ABSTRACT
Deficiency in decidualization has been widely regarded as an important cause of spontaneous abortion. Generalized decidualization also includes massive infiltration and enrichment of NK cells. However, the underlying mechanism of decidual NK (dNK) cell residence remains largely unknown. Here, we observe that the increased macroautophagy/autophagy of decidual stromal cells (DSCs) during decidualization, facilitates the adhesion and retention of dNK cells during normal pregnancy. Mechanistically, this process is mediated through activation of the MITF-TNFRSF14/HVEM signaling, and further upregulation of multiple adhesion adhesions (e.g. Selectins and ICAMs) in a MMP9-dependent manner. Patients with unexplained spontaneous abortion display insufficient DSC autophagy and dNK cell residence. In addition, poor vascular remodeling of placenta, low implantation number and high ratio of embryo loss are observed in NK cell depletion mice. In therapeutic studies, low doses of rapamycin, a known autophagy inducer that significantly promotes endometrium autophagy and NK cell residence, and improves embryo absorption in spontaneous abortion mice models, which should be dependent on the activation of MITF-TNFRSF14/HVEM-MMP9-adhension molecules axis. This observation reveals novel molecular mechanisms underlying DSCs autophagy-driven dNK cell residence, and provides a potential therapeutic strategy to prevent spontaneous abortion.
Abbreviations: ACTA2/αSMA: actin alpha 2, smooth muscle; ATG: autophagy-related; ATG5over ESC: ATG5-overexpressed ESCs; BTLA: B and T lymphocyte associated; CDH1: cadherin 1; CDH5: cadherin 5; CXCL12: C-X-C motif chemokine ligand 12; dNK: decidual NK; DIC: decidual immune cell; DSC: decidual stromal cell; EOMES: eomesodermin; ESC: endometrial stromal cell; FCGR3A/CD16: Fc fragment of IgG receptor IIIa; HUVEC: human umbilical vein endothelial cell; ICAM: intercellular cell adhesion molecule; ILC: innate lymphoid cell; ITGB1: integrin subunit beta 1; ITGA2: integrin subunit alpha 2; IPA: Ingenuity Pathway Analysis; KIR2DL1: killer cell immunoglobulin like receptor, two Ig domains and long cytoplasmic tail 1; KLRD1/CD94: killer cell lectin like receptor D1; KLRK1/NKG2D: killer cell lectin like receptor K1; MAP1LC3B/LC3B: microtubule associated protein 1 light chain 3 beta; 3-MA: 3-methyladenine; MITF: melanocyte inducing transcription factor; MiT-TFE: microphthalmia family of bHLH-LZ transcription factors; MMP9: matrix metalloproteinase 9; MTOR: mechanistic target of rapamycin kinase; NCAM1/CD56: neural cell adhesion molecule 1; NCR2/NKp44: natural cytotoxicity triggering receptor 2; NK: natural killer; KLRB1/NK1.1: killer cell lectin like receptor B1; NP: normal pregnancy; PBMC: peripheral blood mononuclear cell; PECAM1/CD31: platelet and endothelial cell adhesion molecule 1; pNK: peripheral blood NK; PRF1/Perforin: Perforin 1; PTPRC/CD45: protein tyrosine phosphatase receptor type C; Rapa: rapamycin; rh-TNFSF14/LIGHT: recombinant human TNFSF14/LIGHT; SA: spontaneous abortion; SELE: selectin E; SELP: selectin P; SELL: selectin L; siATG5 DSCs: ATG5-silenced DSCs; siTNFRSF14/HVEM DSCs: TNFRSF14/HVEM-silenced DSCs; TBX21/T-bet: T-box transcription factor 21; SQSTM1/p62: sequestosome 1; TNFRSF14/HVEM: TNF receptor superfamily member 14; TNFSF14/LIGHT: TNF superfamily member 14; uNK: uterine NK; UIC: uterine immune cell; USC: uterine stromal cell; VCAM1: vascular cell adhesion molecule 1; VIM: vimentin.
Introduction
Spontaneous abortion is associated with 10–15% of clinically recognized pregnancies in humans. Although studies have reported that various factors (e.g. chromosomal and anatomical uterine abnormalities, endocrine abnormalities, endometrial infections and immune factors) are involved in the etiology of spontaneous abortion [Citation1,Citation2], the underlying mechanism remains unclear and the cases of a large proportion of spontaneous abortion remain unexplained.
Decidualization denotes the differentiation and transformation of endometrial stromal cells (ESCs) into specialized decidual stromal cells (DSCs) prior to and during pregnancy [Citation3]. Loss of endometrial plasticity and aberrant decidualization are associated with early pregnancy loss [Citation3,Citation4]. Decidualization comprises a complex change in cell morphology and function. Fibroblast-like ESCs expand their cytoplasm and nucleus becoming large specialized secretory cells. Cellular homeostasis of DSCs is maintained through protein quality controls that balance synthesis and degradation. Decidualizing stromal cells show dilatation of the rough endoplasmic reticulum and Golgi systems as well as increased number of intracellular phagosomes and lysosomes [Citation5,Citation6].
Autophagy is an evolutionarily conserved process in eukaryotes to maintain intracellular protein quality controls and cellular homeostasis under environmental stress [Citation7]. The functional connection between autophagy and endometrial physiology has been described. The increased detection of an autophagosome marker, the lipidated form of MAP1LC3B/LC3B (microtubule associated protein 1 light chain 3 beta; LC3B-II), correlates with the progression of the menstrual cycle, reaching a maximum level toward the late secretory phase [Citation8]. Endometrial autophagy may be essential for embryo implantation and be associated with endometrial decidualization during early pregnancy [Citation9]. Autophagy is increased during in vitro decidualization of human ESCs [Citation10]. In vivo, inhibition of endometrial autophagy may be implicated in the decreased endometrial apoptosis during decidualization in early pregnancy [Citation11]. However, the role of autophagy in spontaneous abortion has been poorly explored.
Decidualization is accompanied with leukocyte infiltration [Citation12]. NK cells are highly proliferative after ovulation and constitute around 70% of the lymphocytes in the decidua [Citation13]. Human NK cells in the pregnant uterus have positive effects in regulating placental development and angiogenesis [Citation14]. Tissue-resident NK cells in the uterus that secrete growth-promoting factors enhancing fetal growth during critical early stages of fetal development [Citation15]. NK cell recruitment and residence in the tissues is a spatially and temporally integrated multistep process regulated by a number of chemokines and adhesive molecules [Citation16,Citation17]. The uterine NK (uNK) cells traffic in response to chemokines such as trophoblast-derived CXCL12 (C-X-C motif chemokine ligand 12) [Citation18], and proliferate and mature in response to cytokines such as IL11 (interleukin 11) and IL15 produced by resident stromal cells [Citation19]. The number of uNK cells increases in the proliferative phase and reaches a peak in the late secretory phase during menstrual cycle. Numeric variations of uNK cells seems to be more correlated to hormone-induced decidualization rather than the embryo implantation or changes in chemokine expression in decidual tissues [Citation12,Citation20,Citation21]. Currently, researches on the successful completion of decidualization and the accumulation and residence of uNK cells are still scarce.
