). In non-treated control beds, inoculum density increased from 7.0 ± 2.4 CFU/g to 15.0 ± 2.7 CFU/g over season duration (). In plots only subjected to crop termination, inoculum density decreased from 7.0 ± 2.4 CFU/g to 5.1 ± 0.7 CFU/g (). For plots subjected to crop termination with Mp, V. dahliae inoculum density decreased from 9.5 ± 1.5 CFU/g to 5.7 ± 0.4 CFU/g. For plots subjected to crop termination with Ms, V. dahliae inoculum density decreased from 5.3 ± 1.6 CFU/g to 4.8 ± 0.5 CFU/g. There was no significant difference in pathogen density between plots treated with Mp or Ms (P = 0.87). There was no significant difference overall in pathogen density between the outer and inner plant rows (P = .89).
Figure 3. Soil inoculum density of Verticillium dahliae for the non-treated control beds at different timepoints during the fumigation series. PCT denotes after the crop termination treatment. PBF denotes after the bed fumigation treatment. Error bars represent standard error of the mean. n = 3.
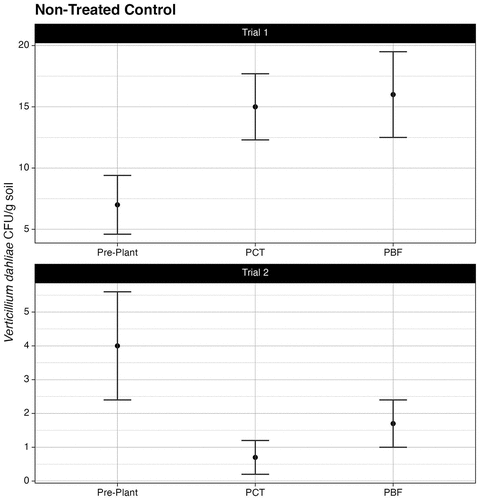
Figure 4. Soil inoculum density of Verticillium dahliae for all treatments, separated by fumigant type, at the conclusion of the fumigation series in trial 1. Negative binomial generalized linear model regression (α = 0.05). CT denotes plots only crop terminated. BF denotes plots only bed fumigated. CT+BF denotes plots sequentially treated with both methods. Error bars represent standard error of the mean. n = 3.
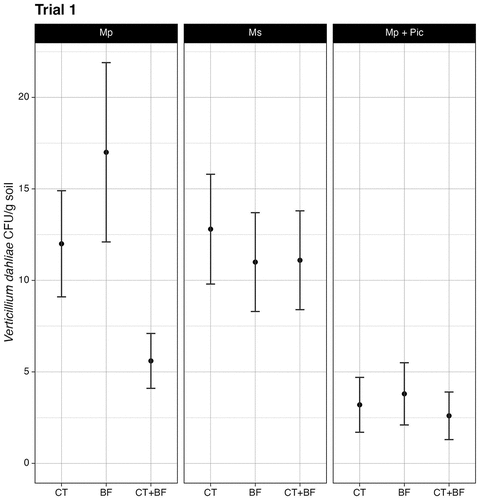
The overall pre-plant soil inoculum density of V. dahliae for trial 2 was 3.3 ± 0.7 CFU/g. Following crop termination, no significant decrease in inoculum density was found in beds treated versus non-treated control beds (X2 = 3.9, df = 2, P = 0.14). Unexpectedly, inoculum density decreased in non-treated control beds from 4.0 ± 1.6 CFU/g to 0.7 ± 0.5 CFU/g over the season (). In plots that were subject to only crop termination, inoculum density decreased from 3.0 ± 0.8 CFU/g to 1.8 ± 0.2 CFU/g (). For plots subjected to crop termination with Mp, V. dahliae inoculum density decreased from 3.0 ± 1.0 CFU/g to 1.4 ± 0.2 CFU/g. For plots subject to crop termination with Ms, V. dahliae inoculum density decreased from 3.0 ± 1.3 CFU/g to 2.6 ± 0.4 CFU/g. Consistent with trial 1, there was no significant difference in pathogen density for plots treated with Mp or Ms (P = 0.35) and there was no significant difference overall in pathogen density between the outer and inner plant rows (P = .34). In both years of the trial, crop termination was insufficient in reducing levels below the disease threshold (2 CFU/g) for strawberry (Harris and Yang, Citation1996).
