ABSTRACT
Mitochondrial dysfunction plays a crucial role in the macroautophagy/autophagy cascade. In a recently published study Sun et al. described the induction of autophagy by the membranophilic triphenylphosphonium (TPP)-based cation 10-(6′-ubiquinonyl) decyltriphenylphosphonium (MitoQ) in HepG2 cells (Sun C, et al. “MitoQ regulates autophagy by inducing a pseudo-mitochondrial membrane potential [PMMP]”, Autophagy 2017, 13:730-738.). Sun et al. suggested that MitoQ adsorbed to the inner mitochondrial membrane with its cationic moiety remaining in the intermembrane space, adding a large number of positive charges and establishing a “pseudo-mitochondrial membrane potential,” which blocked the ATP synthase. Here we argue that the suggested mechanism for generation of the “pseudo-mitochondrial membrane potential” is physically implausible and contradicts earlier findings on the electrophoretic displacements of membranophilic cations within and through phospholipid membranes. We provide evidence that TPP-cations dissipated the mitochondrial membrane potential in HepG2 cells and that the induction of autophagy in carcinoma cells by TPP-cations correlated with the uncoupling of oxidative phosphorylation. The mild uncoupling of oxidative phosphorylation by various mitochondria-targeted penetrating cations may contribute to their reported therapeutic effects via inducing both autophagy and mitochondria-selective mitophagy.
Mitochondria could be considered both as the “victims” of autophagy and as important regulators of signaling pathways that eventually cause autophagy. Hence, mitochondria contribute to prosurvival functions of autophagy such as hormesis, an adaptive response to toxins and other stressors. Furthermore, activation of autophagy can lead to the removal of damaged organelles (including mitochondria) and protein aggregates (such as polyglutamine proteins that cause Huntington disease). In addition, autophagy can provide substrates for fasting cells, thereby interfering with apoptosis and protecting against tissue damage. One of the well-known examples of hormesis is ischemic preconditioning (IPC) where short episodes of ischemia protect various organs against a later ischemic insult. The IPC-activated autophagy in heart, brain, kidney, and liver is due to the decrease in mitochondrial ATP production, whereas the inhibition of autophagy ameliorates the protection of these organs.Citation1 A decrease in the cellular ATP:AMP ratio may induce autophagy via the AMP-activated protein kinase (AMPK) acting either as the negative regulator of MTOR (mechanistic target of rapamycin kinase) or directly by phosphorylating the kinase ULK1 that is involved in the initiation of autophagy.Citation2
Recently, Sun et al. have reportedCitation3 that autophagy in HepG2 cells could be stimulated by 10-(6′-ubiquinonyl) decyltriphenylphosphonium (MitoQ), a mitochondria-targeted antioxidant.Citation4 Sun et al. showed that the addition of 1 μM MitoQ leads to the decrease in ATP:AMP ratio, stimulates AMPK and inhibits MTOR. To explain the diminishing of the ATP:AMP ratio, the authors suggested that the ATP synthesis was suppressed by “pseudo-mitochondrial membrane potential”. Sun et al. suggested that “MitoQ is adsorbed to the inner mitochondrial membrane; however, its cationic moiety remains in the intermembrane space, resulting in the addition of a large number of positive charges…. Furthermore, the positive charges in MitoQ replaced protons in the establishment of the electrochemical gradient across the inner membrane…. This distinct mitochondrial membrane potential (MMP) … was designated “pseudo-MMP” (PMMP)” (quoted from ref.Citation3).