The current study is to investigate the role and molecular mechanism of autophagy state during decidualization in dNK cell residence and homeostasis, and the dysregulated decidua autophagy-mediated unstable dNK cell niche in the pathogenesis of spontaneous abortion, and to explore the potential intervention strategies in vitro and in vivo.
Results
Decidualization is accompanied by enhanced autophagy and NK cell enrichment
To assess autophagy levels during decidualization, we compared the number of autophagy-related structures (autophagosomes and autolysosomes) and the expression of autophagy-related proteins (LC3 and SQSTM1/p62) between primary ESCs of secretory phase and DSCs by transmission electron microscopy and western blotting analysis, respectively. As shown, DSCs have increased number of autophagy structures () and ratio of membrane LC3 (LC3-II) to cytoplasmic LC3 (LC3-I), and lower level of autophagy negatively-related protein SQSTM1 () compared with ESCs. Similarly, the autophagy in the uterus of pregnant mice was significantly higher than that of non-pregnant mice (Figure S1A,B), suggesting that the autophagy level of stromal cells is increased during the process of endometrium decidualization in normal pregnancy. Intraperitoneal injection with 3-methyladenine (3-MA), an autophagy inhibitor, markedly suppressed endometrium autophagy (Figure S1C,D), and led to the increased ratio of embryo resorption in pregnant mice (), indicating that insufficient autophagy of decidualized stromal cells increases the risk of spontaneous abortion.
Figure 1. Decidualization is accompanied by enhanced autophagy and NK cell enrichment. (A) Primary cultured ESCs of secretory phase (n = 4) and DSCs (n = 5) were examined by transmission electron microscopy for the number of autophagic structures, including autophagosomes and autolysosomes (indicated by the red triangle). The quantity statistics were shown in (B). (C) The expression of autophagy-related proteins LC3-I, LC3-II and SQSTM1 in ESCs of secretory phase (n = 6) and DSCs (n = 6) was detected by western blotting assays. (D) The ratio of LC3-II to LC3-I was quantified. (E) After C57 pregnant mice were treated with PBS (n = 9) or 3-MA (n = 9, 100 mg/kg, twice a week), the number of embryos implanted and the embryo resorption rate of pregnant mice at the gestation of day 13.5 were quantified in (F). (G) In vitro cell adhesion assays were performed to analyze the adhesion of PKH67-labeled ESCs (n = 5) or DSCs (n = 5) to PKH26-labeled dNK cells. The number of adhered dNK cells was counted in (H). (I) RT-PCR was used to detect the expression levels of adhesion-related genes (SELE, SELP, SELL, VCAM1, ICAM2 and ICAM5) in ESCs (n = 5) and DSCs (n = 6). (J) Flow cytometry was used to detect the proportion and number of NK cells in the uterus of estrus (n = 8) and pregnant mice (n = 6) at the gestation of day 7.5. The statistical graph was displayed in (K). Data were presented as mean ± SEM or median and quartile and analyzed by t test. *P < 0.05, **P < 0.01, ***P < 0.001, ****P < 0.0001, NS: no significance
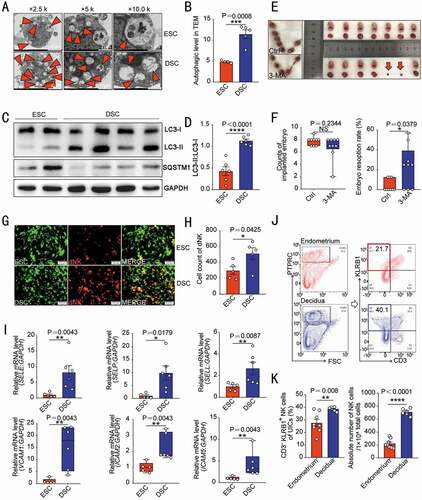
Data of in vitro cell adhesion assay showed DSCs had stronger adhesion to dNK cells than ESCs of secretory phase (). Compared with ESCs, DSCs expressed higher levels of adhesion-related genes, including SELE, SELP, SELL, VCAM1, ICAM2, and ICAM5 (). Further analysis showed that the percentage of uNK cells in uterine immune cells (UICs) and absolute number of uNK cells in pregnant mice were significantly higher than that in endometrium of estrous mice (). These results suggest that decidualization is accompanied by enhanced autophagy and adhesion ability to NK cell, contributing to the establishment and maintenance of pregnancy.
DSC autophagy promotes NK cells residence in decidua
As silencing ATG5 is more effective than ATG7 to impair autophagy during decidualization [Citation10], the ATG5-overexpressed ESCs (ATG5over ESC, Figure S2A) and ATG5-silenced DSCs (siATG5 DSC, Figure S2C) was constructed to investigate the relationship of ESC/DSC autophagy and cell adhesion ability. Notably, the expression adhesion-related genes (SELE, SELP, VCAM1, ICAM2, ICAM5 and CDH5) (Figure S2B) and cell adhesion ability to dNK () were increased in ATG5over ESC with high autophagy. In contrast, siATG5 DSC had low levels of adhesion-related genes (Figure S2D) and cell adhesion ability ().
