ABSTRACT
A forward chemical genetic approach led to identification of autogramins as novel autophagy inhibitors. Autogramins selectively target the cholesterol transfer protein GRAMD1A (GRAM domain containing 1A). Autogramins compete with cholesterol binding to the StART domain of GRAMD1A, thereby inhibiting its cholesterol transfer activity. GRAMD1A associates with phosphatidylinositol monophosphate via its GRAM domain. GRAMD1A accumulates at autophagosome initiation sites upon starvation. This protein is involved in cholesterol distribution in response to starvation and is required for autophagosome biogenesis. Therefore, we identify a novel function of GRAMD1A and a new role of cholesterol in macroautophagy/autophagy.
The biogenesis of the autophagosome requires orchestrated association of protein complexes and lipids to build up the double-membrane structure. Certain phosphatidylinositol phosphates (PtdInsPs) and phosphatidic acid play an essential role in autophagosome formation and fusion with lysosomes. However, the role of lipids and lipid-binding/transfer proteins in autophagosome biogenesis remains poorly understood. Particularly, the role of cholesterol, a key neutral lipid, in autophagosome formation is largely unclear.
Chemical genetic approaches are robust strategies to dissect the complexity of biological systems. To identify new tool compounds modulating new mechanisms involved in autophagy, we employed a forward chemical genetic approach involving a high-content phenotypic screening for small-molecular modulators of autophagy and subsequent target identification of the compounds [Citation1]. The screen led to identification of novel chemotypes for autophagy modulation, including oxautin/autoquin that inhibits autophagosome-lysosome fusion, the specific PIK3C3/VPS34 inhibitor autophinib, and aumitin and authipyrin that target mitochondrial complex I. A class of aminothazoles was identified with submicromolar IC50 values. A positive probe and a negative control probe were employed in affinity-based proteomic experiments to identify targets of the compound. The only protein that was selectively enriched in a statistically significant manner was GRAMD1A (GRAM domain containing 1A). Therefore, we termed these compounds autogramins. Target engagement for autogramins was confirmed by a set of independent methods in vitro and in cells.
The members of the GRAMD1 protein family (GRAMD1A, 1B and 1C) contain a GRAM domain, a StAR-related lipid transfer (StART) domain and a transmembrane helix (). We and other labs have shown that the StART domains of GRAMD1 proteins transfer cholesterol between membranes. Autogramins specifically bind to the StART domain of GRAMD1A and also specifically inhibit the cholesterol-transfer activity of GRAMD1A but not GRAMD1B and GRAMD1C. The docked 25-hydroxycholesterol in the GRAMD1C structure adapts a binding mode similar to the sterols in the structures of yeast homologs Ysp2/Lam2 and Lam4, suggesting an evolutionarily conserved sterol-binding mode. Hydrogen deuterium exchange mass spectrometry (HDX-MS) shows that 25-hydroxycholesterol and autogramin share a similar binding site at the GRAMD1A StART domain. A mutational analysis of the ligand-binding site of the GRAMD1A StART domain confirms the conserved sterol-binding mode and suggests that the residues of the Ω1 and Ω2 loops at the entrance of the ligand-binding site could be involved in defining the selectivity of autogramins for GRAMD1A over 1B and 1C.
Figure 1. Schematic diagram of the function of GRAMD1A and cholesterol in autophagosome formation. Upon autophagy induction, GRAMD1A accumulates at the autophagosome initiation sites at the ER, potentially due to the enrichment of PtdIns3P in these sites. GRAMD1A appears to be directly engaged in autophagosome biogenesis through regulation of cholesterol homeostasis at phagophores/autophagosomes. The cholesterol content of autophagosomal membranes increases as the autophagosome matures, due in part to fusion with cholesterol rich lysosomes and late endosomes. Enhanced membrane cholesterol regulates the fusion of autophagosomes with late endosomes/lysosomes and the transport of amphisomes/autolysosomes to the cell center via the cholesterol-sensing protein OSBPL1A/ORP1L. TM, transmembrane domain; LE, late endosome; LY, lysosome.