We think that the suggested accumulation of MitoQ molecules in the outer leaflet of the inner mitochondrial membrane with the cationic moieties of triphenylphosphonium (TPP+) facing the intermembrane space is physically implausible. The electric field of >200,000 V/cm over mitochondrial membranes (negative inside) would push the TPP+ cations towards the inner leaflet and, ultimately, inside the mitochondrial matrix. Application of potential to voltage-clamped phospholipid bilayers leads to the translocation of membranophilic cations from the positively charged monolayer to the negatively charged monolayer in a voltage-dependent manner with characteristic times in the range from 10−1 sec (at temperatures above the phase transition of lipids)Citation5 to 10–100 sec (at temperatures below the phase transition).Citation6
The transmembrane electrophoresis of TPP+ and other “penetrating ions,” as driven by the transmembrane difference in electric potential (membrane potential, Δψ), was demonstrated by one of us (VPS) in the end of 1960s.Citation7,Citation8 Later, with phospholipid bilayers, it was shown that the transmembrane concentration gradients of MitoQ, its plastoquinone-containing analog 10-(6′-plastoquinonyl) decyltriphenylphosphonium (SkQ1), and dodecyltriphenylphosphonium (C12TPP), the analog of the cationic residue of MitoQ and SkQ1, causes the appearance of a Nerstian electric potential (approx. 60 mV per 10-fold gradient of a monovalent cation).Citation9 It was also shown that membranophilic cations can diminish membrane potential not only by their own redistribution, but also by facilitating the transmembrane transport of free fatty acid (FFA) anions.Citation10 The transport of FFA anions across the inner mitochondrial membrane is the rate-limiting step of the FFA-mediated uncoupling proton flux (see the scheme in A). A FFA-dependent induction of the transmembrane proton flow by membranophilic cations was directly demonstrated in FFA-containing phospholipid bilayers, liposomes, isolated mitochondria, and yeast cells.Citation10
Figure 1. Membrane depolarization as a trigger of autophagy in mitochondria. (A) Scheme of the protonophoric action of membranophilic cations (adapted from ref.Citation10 with permission). Membranophilic cations (R-TPP) facilitate the transmembrane translocation of anionic free fatty acids (FFA) by forming complexes with them. The symbols ⊖ and ⊕ correspond to the anionic and cationic groups of FFA and R-TPP, respectively. The letter “H” within a circle indicates the protonated COOH headpiece of a fatty acid. (B) Dissipation of Δψ by MitoQ in HepG2 cells. The conditions of the experiments were exactly the same as in Sun et al.Citation3 Cells were incubated for 60 min at 37°C with 1 μM MitoQ and then for 30 min with 10 μM CCCP (Sigma-Aldrich, C2759). MitoQ was synthesized as described in ref.Citation4 To measure Δψ the cells were stained with 100 nM TMRM (Thermo Scientific, T668) for 30 min. Fluorescence was analyzed using a Beckman Coulter FC500 flow cytometer (Beckman Coulter Inc., USA). Unimodal distribution close to Gaussian was observed in all samples and the Xmean ±SD (n = 3) values are plotted. a.u., arbitrary units. (C) Dissipation of Δψ by C12TPP, SkQ1 and FCCP in RKO cells. Cells were incubated with C12TPP, SkQ1 and FCCP (Sigma-Aldrich, C2920) for 60 min at 37°C and analyzed as in B. C12TPP and SkQ1 were synthesized as described in ref.Citation9 (D) LC3 clustering in RKO cells in response to the SkQ1 addition. To follow LC3 clustering a GFP-LC3 fusion construct was expressed in RKO cells using lentivirus transfection. Cells were treated with SkQ1 for 24 h and analyzed using a fluorescence Axiovert 200 microscope (Carl Zeiss, Germany). Typical images are shown. Scale bar: 15 μm. (E) Quantification of the LC3 clustering in RKO cells in response to the addition of SkQ1 and C12TPP. Cells were treated as in D. GFP-LC3 clusters were counted in 100 cells/probe using automated counting software Kalaimoscope (Transinsight, Germany). Data are presented as Xmean ±SD; n = 3; *P < 0.05 (ANOVA) for treated vs control cells. (F) LC3 lipidation in RKO cells in response to the addition of SkQ1 and FCCP. Сells were incubated as in D and subjected to immunoblot analysis using anti-LC3 antibody (Cell Signaling Technology, 2775) and anti-GAPDH antibody (as a loading control; Santa Cruz Biotechnology, sc-25778).
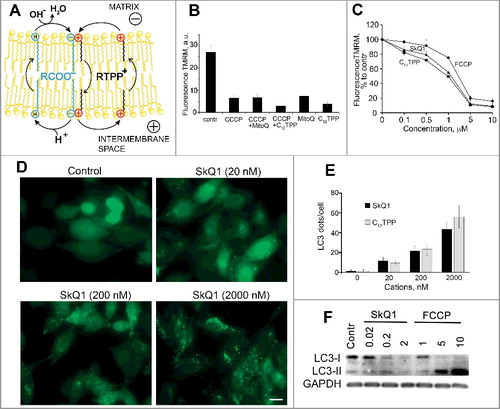
The Δψ−dependent accumulation of MitoQ (and the other TPP-containing compounds) in the mitochondrial matrix was shown in many works from different labs, as reviewed elsewhere.Citation4,Citation9,Citation11 Therefore, the addition of membranophilic cations should lead to the diminishing of membrane potential, but not to its increase.