Figure 2. DSC autophagy promotes NK cells residence in decidua. (A) Adhesion assays were performed on ATG5over ESCs (n = 6) or control ESCs (n = 6, GFP green fluorescence) and PKH-67-labeled dNK cells. The number of attached dNK cells was counted in (B). (C) Adhesion assays were performed on siATG5 ESCs (n = 5) or control ESCs (n = 5, GFP green fluorescence) and PKH67-labeled dNK cells. The number of adherent dNK cells was counted in (D). (E) The differential protein expression profile of ATG5over ESCs and control ESCs from protein microarray assay was shown, and differential expression of adhesion-related proteins were selected. (F) The expression levels of a series of adhesion molecules in DSCs of control group (n = 6) and 3-MA treatment group (n = 6, 10 mM, 48 h) were detected by flow cytometry. (G–J) C57 pregnant mice were injected intraperitoneally with PBS (n = 6) or 3-MA (n = 6, 100 mg/kg, twice a week), and the uterus of mice at the gestation of day 7.5 was collected. (G,H) The expression level of adhesion molecules on VIM+ USCs (uterine stromal cells) was evaluated by flow cytometry. (I,J) The proportion and absolute number of CD3− KLRB1+ NK cells in PTPRC+ uterine immune cells (UICs) was also detected by flow cytometry at the gestation of day 7.5. Data were presented as mean ± SEM or median and quartile and analyzed by t test. *P < 0.05, **P < 0.01, ***P < 0.001, ****P < 0.0001
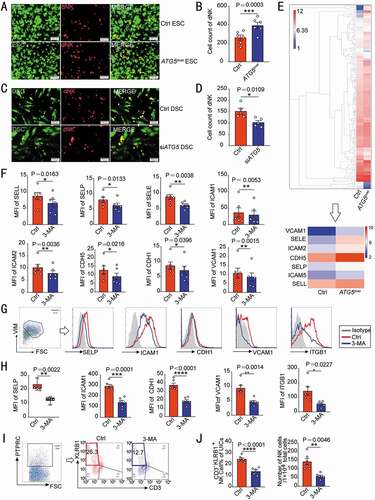
To investigate the potential effect of ESC autophagy on cell adhesion ability, a proteomic microarray was performed to evaluate the differential proteins of culture supernatant between Ctrl-ESC and ATG5over ESC. As shown, differential expression of seven kinds of protein (VCAM1, SELE, ICAM2, CDH5, SELP, ICAM5, and SELL) was observed (). Further analysis showed that treatment with 3-MA led to low levels of adhesion molecules on the surface of DSCs in vitro (). After 3-MA treatment, the expression of adhesion molecules on VIM (vimentin)+ uterine stromal cells (USCs) was obviously down-regulated (), and the proportion and absolute number of CD3− KLRB1/NK1.1+ NK cells in uterine PTPRC/CD45+ immune cells of pregnant mice decreased significantly (), which echoed in vitro results.
In term of the autophagy difference between peripheral blood NK (pNK) and dNK cells (), further investigation was carried out to rule out the direct effects of autophagy on adhesion and function of NK cells. As shown, there was no significant difference about the adhesion ability between 3-MA-pretreated dNK and control dNK cells in vitro (). Additionally, autophagy inhibition induced by 3-MA did not significantly influence the expression of adhesion molecules and cytotoxic activity-related molecules (NCR2/NKp44, FCGR3A/CD16, PRF1/perforin, KLRK1/NKG2D and KIR2DL1) of dNK cells (), indicating that autophagy is not involved in the adhesion regulation of NK cell directly. Collectively, these data suggest that DSC autophagy promotes DSC adhesion and NK cell residence in decidua during early pregnancy, and autophagy suppression results in the decreased adhesion of DSC, insufficient enrichment of dNK cell and increased embryo absorptions.
Figure 3. NK cell autophagy does not regulate its adhesion ability and cytotoxic activity-related molecules expression. (A) The autophagy structures in pNK (n = 6) and dNK cells (n = 6) were photographed using a transmission electron microscope. The number of autophagy structures was counted in (B). (C) The adhesion of dNK cells pre-treated with 3-MA (10 mM, 48 h, n = 7) or vehicle (1‰ PBS, n = 9) to DSCs was evaluated by in vitro adhesion assays. The number of adhered dNK cells was counted in (D). The expression of adhesion molecules (E) or functional molecules (F) on dNK cells treated with 3-MA (10 mM, 48 h, n = 7) or vehicle (1‰ PBS, n = 7) was analyzed by flow cytometry. Data were presented as mean ± SEM or median and quartile and analyzed by t test. *P < 0.05, *P < 0.01, NS: no significance
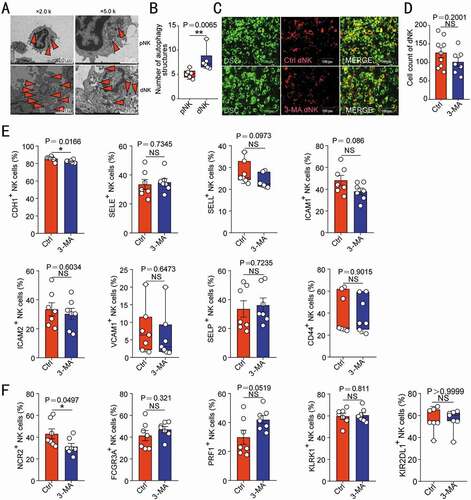
DSC autophagy-mediated NK cell residence is dependent on the MITF-TNFRSF14 pathway
Herpesvirus entry mediator (TNFRSF14/HVEM) belongs to tumor necrosis factor receptor superfamily member. Data of the proteomic microarray () and further experiments verified that TNFRSF14 was highly expressed in DSCs () and ATG5over ESC () compared with control ESCs, respectively. Of note, the autophagy inducer rapamycin markedly upregulated TNFRSF14 expression of DSCs (). To further investigate the potential role of TNFRSF14 in the autophagy-mediated cell adhesion, control DSC and siATG5 DSC were treated with the autophagy inducer rapamycin, and then in vitro cell adhesion assay and flow cytometry assay were performed to evaluate the adhesion ability of these DSCs to dNK cells and the expression of adhesion molecules on DSCs. As depicted in , rapamycin upregulated the adhesion of DSCs to dNK cells and the expression of adhesion molecules, however, TNFRSF14 silence partly or completely reversed these effects. These data above indicate that autophagy promotes the adhesion of DSCs to NK cells by upregulation of TNFRSF14.