Figure 5. Soil inoculum density of Verticillium dahliae for all treatments, separated by fumigant type, at the conclusion of the fumigation series in trial 2. Negative binomial generalized linear model regression (α = 0.05). CT denotes plots only crop terminated. BF denotes plots only bed fumigated. CT+BF denotes plots sequentially treated with both methods. Error bars represent standard error of the mean n = 3.
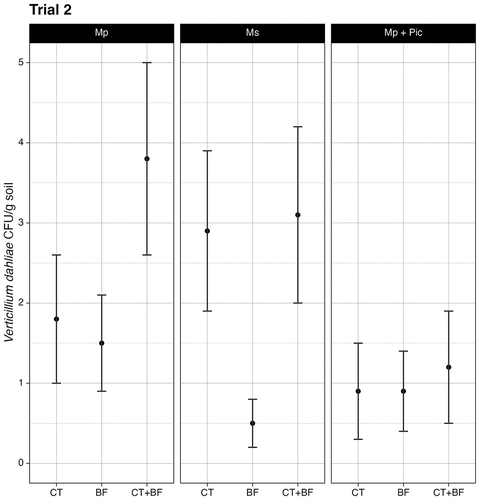
In trial 2, we observed a decrease in pathogen density in the non-treated control beds, which is not consistent with the findings in trial 1. This difference may be attributed to the field’s natural infestation with V. dahliae and how fungal population densities vary spatially within a single field. The heterogenous spatial distribution of V. dahliae microsclerotia within fields (Bejarano-Alcazar et al., Citation1995; Xiao et al., Citation1997) contributed to inconsistencies within trials. With a lower initial pathogen level in soil in trial 2, we did not observe treatment effects on pathogen density in soil. Our findings suggest that crop termination alone is not sufficient for lowering pathogen inoculum below the disease threshold, but it could lower pathogen pressure in fields that are heavily infested and serve as a potential tool in an integrative approach to disease management. Site-specific management methods may need to be implemented and investigations into spatial distributions of pathogen inoculum densities within California cropping systems is underway (Martin et al., Citation2019).
Pathogen Density in Soil After Both Crop Termination and Bed Fumigation Treatments
At the conclusion of both the crop termination and bed fumigation treatments, the estimated mean pathogen density for non-treated control plots in trial 1 was 16.0 ± 3.5 CFU/g (). Plots that only received treatments with Mp (10.5 ± 1.6 CFU/g) resulted in a significantly higher estimated mean pathogen density compared to plots sequentially treated with Mp and Pic (3.2 ± 1.1 CFU/g; Z = 2.8, P = .021). Plots sequentially treated with Mp and Pic had a significantly lower estimated mean pathogen density compared to non-treated control beds (Z = −3.9, P ≤.001) and compared to plots sequentially treated with Ms (11.6 ± 1.7 CFU/g; Z = −3.1, P = .012). No other significant differences were found between chemistries. Among plots that were only subject to treatment with Mp, plots that were bed fumigated with Mp (17.0 ± 4.9 CFU/g) had a significantly higher estimated mean pathogen density versus plots that were sequentially crop terminated and bed fumigated with Mp (; 5.6 ± 1.5 CFU/g; Z = 2.9, P = .010). No significant differences were found between other fumigants and treatments. No other plots had significant differences in mean pathogen density versus the non-treated control beds.
At the conclusion of the crop termination and bed fumigation treatments, the estimated mean pathogen density for non-treated control plots in trial 2 was 1.7 ± 0.7 CFU/g (). Among plots only subjected to treatment with Ms, plots that were only bed fumigated with Ms (0.49 ± 0.31 CFU/g) had a significantly lower estimated mean pathogen density versus plots that were only crop terminated with Ms (2.9 ± 1.0 CFU/g; Z = −2.7, P = .020). Plots that were only bed fumigated with Ms had a significantly lower estimated mean pathogen density versus plots that were sequentially crop terminated and bed fumigated with Ms (; 3.1 ± 1.1 CFU/g; Z = −2.8, P = .016). No significant differences were found between other fumigants and treatments.