To measure the “pseudo-mitochondrial membrane potential” Sun et al.Citation3 have used the potential-sensitive cyanine dye 5,5',6,6'-tetrachloro-1,1',3,3'-tetraethylbenzimidazolylcarbocyanine iodide (JC-1). This green fluorescent cationic dye accumulates in mitochondria due to the membrane potential and forms J-aggregates with red fluorescence. In the study of Sun et al.,Citation3 MitoQ did not affect the red fluorescence of JC-1, which speaks against the ability of MitoQ to generate an additional “pseudo-mitochondrial membrane potential.” At the same time, Sun et al.Citation3 showed that the green shift of JC-1 fluorescence, as caused by protonophore carbonyl cyanide m-chlorophenylhydrazone (CCCP), was smaller in the presence of MitoQ than in its absence. The authors attributed this difference to the contribution of “pseudo-mitochondrial potential” induced by MitoQ in the presence of CCCP. This set of intrinsically ambiguous observations, however, can be explained in several other ways. JC-1 was shown to form large aggregates that do not dissociate after depolarization of the membrane.Citation12 This property of JC-1 leads to some well-known artifacts such as mosaic heterogeneity of Δψ along a single mitochondrion.Citation13 The earlier attempt to analyze the effects of MitoQ on Δψ by using JC-1 resulted in observations of either depolarization or hyperpolarization of mitochondria depending on the cell line.Citation14 In addition, MitoQ and JC-1 can compete for ABCB1/Pgp170, a P-glycoprotein multidrug efflux pump. TPP+-containing cations are the substrates of ABCB1 and inhibit the efflux of other cationic substrates.Citation15,Citation16 In addition, JC-1 is a substrate of ABCB1 as well,Citation17 so that MitoQ, by blocking ABCB1, could stimulate the accumulation of JC-1 in the cells with active multidrug efflux pumps (such as HepG2) and promote the formation of JC-1 aggregates insensitive to CCCP. All these effects could additionally interfere with the shown ability of CCCP to induce proton release from lysosomes and acidify the cytoplasm.Citation18 The named factors could account for the intrinsic discrepancies in the work of Sun et al.Citation3
To make a long story short, we have repeated the experiment shown in Figure 3A of Sun et al.Citation3 To avoid pitfalls of the JC-1-based assay, we used tetramethylrhodamine methyl ester (TMRM) as a fluorescent cation for measuring mitochondrial membrane potential.Citation19 As shown in B, MitoQ, C12TPP, and CCCP dissipated Δψ in HepG2 cells; no particular interference between MitoQ and CCCP was observed.
That said, we agree with Sun et al.Citation3 that MitoQ (and other TPP+-containing compounds) can induce autophagy. However, alternatively to Sun et al.,Citation3 we attribute the effects to the partial uncoupling of oxidative phosphorylation. In support of our suggestion, we demonstrate that mitochondrial depolarization in response to the addition of the membranophilic cations C12TPP and SkQ1, as well as the uncoupler carbonyl cyanide p-trifluoromethoxyphenylhydrazone (FCCP) correlated with the activation of autophagy in RKO carcinoma cells (C-F). The membrane potential of mitochondria was measured by TMRM (C). The autophagy was followed by the accumulation of MAP1LC3B/LC3B (microtubule associated protein 1 light chain 3 beta) clusters (D,E) and LC3 lipidation (LC3-II formation) (F). The data in B-F suggest that the autophagy in the experiments of Sun et al.Citation3 could be also induced by depolarization of mitochondria in response to the addition of MitoQ.
In our experiments, membranophilic cations C12TPP and SkQ1 appeared more effective in dissipating Δψ than the dedicated protonophoric uncoupler FCCP (B,C). These data are in agreement with earlier observations where C12TPP, already at nanomolar concentrations, induces a measurable decrease in mitochondrial membrane potential in endothelial cells, being slightly more effective than SkQ1.Citation20 The high efficiency of C12TPP and SkQ1 in dissipating the membrane potential could be caused by their selective accumulation in mitochondria, which is driven by the cumulative impact from the membrane potential at the plasma membrane and at the inner mitochondrial membrane.Citation7
In the experiments presented in F, the high doses of FCCP induced excessive accumulation of LC3-II (as compared to the effects of C12TPP and SkQ1), which was presumably due to the inhibition of autophagic flux. Earlier it was shown that high doses of protonophoric uncouplers induce proton release from lysosomes and appear to hinder the lysosomal degradation of autophagosomal cargo in both yeast and mammalian cells.Citation18 In contrast, membranophilic cations are unlikely to interfere with the autophagic flux. These cations selectively accumulate in mitochondria; their concentrations in the other cellular membranes are much lower, so that the artifacts inherent to traditional uncouplers are minimized.