Figure 4. DSC autophagy-mediated NK cell residence is dependent on the MITF-TNFRSF14 pathway. The transcription level or protein of TNFRSF14 in ESCs (n = 4) and DSCs (n = 4) were compared by RT-PCR (A) and western blotting (B). The transcription or protein level of TNFRSF14 in ATG5over (n = 4) and control ESCs (n = 4) was detected by RT-PCR (C) or western blotting (D). (E) RT-PCR was performed to detect the level of TNFRSF14 in vehicle (1‰ DMSO) or rapamycin-treated (2 µM, 48 h) control and siTNFRSF14 DSCs (n = 6 per group). (F) The adhesion ability of vehicle or rapamycin-treated DSCs and/or siTNFRSF14 DSCs (GFP green fluorescence) to PKH-67 labeled dNK cells was evaluated by in vitro cell adhesion assays (n = 6 per group). The statistical graph of the number of dNK cells adhered to each group was shown in (G). (H) The expression of adhesion-related genes in vehicle (1‰ DMSO), or rapamycin-treated (2 µM, 48 h) control and/or siTNFRSF14 DSCs (n = 6 per group) was detected by RT-PCR. (I) The transcription levels of MITF in ESCs (n = 5) and DSCs (n = 6) were detected by RT-PCR. (J) The expression level of MITF in siATG5 DSCs (n = 8) and control DSCs (n = 8) was analyzed by RT-PCR. (K) The protein level of TNFRSF14 in ATG5over ESCs (n = 4) or control ESCs (n = 4) was detected by western blotting. (L) Schematic diagram of the prediction of the binding region of the transcription factor MITF and wild-type or mutated TNFRSF14 promoter region. (M) Dual luciferase reporter assays were performed in HEK-293 T cells to verify the combination of MITF and wild-type or mutated TNFRSF14 promoter region. Data were presented as mean ± SEM or median and quartile and analyzed by t test or ANOVA. *P < 0.05, **P < 0.01, ***P < 0.001, ****P < 0.0001, NS: no significance
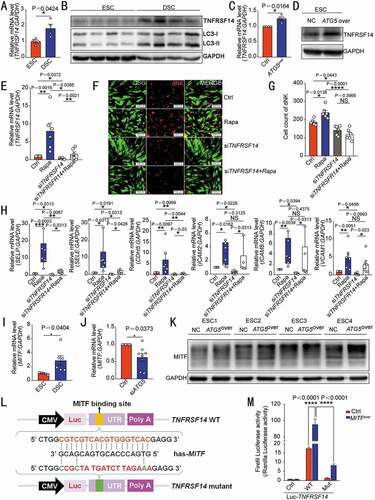
TNFSF14/LIGHT (TNF superfamily member 14), a well-known TNFRSF14 ligand, exhibits inducible expression and competes with other ligands for binding to TNFRSF14 [Citation22]. Interestingly, TNFSF14 was highly expressed on decidual immune cells (DICs) (Figure S3A) and dNK cells (Figure S3B–D). The data suggest that high expression of the autophagy and TNFSF14-TNFRSF14 axis in decidua should be involved in the interaction and crosstalk between NK cells and DSCs in early pregnancy. The inhibition (3-MA) or promotion (rapamycin) of autophagy level of dNK cells did not affect the expression of TNFSF14 (Figure S3E), indicating that TNFSF14 expression of dNK cells is independent on the autophagy level.
To further explore the possible mechanism of autophagy on TNFRSF14 in DSCs, Ingenuity Pathway Analysis (IPA) was used to predict the intermediate molecule between ATG5 and TNFRSF14. MITF (melanocyte inducing transcription factor) was then predicated to be the upstream molecule of TNFRSF14, and the downstream molecule of ATG5 (Figure S4A). Next, we observed that DSCs displayed high level of MITF compared with control ESCs (). ATG5 silence led to the down-regulation of MITF expression in DSCs (), whereas ATG5 overexpression obviously upregulation of MITF expression in ESCs (), confirming that MITF is indeed a transcription factor in ESC/DSC that can be positively regulated by autophagy. Considering that the combination of MITF and TNFRSF14 gene promoter region was be predicted, luciferase reporter assay was carried out. In HEK-293T cells, MITF overexpression promoted TNFRSF14 transcription by combing the −1375~−1392 sites in the TNFRSF14 promoter, however, the mutant TNFRSF14 could not be activated by the MITF (). Collectively, these results suggest that DSC autophagy promotes NK cell residence in decidua by the MITF-TNFRSF14 pathway.
It has been reported that TNFSF14 normalizes angiogenic blood vessels in tumor and induces high endothelial venules [Citation23]. However, TNFSF14-TNFRSF14 signaling has not been reported in vascular remodeling in pregnancy. In human decidual tissues during normal pregnancy, vascular endothelial cells expressed TNFRSF14 but rarely expressed TNFSF14 (Figure S5A). In view of the high expression of TNFRSF14 on HUVECs (human umbilical vein endothelial cells) (Figure S5B), HUVECs were treated with different concentrations of rh (recombinant human)-TNFSF14 (Figure S5C). Although the tube length was not changed significantly, the number of branch points was increased significantly in the middle and high concentration groups (Figure S5D). These data suggest that the TNFSF14-TNFRSF14 axis should be also involved in the formation of decidual blood vessels during pregnancy.
TNFRSF14 enhances adhesion ability of DSC through MMP9
To investigate the mechanism of TNFRSF14 on cell adhesion, IPA was then performed to analyze the relationship between TNFRSF14 and adhesion molecules (e.g. SELL, SELE, SELP, VCAM1, CD44, ICAM1, ICAM2 and ITGB1). As shown, MMP9 (matrix metalloproteinase 9) was predicted to be the central molecule for TNFSF14-TNFRSF14 signaling to regulate adhesion molecules (Figure S4B). Further analysis showed that rapamycin significantly increased MMP9 expression in DSCs, however, siTNFRSF14 could inhibit the expression of MMP9 and weaken the stimulatory effect of rapamycin on MMP9 (), suggesting that MMP9 is located downstream of TNFRSF14. Moreover, DSCs with higher autophagy expressed higher levels of MMP9 (), and ATG5 positively regulated MMP9 expression in ESCs and DSCs ().
Figure 5. TNFRSF14 enhances adhesion ability of DSC through MMP9. (A) MMP9 levels in vehicle or rapamycin-treated control or siTNFRSF14 DSCs (n = 6 per group) were analyzed by RT-PCR. (B) The transcription level of MMP9 in ESCs (n = 5) and DSCs (n = 6) was analyzed by RT-PCR. (C) The MMP9 transcription levels in ATG5over ESCs (n = 4), control ESCs (n = 4), siATG5 DSCs (n = 8) and control DSCs (n = 8) were analyzed by RT-PCR. (D) The level of MMP9 protein in ATG5over (n = 3) and control ESCs (n = 3) was detected by western blotting. (E) After treatment with different concentrations (0, 1, 10, 100, 1000 µM) of edaravone, the expression of MMP9 in DSCs (n = 6) was evaluated by western blotting. (F) The expression of adhesion molecules in DSCs (n = 6) after 1 mM edaravone treatment was analyzed by flow cytometry. (G) After treatment with C57 pregnant mice by vehicle (1% DMSO) (n = 5) or edaravone (n = 5, 2 mg/kg, daily). The embryo resorption rates of the two groups were compared (H) and the weight of the embryo and placenta was recorded (I) at the gestation of day 13.5. At the gestation of day 7.5, the proportion of PTPRC+ immune cells (J,K) in the uterus, the proportion and number of CD3− KLRB1+ NK cells (J,L) and the expression of adhesion molecules on VIM+ USCs (M,N) of pregnant mice were analyzed by flow cytometry (n = 7 per group). Data were presented as mean ± SEM or median and quartile and analyzed by t test or ANOVA. *P < 0.05, **P < 0.01, ***P < 0.001, ****P < 0.0001, NS: no significance
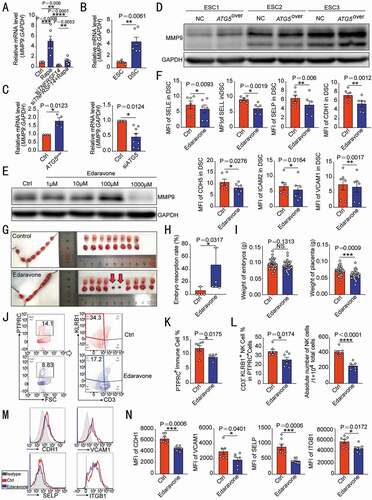
Next, an MMP inhibitor (edaravone, 1 mM) () was used to evaluate the effect of MMP9 on adhesion molecules of DSC. Under the interference of edaravone, the expression of a series of adhesion molecules on DSCs was significantly weakened (). In addition, application of edaravone in vivo led to increased embryo resorption rate and placental dysplasia in pregnant mice (). At gestation of day 7.5, poor residence of the uterine immune cells was observed (). In particular, the proportion of NK cells was decreased, and the absolute number has fallen by almost half (). Meanwhile, the expression of adhesion molecules was also significantly reduced in USCs (). These data suggest that the autophagy-MITF-TNFRSF14-triggered DSC adhesion to NK cells should be dependent on the MMP9.