For plots that were subject to both crop termination and bed fumigation, inoculum levels were somewhat reduced but not to a level that is below the threshold for strawberry. In trial 1, the overall pre-plant inoculum density for V. dahliae in the experimental plots was 7.0 ± 2.4 CFU/g and at the end of the fumigation series, plots that were sequentially crop terminated and bed fumigated resulted in an inoculum density of 6.4 ± 0.77 CFU/g. In trial 2, the overall pre-plant inoculum density for V. dahliae in the experimental plots was 3.3 ± 0.71 CFU/g and at the end of the fumigation series, plots that were sequentially crop terminated and bed fumigated resulted in an inoculum density of 2.8 ± 0.50 CFU/g. These findings show V. dahliae inoculum densities remaining somewhat constant even with sequential crop termination and bed fumigation applications.
This study demonstrates that sequential fumigation applications of crop termination and bed fumigation does not reduce V. dahliae populations in the soil below the threshold of infection for strawberry. In our study, the two trials had differences in crop symptom expression and levels of mortality in the susceptible “Seascape” crop prior to the crop termination application. In trial 1, 45% of our crop showed symptom expression before the crop termination treatment, with 5% of the crop being completely dead. In trial 2, 20% of our crop showed symptom expression before the crop termination treatment, with 2% of the crop being completely dead. Since plants that are completely necrotic and dead do not actively uptake metam fumigants due to occlusion of the vasculature by the pathogen (Street and Cooper, Citation1984), V. dahliae propagules inhabiting the crop tissue were not eradicated and were released into the soil upon decomposition of the plant material. These findings are consistent with work reported by Baggio et al. (Citation2021); remaining populations of M. phaseolina in soil also increased after crop termination and bed fumigation was not sufficient to reduce pathogen inoculum levels. Oscillations in pathogen density can be attributed to redistribution of microsclerotia in the soil profile due to tillage practices, which corresponds with findings from Chellemi et al. (Citation1997).
In general, both trials showed no significant differences between the various treatments that utilized the same fumigant, not demonstrating additive effects of crop termination and bed fumigation.
Pic has shown to provide effective control of V. dahliae in strawberry systems when applied via drip irrigation (Wilhelm and Koch, Citation1956) and our study also illustrates that Pic provides adequate control. A synergistic effect between mixtures of Mb and Pic has been reported (Wilhelm and Paulus, Citation1980; Wilhelm et al., Citation1961); our findings show that among plots treated with sequential crop termination and bed fumigation, those crops terminated with Mp and bed fumigated with Pic showed lower inoculum densities compared to plots sequentially treated with metam products. Future studies should examine the possible synergistic effect between Pic and other metam products such as Ms. There was no significant difference between Mp and Ms in decreasing soil inoculum in a crop termination or bed fumigation setting, which suggests that these two fumigants have similar chemistries and abilities to suppress V. dahliae populations.
Pathogen Density Between the Inner and Outer Lines After All Fumigation Treatments
For all treatments in trial 1, the estimated mean pathogen density for the inner and outer lines were 7.2 ± 0.85 CFU/g and 5.2 ± 0.65 CFU/g, respectively. For all treatments in trial 2, the estimated mean pathogen density for the inner and outer lines were 0.72 ± 0.19 CFU/g and 1.7 ± 0.32 CFU/g, respectively. This inconsistency between inner and outer line pathogen density between the trials could be due to the unequal placement of drip lines in the beds, inconsistencies of the drip irrigation system during the fumigant applications, or the tillage practices that were employed.