Membranophilic cations can be considered as self-limiting or “mild” uncouplers because the increase in their concentration should result in lowering the mitochondrial membrane potential that drives their accumulation in mitochondria. The mild uncoupling is largely considered as a promising therapeutic approach because it minimizes the production of reactive oxygen species without notably decreasing the ATP yield.Citation21 Induction of autophagy (and mitophagy) in response to the decrease in membrane potential could additionally contribute to the therapeutic effects of the membranophilic cation, which are observed with the models of kidney and brain ischemia/reperfusion injuriesCitation22,Citation23 as well as with the model of systemic inflammatory response syndrome.Citation20,Citation24
Disclosure of Potential Conflicts of Interest
The authors report no conflicts of interest.
Additional information
Funding
References
- Martins I, Galluzzi L, Kroemer G. Hormesis, cell death and aging. Aging (Albany NY). 2011;3(9):821–828. doi:10.18632/aging.100380.
- Egan DF, Shackelford DB, Mihaylova MM, Gelino S, Kohnz RA, Mair W, Vasquez DS, Joshi A, Gwinn DM, Taylor R, et al. Phosphorylation of ULK1 (hATG1) by AMP-activated protein kinase connects energy sensing to mitophagy. Science. 2011;331(6016):456–461. doi:10.1126/science.1196371.
- Sun C, Liu X, Di C, Wang Z, Mi X, Liu Y, Zhao Q, Mao A, Chen W, Gan L, et al. MitoQ regulates autophagy by inducing a pseudo-mitochondrial membrane potential. Autophagy. 2017;13(4):730–738. doi:10.1080/15548627.2017.1280219.
- Kelso GF, Porteous CM, Coulter CV, Hughes G, Porteous WK, Ledgerwood EC, Smith RA, Murphy MP. Selective targeting of a redox-active ubiquinone to mitochondria within cells: antioxidant and antiapoptotic properties. J Biol Chem. 2001;276(7):4588–4596. doi:10.1074/jbc.M009093200.
- Melikyan GB, Deriy BN, Ok DC, Cohen FS. Voltage-dependent translocation of R18 and DiI across lipid bilayers leads to fluorescence changes. Biophys J. 1996;71(5):2680–2691. doi:10.1016/S0006-3495(96)79459-6.
- Rokitskaya TI, Klishin SS, Severina II, Skulachev VP, Antonenko YN. Kinetic analysis of permeation of mitochondria-targeted antioxidants across bilayer lipid membranes. J Membr Biol. 2008;224(1–3):9–19. doi:10.1007/s00232-008-9124-6.
- Liberman EA, Skulachev VP. Conversion of biomembrane-produced energy into electric form .4. General Discussion. Biochim Biophys Acta. 1970;216(1):30–42. doi:10.1016/0005-2728(70)90156-8. PMID:4250572
- Liberman EA, Topaly VP, Tsofina LM, Jasaitis AA, Skulachev VP. Mechanism of coupling of oxidative phosphorylation and the membrane potential of mitochondria. Nature. 1969;222(5198):1076–1078. doi:10.1038/2221076a0.
- Skulachev VP, Anisimov VN, Antonenko YN, Bakeeva LE, Chernyak BV, Erichev VP, Filenko OF, Kalinina NI, Kapelko VI, Kolosova NG, et al. An attempt to prevent senescence: a mitochondrial approach. Biochim Biophys Acta. 2009;1787(5):437–461. doi:10.1016/j.bbabio.2008.12.008.
- Severin FF, Severina II, Antonenko YN, Rokitskaya TI, Cherepanov DA, Mokhova EN, Vyssokikh MY, Pustovidko AV, Markova OV, Yaguzhinsky LS, et al. Penetrating cation/fatty acid anion pair as a mitochondria-targeted protonophore. P Natl Acad Sci USA. 2010;107(2):663–668. doi:10.1073/pnas.0910216107.
- Kagan VE, Wipf P, Stoyanovsky D, Greenberger JS, Borisenko G, Belikova NA, Yanamala N, Samhan Arias AK, Tungekar MA, Jiang J, et al. Mitochondrial targeting of electron scavenging antioxidants: Regulation of selective oxidation vs random chain reactions. Adv Drug Deliv Rev. 2009;61(14):1375–1385. doi:10.1016/j.addr.2009.06.008.