Patients with unexplained spontaneous abortion display insufficient DSC autophagy and dNK residence
Compared with DSCs from women with normal pregnancy (NP), ATG5 and MAP1LC3B expression levels of DSCs from patients with unexplained spontaneous abortion (SA) were lower, whereas SQSTM1 levels were higher (). Additionally, the expression levels of autophagy downstream signaling molecules (MITF, TNFRSF14 and MMP9), in vitro adhesion ability to dNK and adhesion molecules of DSCs were also significantly reduced in SA group (). There was a lower density of NCAM1/CD56bright NK cells in decidua of SA group (). Previous studies revealed that among traditional dNK cells, which are identified as CD3− NCAM1+ cells, NCAM1+ KLRD1/CD94+ cells are recognized as bona fide NK cells, whereas NCAM1+ KLRD1− cells may include innate lymphoid cell (ILC)1 and NCR+ ILC3 distinct from “conventional NK” cells [Citation24,Citation25]. Among the decidual PTPRC+ immune cells, the proportion and absolute number of CD3− NCAM1+ cells in the SA group were significantly lower than those in the NP group (Figure S6A,B). There was not significant difference between the proportion of NCAM1+ KLRD1+ NK cells and NCAM1+ KLRD1− ILCs in the total CD3− NCAM1+ population (Figure S6A,C). The number of NK cells decreased significantly in SA group, but there was no difference in the number of ILCs (Figure S6D), indicating that the decrease of the density and number of CD3− NCAM1+ cells was mainly caused by the decrease of KLRD1+ NK cells without correlation with the number of ILCs.
Figure 6. Patients with unexplained spontaneous abortion display insufficient DSC autophagy and dNK residence. (A) The transcriptional levels of autophagy-related genes (ATG5, MAP1LC3B and STSQT1) in DSCs of normal early pregnant women (NP, n = 6) and patients with unexplained spontaneous abortion (SA, n = 4) were detected by RT-PCR. (B) The mRNA levels of MITF, TNFRSF14 and MMP9 in DSCs of NP group (n = 6) and SA group (n = 4) were detected by RT-PCR. (C) The adhesion ability of NP group or SA group DSCs to dNK cells from NP or SA group (n = 6 per group) was evaluated by in vitro adhesion assays. The statistical graph of the number of adherent dNK cells was presented in (D). (E) The adhesion molecules on the surface of DSCs in NP (n = 6) or SA (n = 6) group were analyzed by flow cytometry. The statistical graph was shown in (F). (G) The density of NCAM1+ NK cells in NP (n = 6) or SA (n = 6) tissues was observed by immunofluorescence. The number of NK cells in the decidua was counted in (H). (I,J) After C57 pregnant mice were treated with KLRB1 neutralizing antibody (n = 7, 15 mg/kg, every other day) or isotype control IgG antibody (n = 7, 15 mg/kg, every other day) by intraperitoneal injection, the depletion degree of uterine NK cells in pregnant mice at the gestation of day 7.5 was verified by flow cytometry. (K,L) The number of implanted embryos and the embryo resorption rate of pregnant mice at the gestation of day 13.5 were recorded and counted. Data were presented as mean ± SEM or median and quartile and analyzed by t test or ANOVA. *P < 0.05, **P < 0.01, ***P < 0.001
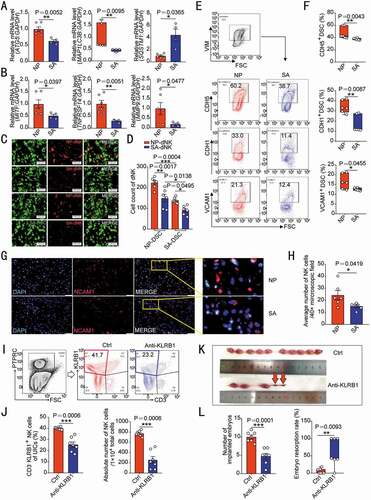
Specially, only uILC1s are identified in the decidua of pregnant mice, which can also express KLRB1 and were gated as EOMES− TBX21/T-bet+ [Citation26]. Here, we observed that the proportion of EOMES− TBX21+ ILC1 in C57 mouse uterus CD3− KLRB1+ cells (Figure S6E) was extremely low at gestation of day 7.5. For NK cell depletion, in vivo intraperitoneal injection of anti- KLRB1 neutralizing antibody in pregnant mice resulted in inadequate residence of NK cells in the uterine of pregnant mice, which was confirmed by the reduced proportion of NK cells in uterine immune cells and the number of NK cells (). In addition, NK cell depletion led to adverse pregnancy outcomes, including reduction of embryo implantation number and increase of embryo resorption rate (). Additionally, we detected the expression of PECAM1/CD31 (platelet and endothelial cell adhesion molecule 1) to evaluate placenta vascularization and coverage of ACTA2/α-SMA (actin alpha 2, smooth muscle)+ cells to analyze vessel subsequent maturation of placenta at gestation of day 13.5 [Citation27]. In NK depletion group, the fluorescence intensity of PECAM1 and coverage fraction of ACTA2+ cells were suppressed (Figure S7A,B), indicating that the sufficient density of NK cells in the decidua plays an important role in vascular remodeling and placental development. Collectively, these results indicate that insufficient DSC autophagy and NK cell residence contributes to the occurrence of spontaneous abortion.