For plots that were subject to treatment with only Mp in trial 1, a significantly lower estimated mean pathogen density was found in the outer lines for plots only bed fumigated with Mp (; Z = −3.2, df = 1, P = .0015). No significant differences were found in estimated mean pathogen density between the inner and outer lines in plots only crop terminated with Mp (Z = −0.78, df = 1, P = .44) and in plots both crop terminated and bed fumigated with Mp (Z = −0.52, df = 1, P = .60). For plots that were subject to treatment with Mp in trial 2, no significant differences in estimated mean pathogen density were found between the inner and outer lines in plots only crop terminated with Mp (; Z = −0.80, df = 1, P = .42) and in plots only bed fumigated with Mp (Z = −0.30, df = 1, P = .76). In plots both crop terminated and bed fumigated with Mp, the estimated mean pathogen density of the outer lines was significantly higher than that of the inner lines (; Z = 2.0, df = 1, P = .041).
Table 3. Estimated mean pathogen densities for trials 1 and 2. Lines I/IV denote the outer lines and lines II/III denote the inner lines of drip irrigation tape. CT = crop termination only; BF = bed fumigation only; CT + BF + crop termination and bed fumigation. Mp = metam potassium; Ms = metam sodium; Pic = chloropicrin. ± represent standard error of the mean. P-value resulting from a Tukey multiple comparison test with α = 0.05.
For plots that were subject to treatment with only Ms in trial 1, no significant differences in estimated mean pathogen density were found between the inner and outer lines in plots only crop terminated with Ms (Z = −0.83, df = 1, P = .41), in plots only bed fumigated with Ms (Z = −1.7, df = 1, P = .10), and in plots both crop terminated and bed fumigated with Ms (Z = 0.85, df = 1, P = .40). For plots that were subject to treatment with Ms in trial 2, a significantly higher estimated mean pathogen density was found in the outer lines for plots only crop terminated with Ms (; Z = 2.1, df = 1, P = .038). No significant differences in estimated mean pathogen density between the inner and outer lines in plots only bed fumigated with Ms (Z = 0.56, df = 1, P = .57) and in plots both crop terminated and bed fumigated with Ms were found (Z = 1.9, df = 1, P = .055).
Finally, for plots that were subject to sequential crop termination with Mp and bed fumigation with Pic, similar results apply. In both trials, no significant differences were found in estimated mean pathogen density between the inner and outer lines in plots only crop terminated with Mp (trial 1: Z = −0.17, df = 1, P = .87; trial 2: Z = 1.4, df = 1, P = .14), in plots only bed fumigated with Pic (trial 1: Z = −1.1, df = 1, P = .26; trial 2: Z = 1.4, df = 1, P = .15), and in plots sequentially crop terminated with Mp and bed fumigated with Pic (trial 1: Z = 1.0, df = 1, P = .31; trial 2: Z = 1.3, df = 1, P = .19).
Khatri et al. (Citation2020) established that metam products such as Mp when applied via drip irrigation in a crop termination application provided adequate crop kill, suppression of M. phaseolina at bed center, and control of various weeds such as purple nutsedge. Furthermore, Chamorro et al. (Citation2016) demonstrated that the application of Mp had similar effects to that of Mb in reducing pathogen survivability, yet it was not effective against M. phaseolina inoculum at the edge of the bed. Candole et al. (Citation2007) showed that in sandy soil beds, highest metam product fumigant concentrations were found 20 cm below the centrally located drip tape emitter and was lowest at 30 cm away from the emitter, exhibiting the lack of dispersion of these products. Our findings are somewhat similar to this study, for in trial 2 plots sequentially crop terminated and bed fumigated with Mp and plots only crop terminated with Ms had statistically higher inoculum density away from the centrally placed drip tape even with our clay loam soil type. Yet, these findings were not consistent between trials. Methyl isothiocyanate generators like Mp and Ms have been shown to provide adequate distribution in sandy loam soil at medium water application amounts (Nelson et al., Citation2013). Our trial site had soil characterized as clay loam, and MITC absorption has shown to be lower in soils with higher clay characteristics (Gerstl et al., Citation1977) and clay soils are more difficult to fumigate compared to sandy loam soils due to sorptive properties that differ at various depths within the soil profile (Munnecke and Van Gundy, Citation1979).