- Kawasaki M, Inokuma H. Contribution of electrostatic cohesive energy in two-dimensional J-aggregation of cyanine dye. J Phys Chem B. 1999;103(8):1233–1241. doi:10.1021/jp983736f.
- Smiley ST, Reers M, Mottolahartshorn C, Lin M, Chen A, Smith TW, Steele GD, Chen LB. Intracellular heterogeneity in mitochondrial-membrane potentials revealed by a J-Aggregate-forming lipophilic Cation Jc-1. P Natl Acad Sci USA. 1991;88(9):3671–3675. doi:10.1073/pnas.88.9.3671.
- Ng MRAV, Antonelli PJ, Joseph J, Dirain CO. Assessment of mitochondrial membrane potential in HEI-OC1 and LLC-PK1 cells treated with gentamicin and mitoquinone. Otolaryng Head Neck. 2015;152(4):729–733. doi:10.1177/0194599814564934.
- Fetisova EK, Avetisyan AV, Izyumov DS, Korotetskaya MV, Chernyak BV, Skulachev VP. Mitochondria-targeted antioxidant SkQR1 selectively protects MDR (Pgp 170)-negative cells against oxidative stress. Febs Lett. 2010;584(3):562–566. doi:10.1016/j.febslet.2009.12.002.
- Knorre DA, Markova OV, Smirnova EA, Karavaeva IE, Sokolov SS, Severin FF. Dodecyltriphenylphosphonium inhibits multiple drug resistance in the yeast Saccharomyces cerevisiae. Biochem Bioph Res Co. 2014;450(4):1481–1484. doi:10.1016/j.bbrc.2014.07.017.
- Kuhnel JM, Perrot JY, Faussat AM, Marie JP, Schwaller MA. Functional assay of multidrug resistant cells using JC-1, a carbocyanine fluorescent probe. Leukemia. 1997;11(7):1147–1155. doi:10.1038/sj.leu.2400698.
- Padman BS, Bach M, Lucarelli G, Prescott M, Ramm G. The protonophore CCCP interferes with lysosomal degradation of autophagic cargo in yeast and mammalian cells. Autophagy. 2013;9(11):1862–1875. doi:10.4161/auto.26557.
- Scaduto RC, Grotyohann LW. Measurement of mitochondrial membrane potential using fluorescent rhodamine derivatives. Biophysical J. 1999;76(1):469–477. doi:10.1016/S0006-3495(99)77214-0.
- Romaschenko VP, Zinovkin RA, Galkin II, Zakharova VV, Panteleeva AA, Tokarchuk AV, Lyamzaev KG, Pletjushkina OY, Chernyak BV, Popova EN. Low concentrations of uncouplers of oxidative phosphorylation prevent inflammatory activation of endothelial cells by tumor necrosis factor. Biochemistry-Moscow. 2015;80(5):610–619. doi:10.1134/S0006297915050144.
- Korshunov SS, Skulachev VP, Starkov AA. High protonic potential actuates a mechanism of production of reactive oxygen species in mitochondria. Febs Lett. 1997;416(1):15–18. doi:10.1016/S0014-5793(97)01159-9.
- Antonenko YN, Denisov SS, Silachev DN, Khailova LS, Jankauskas SS, Rokitskaya TI, Danilina TI, Kotova EA, Korshunova GA, Plotnikov EY, et al. A leng-linker conjugate of fluorescein and triphenylphosphonium as mitochondria-targeted uncoupler and fluorescent neuroand nephroprotector. Biochim Biophys Acta. 2016;1860(11):2463–2473. doi:10.1016/j.bbagen.2016.07.014.
- Plotnikov EY, Silachev DN, Jankauskas SS, Rokitskaya TI, Chupyrkina AA, Pevzner IB, Zorova LD, Isaev NK, Antonenko YN, Skulachev VP, et al. Mild uncoupling of respiration and phosphorylation as a mechanism providing nephro- and neuroprotective effects of penetrating cations of the SkQ family. Biochemistry-Moscow. 2012;77(9):1029–1037. doi:10.1134/S0006297912090106.
- Zakharova VV, Pletjushkina OY, Galkin II, Zinovkin RA, Chernyak BV, Krysko DV, Bachert C, Krysko O, Skulachev VP, Popova EN. Low concentration of uncouplers of oxidative.phosphorylation decreases the TNF-induced endothelial permeability and lethality in mice. Biochim Biophys Acta. 2017;1863(4):968–977. doi:10.1016/j.bbadis.2017.01.024.