Rapamycin prevents pregnancy loss by promoting DSC autophagy and NK cell residence in decidua
Owing to the positive regulatory effects of rapamycin on the MITF-TNFRSF14-MMP-adhesion molecules axis (), we further explored the potential value of rapamycin in preventing spontaneous abortion in vivo. As expected, spontaneous abortion mice models (female CBA/J mice were mated with male DBA/2 mice) displayed high embryo resorption rate compared with normal pregnancy models (female CBA/J mice were mated with male BALB/c mice). However, high dosage (1 mg/kg) of rapamycin resulted in severe embryo resorption (Figure S8A). Excitedly, low-dose (0.04 mg/kg) rapamycin effectively reversed embryo loss, and reached the level close to those of normal pregnant group (). Low doses of rapamycin did not affect the expression of placental PECAM1, but upregulated the coverage of ACTA2+ cells (Figure S8B,C). After in vitro treatment with different concentrations of rapamycin, the tube length and the number of branch points of HUVECs were not significantly inhibited at low concentrations, but began to be inhibited at medium and high concentrations (Figure S8D,E). Further analysis showed that there were decreased percentage and number of uNK cells, and expression of adhesion molecules in USC of spontaneous abortion mice models compared with normal pregnancy models, at gestation of day 7.5 (). However, low doses of rapamycin could notably increase the residence of NK cells and the expression of adhesion molecules in USC of spontaneous abortion mice models (). Similarly, EOMES− TBX21+ ILC1s in CBA/J mouse uterus PTPRC+ CD3− ITGA2+ cells (Figure S6F) were almost nonexistent. Taken together, these findings indicate that low doses of rapamycin promote DSC autophagy-mediated NK cell residence in decidua, and further decrease the risk of spontaneous abortion.
Figure 7. Rapamycin prevents pregnancy loss by promoting DSC autophagy and NK cell residence in decidua. After ESCs were treated with rapamycin (2 µM, 48 h), the transcriptional levels of MITF (n = 6), TNFRSF14 (n = 4) and MMP9 (n = 5) (A) and adhesion-related genes (n = 5) (B) were detected by RT-PCR. (C,D) At the gestation of day 13.5, embryo resorption rates were counted in normal pregnant mice (CBA/J♀×BABL/c♂, n = 6), spontaneous aborted mice (CBA/J♀×DBA/2♂) treated with vehicle (1% DMSO) (n = 4), or spontaneous aborted mouse models treated with rapamycin (n = 6, 0.04 mg/kg, three times a week). (E,F) Flow cytometry was used to detect the proportion and number of uterine CD3−ITGA2+ NK cells in PTPRC+ immune cells (n = 9) at the gestation of day 7.5. (G,H) The expression levels of adhesion molecules on VIM+ UCSs cells in the uterus of pregnant mice were also analyzed by flow cytometry (n = 9) at the gestation of day 7.5. Data were presented as mean ± SEM or median and quartile and analyzed by t test or ANOVA. *P < 0.05, **P < 0.01, ***P < 0.001, ****P < 0.0001, NS: no significance
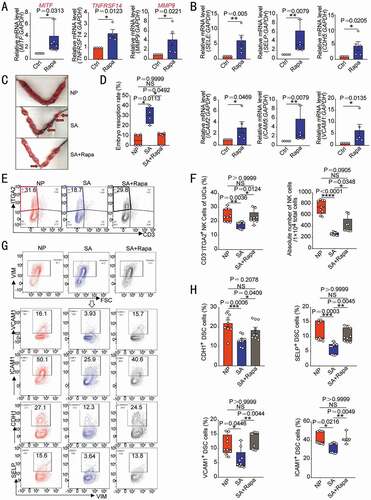
Discussion
Endometrial cells undergo a dynamic autophagy in the periodic growth and shedding off during the menstrual cycle, and the change of autophagy level is associated with physiological processes including decidualization and atrophy [Citation28]. Autophagy inhibition results in abnormal decidualization [Citation9,Citation10]. In this article, we have found that autophagy is highly activated in the decidualized endometrium of early pregnancy. Inadequate autophagy of decidualized stromal cells contributes to increased risk of spontaneous abortion.
Autophagy at the maternal-fetal interface constitutively exists in in both villous and decidua tissues in normal early pregnancy [Citation29], which was also reported to exhibit a dynamic pattern during human pregnancy [Citation30]. Autophagy should be critical to deal with the high energetic requirements that the decidual program demands via recycling metabolites that would need energy-expensive biosynthesis. Moreover, autophagy could contribute to the survival of decidual cells under the low oxygen and low-nutrient conditions of the maternal-fetal interface [Citation31]. Autophagy induction of early pregnancy also preserves trophoblast function in the low oxygen and nutrient placental environment [Citation32]. Interestingly, our results of the proteomic microarray and further analysis confirm autophagy significantly upregulates the expression of adhesion molecules (VCAM1, SELE, ICAM2, CDH5, SELP, ICAM5, and SELL) on ESCs and DSCs in vitro and in vivo, and DSCs with high autophagy present strong adhesion to NK cells, and accelerate the residence and enrichment of dNK cells during early pregnancy. Depletion or absence of NK cells resulted in adverse outcomes (the decreased number of embryo implantation, increased embryo resorption and placental angiogenesis disorder) of pregnant mice, emphasizing that the important role of NK cells in the establishment and maintenance of normal pregnancy.
Recently, more attention is paid to the effects of autophagy of nonimmune cells on local immune cells and immune microenvironment. Autophagy inhibits the anticancer immune response by reducing the efficacy of NK cell-mediated lysis by degrading granzyme B, contributing to less sensitivity and stronger immune escape ability of hypoxic tumor cells to NK-mediated killing [Citation33]. Our previous study has shown the endometriotic ESC autophagy under negatively control of estrogen induces the differentiation of FCGR3A− NK cells with lower cytotoxicity, which is beneficial to development of endometriosis [Citation34–36]. Here we observed that the autophagy level of pNK cells was much lower than that of dNK, it is noteworthy that autophagy does not seem to be directly involved in the regulation of NK cell adhesion and cytotoxic activity. Along with blastocyst implantation, it is widely recognized that embryonic trophoblast cells secrete large amounts of CXCL12 at the maternal-fetal interface, which recruit and promotes the adhesion ability of NCAM1brigh NK cells to DSCs in a CD82/ITGB1-dependetnt manner [Citation37,Citation38]. In this study, we found that decidualization was accompanied with the increase of autophagy, which might be due to the positive role of a significant increase in progesterone during early pregnancy [Citation34]. However, the molecular mechanism is needed to further research. Additionally, we found for the first time that DSCs autophagy promoted NK cell adhesion and retention in decidua, suggesting that the infiltration and residence of NK cell in decidua of early pregnancy, is not a simple passive receiving process for decidua, but an active participation and regulation process through DSC autophagy.