In trial 1, the inner lines in plots bed fumigated with Mp had a significantly higher inoculum level compared to the outer lines. Although this was not consistent with trial 2, plots that were both crop terminated and bed fumigated with Mp in trial 2 had significantly higher inoculum density in the outer lines. Our study utilized three lines of drip tape per bed providing greater fumigant dispersal compared to two lines of drip tape. After the crop termination application, experimental beds were hand-tilled, and plastic and drip tape were laid by hand prior to bed fumigation treatments; this could explain the higher inoculum densities in the outer plant rows. While we were cognizant to not move soil from the furrows to the beds, inadvertent placement of plant debris in the middle of the beds may have led to higher inoculum levels in the inner plant rows. Although a rototiller was utilized in trial 2, we observed lower V. dahliae inoculum density in the middle lines compared to trial 1. This may indicate that employing the rototiller resulted in more adequate incorporation and dispersal of plant debris compared to hand-tillage. With this, significant differences found in V. dahliae inoculum density in the lines between trials were not consistent.
In this experiment, the integration of other disease management practices like crop rotation was not implemented. The integration of crop rotation may be able to further reduce inoculum levels (Chellemi et al., Citation1997) before strawberry is planted again. Crop rotation with broccoli and the applications of crab/feather meal has shown to bring microsclerotia densities down to 1 CFU/g (Chellemi et al., Citation1997). Thus, fumigation practices alone may not be suitable for adequate control of V. dahliae.
Conclusion
Proper management of Verticillium wilt can possibly be achieved with the reduction of V. dahliae populations in strawberry cropping systems. This study focused not only on the adequacy of inoculum reduction in the soil but reducing the buildup of V. dahliae survival structures in plant tissue prior to tillage. Further, the applications of an end-of-season fumigation practice like crop termination, together with a pre-plant fumigation practice like bed fumigation, could aid growers in decreasing disease occurrence in cropping systems with high pathogen pressure. Our findings show that among plots treated with sequential crop termination and bed fumigation, those crop terminated with Mp and bed fumigated with Pic showed lower inoculum densities compared to plots sequentially treated with metam products. Drip irrigation system is subject to deterioration over time during the season that can affect the crop termination treatment efficacy due to uneven movement of the fumigants. Our study was conducted in a less than 0.01-hectare area with heterogeneously distributed natural inoculum of V. dahliae. Large scale studies should be used to further validate findings of the current study. The integration of resistant cultivars could also reduce mortality, yet further investigations are needed to examine the selection of cultivars after fumigation applications, as well as the application of these fumigation methods in soil-types (sandy loam) more commonly found within strawberry cropping systems and in applications on a larger scale. With focus on reducing inoculum already present, as well as preventing inoculum buildup due to successive strawberry crops, integrating different approaches for control of Verticillium wilt would also provide favorable conditions for plant health and yield improvements.
Supplemental Material
Download Zip (188.3 KB)Acknowledgments
The authors thank: A. Bella, K. Blauer, C. Calvin, C. Chavez-Gonzalez, A. Cruz, E. Hernandez, A. Hysell, A. Inoue, V. Longacre, R. Pappani, J. Ramirez, R. Ravarra, K. Roberson, G. Rodriguez, S. Simard, M. Steele, D. Summerfield, L. Tavoletti, S. Tobin, and Y. Wang for their technical support throughout this project.
Disclosure statement
No potential conflict of interest was reported by the author(s).
Data availability statement
The data that support the findings of this study are available from the corresponding author, JTK, upon reasonable request.
Supplementary material
Supplemental data for this article can be accessed online at https://doi.org/10.1080/15538362.2023.2292132
Additional information
Funding
References
- Ajwa, H.A., T. Trout, J. Mueller, S. Wilhelm, S.D. Nelson, R. Soppe, and D. Shatley. 2002. Application of alternative fumigants through drip irrigation systems. Phytopathology 92(12):1349–1355. doi: 10.1094/PHYTO.2002.92.12.1349.
- Andersen, A.A. 1958. New sampler for the collection, sizing, and enumeration of viable airborne particles. J. Bacteriol. 76(5):471–484. doi: 10.1128/jb.76.5.471-484.1958.