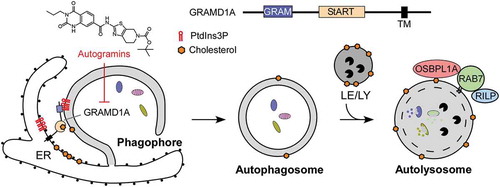
We have shown that GRAMD1A is involved in autophagosome biogenesis through regulation of cholesterol homeostasis at phagophores/autophagosomes. Autogramin-1 blocks cholesterol accumulation at autophagosomes and lysosomes in response to starvation in the presence of chloroquine, suggesting a role of GRAMD1A-mediated cholesterol transfer in autophagosome formation. Autogramin-1 inhibits formation of ATG5-labeled phagophores and LC3-labeled autophagosomes but not autolysosomes in a dose-dependent manner. Autogramin-1 does not reduce the number of WIPI2 structures but increases their lifetime, suggesting that GRAMD1A is engaged in phagophore development by acting downstream of PtdIns3P synthesis in the autophagosome formation pathway. The function of GRAMD1A was further confirmed by knockdown using the RNAi approach. After 24 h GRAMD1A knockdown leads to a robust inhibition of LC3 puncta formation, which is almost completely reversed after 72 h despite high levels of knockdown. These results indicates that adaptation and/or redundancy may play a role in genetic perturbation and stresses the importance of chemical perturbation for evaluating functions of lipid transfer proteins.
GRAMD1 proteins have been reported to localize at plasma membrane (PM)-endoplasmic reticulum (ER) contact sites in response to cholesterol and facilitate transport of cholesterol from the PM to the ER. The GRAM domain is required for the redistribution of GRAMD1B from the ER to the ER-PM contact sites in response to cholesterol. We showed that the GRAM domain of GRAMD1A specifically binds to PtdIns3P, PtdIns4P and PtdIns5P. Upon starvation, GRAMD1A enrichment occurs simultaneously with the recruitment of WIPI1, a marker of early autophagic structures, which binds to PtdIns3P. GRAMD1A accumulates at the autophagosome initiation sites at the ER, potentially because of enrichment of PtdIns3P at these sites. The GRAMD1A foci persist after the loss of WIPI1 and become the sites of LC3 foci formation. LC3-structures increase in size and remain associated with GRAMD1A foci at the ER before moving toward the perinuclear region of the cell; an event that is regulated by the cholesterol sensor OSBPL1A/ORP1L. The cholesterol content of autophagosomal membranes increases as the autophagosome matures. Elevated membrane cholesterol regulates late autophagosome transport and maturation mediated by OSBPL1A. OSBPL1A forms a tripartite complex with RAB7 and its effector RILP. OSBPL1A controls the recruitment of the dynein-dynactin motor to RILP on amphisomes/autolysosomes and the association of PLEKHM1 and the HOPS complex with RAB7-RILP on late endosomes/lysosomes via its liganding by cholesterol and binding to the ER protein VAPA at membrane contact sites under low cholesterol conditions. Our results suggest that cholesterol may play another role in regulating early events in autophagosome initiation or phagophore expansion ().
The answers to several questions remain elusive. What is the role of cholesterol and its transfer in autophagosome biogenesis? Are there unidentified cholesterol-sensing/transfer proteins involved? Would cholesterol-containing microdomains (e.g., lipid rafts) be important for autophagosome formation? How is the activity of cholesterol in early events of autophagosome formation coordinated with its function in late autophagosome transport and fusion with late endosomes/lyososomes? Future work is required to examine the dynamics of cholesterol during autophagosome formation and how it regulates the progression of autophagosome biogenesis and maturation. Autogramins and other small-molecule modulators for autophagy or cholesterol could be valuable tools for further elucidation of the autophagy mechanism.
Acknowledgments
This work was supported by Max Planck Society to H.W., and by DFG grant (SPP 1623), ERC (ChemBioAP), Vetenskapsrådet (Nr. 2018-04585), and The Knut and Alice Wallenberg Foundation to Y-W.W. We thank Daniel J. Klionsky for critical reading of this manuscript.
Disclosure statement
No potential conflict of interest was reported by the authors.
Additional information
Funding
Reference
- Laraia L, Friese A, Corkery DP, et al. The cholesterol transfer protein GRAMD1A regulates autophagosome biogenesis. Nat Chem Biol. 2019;15(7):710–720.