MITF belongs to the Microphthalmia family of bHLH-LZ transcription factors (MiT-TFE) of the helix-loop-helix leucine-zipper family. Recent studies have shown that these transcription factors function as the master regulators of autophagosome and lysosome biogenesis [Citation39,Citation40]. Here we observed that MITF could be positively regulated by autophagy and ATG5. DSC autophagy upregulated MITF expression, thereby initiating and upregulating the transcription and expression of TNFRSF14. TNFRSF14 is a co-signaling molecular switch by interacting with its binding partners, TNFSF14, BTLA (B and T lymphocyte attenuator) and CD160 [Citation41]. At the maternal-fetal interface, we observed that TNFSF14 was highly expressed DIC, especially dNK cells, and TNFRSF14 was enriched in DSCs with an activate autophagy level. Further analysis TNFRSF14 upregulated the expression of adhesion molecules (e.g. SELE, SELP, VCAM1, ICAM1and ICAM2) and promoted the adhesion of DSC to NK cell by the MMP9. Both embryonic and maternal MMP9 are major contributors to decidual remodeling, blastocyst implantation, and placenta development [Citation42]. LIGHT has been reported to significantly promote intercellular adhesion and cell surface expression of ICAM1 upon interaction with basophils or eosinophils via cell surface receptors TNFRSF14 and LTβR on BEAS-2B cells [Citation43]. In addition, recombinant TNFSF14 can induce MMP9 expression of synovial fibroblasts in an NFKB/NF-κB-dependent fashion [Citation44]. From the above findings, it is not difficult to infer that TNFSF14 and TNFRSF14 signals should be involved in the upregulation of adhesion ability of DSCs by the MMP9-adhesion molecules pathway. Inhibitor of MMP9 led to impaired adhesion of DSC and residence of dNK, and increased embryo loss. These data suggest that the autophagy-TNFSF14-TNFRSF14-MMP9 axis connects the dialogue between DSCs and dNK cells, contributing to decidual remodeling, dNK cells residence, and immune hemostasis for normal pregnancy. Moreover, TNFSF14-TNFRSF14 signaling may also benefit pregnancy for promoting placenta angiogenesis, which is consistent with previous researches and need further investigation [Citation23].
Autophagy induction has been reported to degrade maternal factors essential for early pregnancy in animal [Citation45]. Previous reports showed that intrauterine injection of rapamycin (10 mg/kg) impaired endometrial cell decidualization and embryo implantation [Citation46], whereas subcutaneous injection of rapamycin (2 mg/kg) reversed the decreased endometrial apoptosis under folate deficiency during decidualization [Citation11]. Owing to inadequate DSC autophagy and dNK residence of patients with unexplained spontaneous abortion, we further evaluated the potential therapeutic value of rapamycin (an autophagy inducer via inhibition of MTOR [mechanistic target of rapamycin kinase]) in spontaneous abortion. In this study, intraperitoneal injection of low-dose rapamycin (0.04 mg/kg) displayed a protective effect of decidual autophagy and NK cell residence, and the ability to rescue pregnancy loss without disruption of the placental blood vessel development, and this process should be dependent on the regulation of the MITF-TNFRSF14-MMP9 axis. As a double-edged sword, autophagy is related to both cell survival and apoptosis. Of note, we observed the high dosage of rapamycin (intraperitoneal injection, 1 mg/kg) led to a significant increase of adverse pregnancy outcomes. These findings indicate that the usage and dosage of rapamycin is the key to determining whether it is protective or harmful to normal pregnancy. Therefore, the therapeutic value and safety of rapamycin in the prevention and treatment of spontaneous abortion needs further exploration.
Collectively, as shown in , during decidualization, the increased autophagy promotes the expression of adhesion molecules on the surface of DSCs via the MITF-TNFRSF14-MMP9 axis, thereby facilitating the adhesion and retention of NK cells in decidua, vascular remodeling, fetal and placental development of early pregnancy. Insufficient autophagy of DSCs results in the decrease of dNK cell residence, contributing to spontaneous abortion. The potential therapeutic value of low-dose rapamycin in spontaneous abortion should be emphasized due to the activation of DSC autophagy-MITF-TNFRSF14-MMP9-adhension molecules-mediated NK cell residence during early pregnancy.
Figure 8. Schematic roles of rapamycin in preventing spontaneous abortion by triggering DSC autophagy-mediated NK residence during early pregnancy. Decidualization and establishment of normal pregnancy are accompanied by enhanced autophagy of DSCs and enrichment of dNK cells. High level of autophagy in DSCs increases the expression of the transcription factor MTIF. MITF enters into nucleus, binds to the promoter region of TNFRSF14 and positively regulates its transcription. TNFSF14, the TNFRSF14 ligand, is expressed on dNK cells. The TNFSF14-TNFRSF14 signal should contribute to the increases of adhesion molecules and the adhesion ability by upregulation of MMP9 expression. The strong adhesion of DSCs facilitates the localization and enrichment of NK cells in decidua. NK cells with sufficient density in decidua are beneficial to vascular remodeling, placental development, embryo growth, and maintenance of pregnancy. Spontaneous abortion of early pregnancy is associated with low levels of DSC autophagy, inactivation of the MITF-TNFRSF14-MMP9 axis, and insufficient NK cell residence. Autophagy inhibitor 3-MA or MMP9 inhibitor edaravone increases the risk of spontaneous abortion. The autophagy inducer rapamycin releases the inhibitory effect of MTOR on autophagy by inhibiting MTOR, and initiates the enhancement of DSC adhesion associated with MITF-TNFRSF14-MMP9 axis and more dNK cell residence, and prevents spontaneous abortion
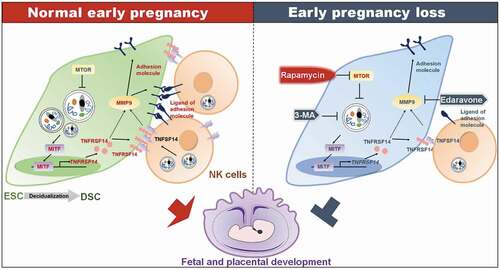
Materials and methods
Tissues
All tissues were collected with permission of ethics committee and consent of the patients from Obstetrics and Gynecology Hospital of Fudan University. Endometrial tissues (n = 36) were collected from women with normal pregnancy history of reproductive ages (25–40 years old), who were undergoing hysterectomy or diagnostic curettage for benign reasons unrelated to endometrial dysfunction. All samples were evaluated by a histopathologist to exclude endometrial pathology and identify the cyclic phase as secretory phase. None of them had received hormonal medication in the 6 months before the surgical procedure. Decidual tissues were from women (n = 86) in normal first-trimester pregnancy for selective termination or patients (n = 30) with unexplained spontaneous abortion caused by non-genetic or non-endocrine factors (age, 21–35 years old; gestational age, 7–9 weeks). All the tissues were collected under sterile conditions and transported to the laboratory on ice within 30 min in Dulbecco’s modified Eagle’s medium (DMEM)/F‐12 (HyClone, SH30023.01B) with 10% fetal bovine serum (FBS; Gibco, 26140-079) for further isolating ESCs, DSCs, and dNK cells. Peripheral blood samples (n = 12) were from women in normal first-trimester pregnancy (age, 21–35 years old; gestational age, 7‐9 weeks). Samples were taken sterilely in heparinized Hanks’ buffer solution (Gibco, 15630-080) and were transported to the laboratory on ice for sorting of peripheral blood mononuclear cells (PBMCs) and peripheral NK (pNK) cells. ESCs, DSCs, pNK and dNK cells were isolated and cultured according to our previous protocols [Citation47].