- Baggio, J.S., M. Chamorro, L.G. Cordova, J.W. Noling, G.E. Vallad, and N.A. Peres. 2018. Effect of formulations of allyl isothiocyanate on survival of Macrophomina phaseolina from strawberry. Plant Dis. 102(11):2212–2219. doi: 10.1094/PDIS-01-18-0172-RE.
- Baggio, J.S., R.G. Ruschel, J.W. Noling, and N.A. Peres. 2021. Physical, cultural, and chemical alternatives for integrated management of charcoal rot of strawberry. Plant Dis. 105(2):295–304. doi: 10.1094/PDIS-04-20-0917-RE.
- Bates, D., M. Mächler, B. Bolker, and S. Walker. 2015. Fitting linear mixed-effects models using lme4. J. Stat Soft. 67(1):1–48. doi: 10.18637/jss.v067.i01.
- Beckman, C.H., and E.M. Roberts. 1995. On the nature and genetic basis for resistance and tolerance to fungal wilt diseases of plants. Adv. Bot. Res. 21:35–77. doi: 10.1016/s0065-2296(08)60008-7.
- Bejarano-Alcazar, J., J.M. Melero-Vara, M.A. Blanco-Lopez, and R.M. Jimenez-Diaz. 1995. Influence of inoculum density of defoliating and nondefoliating pathotypes of Verticillium dahliae on epidemics of Verticillium wilt of cotton in Southern Spain. Phytopathology 85(12):1474–1481. doi: 10.1094/phyto-85-1474.
- Bhat, R.G., and K.V. Subbarao. 1999. Host range specificity in Verticillium dahliae. Phytopathology 89(12):1218–1225. doi: 10.1094/PHYTO.1999.89.12.1218.
- Bok, J.W., and N.P. Keller. 2012. Fast and easy method for construction of plasmidvectors using modified quick-change mutagenesis. Methods Mol. Biol. 944:163–174.
- Bolda, M., J. Murdock, and D.A. Sumner. 2021. 2021 cost of production study for conventional strawberries. UCANR, Davis, CA. 22 Dec. 2021. https://ucanr.edu/blogs/strawberries_caneberries/blogfiles/87914.pdf
- Brannen, P., and B. Cline. 2021. Southeast regional strawberry integrated pest management guide focused on plasticulture production. The University of Georgia, Athens, GA. Jan. 2020. https://smallfruits.org/files/2020/02/2020-Strawberry-IPM-Guide.pdf
- California Department of Food and Agriculture. 2021. California Agricultural Statistics Review. California Department Of Food And Agriculture, California. 1 Sep. 2022. https://www.cdfa.ca.gov/Statistics/PDFs/2022_Ag_Stats_Review.pdf
- California soil resource lab, UC Davis/UC-ANR/USDA NRCS. 2022. SoilWeb. (https://casoilresource.lawr.ucdavis.edu/gmap/)
- Candole, B.L., A.S. Csinos, and D. Wang. 2007. Concentrations in soil and efficacy of drip-applied 1,3-D chloropicrin and metam sodium in plastic-mulched sandy soil beds. Crop Prot. 26(12):1801–1809. doi: 10.1016/j.cropro.2007.03.021.
- Chamorro, M., T.E. Seijo, J.C. Noling, B. De Los Santos, and N.A. Peres. 2016. Efficacy of fumigant treatments and inoculum placement on control of Macrophomina phaseolina in strawberry beds. Crop. Protection 90:163–169. doi: 10.1016/j.cropro.2016.08.020.
- Chellemi, D.O., S.M. Olson, D.J. Mitchell, I. Secker, and R. McSorley. 1997. Adaptation of soil solarization to the integrated management of soilborne pests of tomato under humid conditions. Phytopathology 87(3):250–258. doi: 10.1094/PHYTO.1997.87.3.250.
- Duniway, J.M. 2002. Status of chemical alternatives to methyl bromide for pre-plant fumigation of soil. Phytopathology 92(12):1337–1343. doi: 10.1094/PHYTO.2002.92.12.1337.
- Gerstl, Z., U. Mingelgrin, and B. Yaron. 1977. Behavior of vapam and methylisothiocyanate in soils. Soil. Sci. Soc. Am. J. 41(3):545–548. doi: 10.2136/sssaj1977.03615995004100030024x.
- Gordon, T.R., and K.V. Subbarao. 2007. Production guidelines: Verticillium wilt of strawberry. UC-ANR. https://ucanr.edu/blogs/strawberries_caneberries/index.cfm?tagname=Verticillium%20wilt
- Guthman, J. 2019. Wilted: Pathogens, chemicals, and the fragile future of the strawberry industry. University of California Press, Oakland, CA, USA.
- Hall, T.A. 1999. BioEdit: A user-friendly biological sequence alignment editor and analysis program for windows 95/98/NT. Nucleic Acids Symp. Ser. 41(2):95–98. doi: 10.14601/phytopathol_mediterr-14998u1.29.
- Harris, D.C., and J.R. Yang. 1996. The relationship between the amount of Verticillium dahliae in soil and the incidence of strawberry wilt as a basis for disease risk prediction. Plant Pathol. 45(4):795–806. doi: 10.1046/j.1365-3059.1996.d01-96.x.
- Khatri, K., N.A. Peres, J.S. Baggio, and N.S. Boyd. 2020. Strawberry crop termination, weed control and Macrophomina phaseolina inoculum control with metam potassium at season end. Crop Prot. 135(12):105207. doi: 10.1016/j.cropro.2020.105207.
- Klose, S., H.A. Ajwa, G.T. Browne, K.V. Subbarao, F.N. Martin, S.A. Fennimore, and B.B. Westerdahl. 2007. Dose response of weed seeds, plant-parasitic nematodes, and pathogens to twelve rates of metam sodium in a California soil. Plant Dis. 92(11):1537–1546. doi: 10.1094/PDIS-92-11-1537.
- Kreutzer, W.A. 1963. Selective toxicity of chemicals to soil microorganisms. Annu. Rev. Phytopathol. 106(6):101–126. doi: 10.1146/annurev.py.01.090163.000533.
- Lopez-Escudero, F.J., and M.A. Blanco-Lopez. 2001. Effect of a single or double soil solarization to control Verticillium wilt in established olive orchards in Spain. Plant Dis. 85(5):489–496. doi: 10.1094/PDIS.2001.85.5.489.
- Martin, F., S. Fennimore, A. Putman, M. Matson, F. Melton, R. Goodhue, P. Henry, S. Vougioukas, N. Dorn, C. Greer, et al. 2019. Site-specific soil pest management in strawberry and vegetable cropping systems. Paper presented at the International Conference on Alternatives to Methyl Bromide. San Diego, CA, Sept. 2019.
- Mihail, J.D. 1992. Macrophomina, In: pp. 134–136. In: L.L. Singleton, J.D. Mihail, and C.M. Rush (eds.). Methods for research on soilborne phytopathogenic fungi. APS Press, St. Paul, MN.
- Munnecke, D.E., and S.D. Van Gundy. 1979. Movement of fumigants in soil, dosage responses, and differential effects. Annu. Rev. Phytopathol. 17(1):405–429. doi: 10.1146/annurev.py.17.090179.002201.
- Nelson, S.D., H.A. Ajwa, T. Trout, M. Stromberger, S.R. Yates, and S. Sharma. 2013. Water and methyl isothiocyanate distribution in soil after drip fumigation. J. Environ. Qual. 42(5):1555–1564. doi: 10.2134/jeq2013.03.0072.
- Njoroge, S.M., Z. Kabir, F.M. Martin, S.T. Koike, and K.V. Subbarao. 2009. Comparison of crop rotation for Verticillium wilt management and effect of pythium species in conventional and organic strawberry production. Plant Dis. 93(5):519–527. doi: 10.1094/PDIS-93-5-0519.
- Noling, J.W. 2006. The causes of pest control inconsistency with soil fumigation inFlorida strawberry. Pages 461-466 in: Proc. International Conference onAlternatives to Methyl Bromide. Orlando, FL. Nov, 2006.
- Palhano, M.G., J.K. Norsworthy, and T. Barber. 2018. Evaluation of chemical termination options for cover crops. Weed Technol 32(3):1–9. doi: 10.1017/wet.2017.113.
- Porter, I., S. Mattner, R. Mann, and R. Gounder. 2004. Strawberry nurseries: Summaries of alternatives to methyl bromide fumigation and trials in different geographic regions. Paper presented at the International Conference on Alternatives to Methyl Bromide. Lisbon, Portugal, Sept. 2004.
- Searle, S.R., F.M. Speed, and G.A. Milliken. 2012. Population marginal means in the linear model: An alternative to least square means. Am. Stat. 4(34):216–221. doi: 10.1080/00031305.1980.10483031.
- Sorensen, L.H., A.T. Scheider, and J.R. Davis. 1991. Influence of sodium polygalacturonate sources and improved recovery of Verticillium spp. From soil. Phytopathology 81(10):1347.
- Street, P.F.S., and R.M. Cooper. 1984. Quantitative measurement of vascular flow in petioles of healthy and Verticillium-infected tomato. Plant Pathologyquery 33(4):483–492. doi: 10.1111/j.1365-3059.1984.tb02872.x.
- Subbarao, K.V., Z. Kabir, F.N. Martin, and S.T. Koike. 2007. Management of soilborne diseases in strawberry using vegetable rotations. Plant Dis. 91(8):964–972. doi: 10.1094/PDIS-91-8-0964.
- Tomlin, C. 1994. The pesticide manual, In: pp. 335–339. In: C. Tomlin (ed.). The pesticide manual-World Compendium. 10th ed. British Crop Protection Council, Surrey, United Kingdom.
- White, T.J., T. Bruns, S. Lee, and J.W. Taylor. 1990. Amplification and direct sequencing of fungal ribosomal RNA genes for phylogenetics, In: pp. 315–322. In: M.A. Innis, D.H. Gelfand, J.J. Sninsky, and T.J. White (eds.). PCR protocols: A guide to methods and applications. Academic Press Inc, New York.
- Wilhelm, S. 1958. Longevity of the Verticillium wilt fungus in the laboratory and the field. Phytopathology 45(3):180–181.
- Wilhelm, S. 1966. Chemical treatments and inoculum potential of soil. Annu. Rev. Phytopathology 4(1):53–78. doi: 10.1146/annurev.py.04.090166.000413.
- Wilhelm, S. 1998. Verticillium wilt, In: pp. 1–52. In: J.L. Mass (ed.). Compendium of Strawberry Diseases. American Phytopathological Society, St Paul, MN.
- Wilhelm, S., and E. Koch. 1956. Verticillium wilt controlled: Chloropicrin achieves effective control of Verticillium wilt in strawberry plantings if properly applied as soil fumigant. Calif. Agr. 10(6):3–14.
- Wilhelm, S., and A.O. Paulus. 1980. How soil fumigation benefits the California strawberry industry. Plant Dis. 64(3):264–270. doi: 10.1094/pd-64-264.
- Wilhelm, S., R. Storkan, and J. Sagen. 1961. Verticillium wilt of strawberry controlled by fumigation of soil with chloropicrin and chloropicrin-methyl-bromide mixtures. Phytopathology 51(11):744–748. doi: 10.1016/0304-1131(74)90020-4.
- Xiao, C.L., J.J. Hao, and K.V. Subbarao. 1997. Spatial patterns of microsclerotia of Verticillium dahliae in soil and Verticillium wilt of cauliflower. Phytopathology 87(3):325–331. doi: 10.1094/phyto.1997.87.3.325.
- Zavatta, M., J. Muramoto, E. Milazzo, S. Koike, K. Klonsky, R. Goodhue, and C. Shennan. 2021. Integrating broccoli rotation, mustard meal, and anaerobic soil disinfestation to manage Verticillium wilt in strawberry. Crop Prot. 146:105659. doi: 10.1016/j.cropro.2021.105659.