Mice
C57 mice (5 to 7 weeks-old) were from Shanghai SLAC Laboratory Animal Co., Ltd. (China). After 3 weeks of adaptive feeding, female mice were mated with male mice (2:1) to construct pregnancy mouse models. Pregnant mice were injected with 3-MA (100 mg/kg, twice a week; Sigma-Aldrich, 5142-23-4) or edaravone (2 mg/kg, daily; MCE, HY-B0099) intraperitoneally. The day when the vaginal hydrant was detected was the day 0 of gestation. Uteri were collected at the gestation of day 7.5 or 13.5, and then the pregnancy outcome was observed or the uterus was cut and digested into a single-cell suspension for future flow cytometry assays. The mice in the control group were given the same amount of drug solvent PBS (HyClone, SH30256.01) or DMSO (1%; Sigma-Aldrich, 67-68-5). In order to deplete uterine NK cells in mice, pregnant mice were injected anti-KLRB1/NK1.1 neutralizing antibody (15 mg/kg, every other day; BioLegend, 108760) intraperitoneally. At the gestation of day 7.5, the proportion and number of uterine NK cells in mice and the expression of adhesion molecules on USCs were measured by flow cytometry assays; at the gestation of day 13.5, the number of embryos and resorption rate were counted and the placentas were acquired. The mice in the control group were treated with the same amount of isotype control antibody (BioLegend, 400282).
5 to 7 weeks-old female CBA/J, male DBA/2 and male BALB/c mice were all from Beijing Huafukang Biotechnology Co., Ltd. (China). After adaptive feeding, female CBA/J mice were mated with male BALB/c mice or male DBA/2 mice (2:1) to construct normal pregnancy mouse models or spontaneous abortion mouse models. Spontaneous abortion mice (female CBA/J × male DBA/2) were treated with rapamycin (0.04 mg/kg or 1 mg/kg, three times a week; MCE, HY-10219) or vehicle by intraperitoneal injection, and normal pregnancy mice (female CBA/J ×male BALB/c mice) were also treated with vehicle. As mentioned above, the proportion and number of uterine NK cells in mice and the expression of adhesion molecules on USC were measured, embryos resorption rate were counted, and the placentas were acquired, accordingly.
Cell treatment and transfection
DSCs or dNK cells were treated with or without an autophagy inhibitor 3-methyladenine (3-MA, 10 mM; Sigma-Aldrich, 5142-23-4), an autophagy inducer rapamycin (Rapa, 2 µM; Sigma-Aldrich, 53123-88-9) or a MMP9 inhibitor edaravone (1 mM; MCE, HY-B0099) for 48 h, or ESCs were treated with or without rapamycin (2 µM; Sigma-Aldrich, 53123-88-9) for 48 h, and then the transcriptional levels and protein levels of specific molecules or the adhesion of stromal cells to NK cells were detected. NK cells were treated with or without 3-MA (10 mM; Sigma-Aldrich, 5142-23-4) for 48 h to suppress autophagy for subsequent flow cytometry assays and adhesion assays.
ATG5 overexpression (ATG5over) plasmid, control plasmid, recombinant lentivirus of siRNA targeting ATG5 (siATG5) or TNFRSF14 (siTNFRSF14) and control lentivirus with GFP was all from Shanghai Genechem Co., LTD. (China). ESCs were seeded in 6-well culture plates and subsequently infected with ATG5over or control plasmid at 50% confluence for 48 h. DSCs were seeded in 6-well culture plates and subsequently infected with siATG5, siTNFRSF14 or control lentivirus at 30% confluence. Twelve h after infection, fresh complete culture medium was used to continue the culture. The infection efficiency was evaluated by GFP fluorescence intensity and RT-PCR 72 h and 96 h after infection.
Transmission electron microscopy
Purified ESCs, DSCs, pNK or dNK cells were collected and fixed in 2.5% glutaraldehyde. All samples were post-fixed in 1% osmium tetroxide, dehydrated in an ascending series of alcohols, and then embedded in epoxy resin (Sigma-Aldrich, 45345). The ultrathin sections were cut and stained with uranyl acetate and lead citrate. The autophagic structures in cells were examined under a Philips CM-120 transmission electron microscope (Philips, CM-120).
Western blotting
ESCs or DSCs were lysed in high efficiency cell tissue rapid lysis buffer (RIPA; Beyotime, P0013B) containing 1% phenylmethanesulfonylfluoride (PMSF; Beyotime, ST506) proteinase, detached with a cell scraper and centrifuged for 30 min at 12,000 × g at 4°C. Protein concentrations were quantified using the BCA protein assay kit (Beyotime, P0012). Cell lysates were boiled for 10 min at 95°C for long-term preservation. Protein (25 µg) was electrophoresed in SDS-PAGE gels (Epizyme Biotech, PG113) using a Miniprotein III system (Bio-Rad, 1658033), transferred to PVDF membranes (Millipore, ISEQ00010) for 90 min, and then incubated in 5% skimmed milk in PBST (Beyotime, P0222) at room temperature for 1 h. The PVDF membranes were overnight incubated with primary antibody against MAP1LC3B/LC3B (1:3000; Novusbio, NB100-2220), SQSTM1/p62 (1:1000; Cell Signaling Technology, 39749), TNFRSF14/HVEM (1:500; Abcam, ab185711), MITF (1:1000; Cell Signaling Technology, 97800), MMP9 (1:3000; Abcam, ab76003) and GAPDH (1:3000; Affinity, AF0911) at 4°C. Then PVDF membranes were washed with PBST solution (Beyotime, P0222) and incubated at room temperature for 1 h in peroxidase-conjugated goat anti-rabbit IgG secondary antibody (1:5000; Bioworld Technology, BS10003) or goat anti-mouse IgG secondary antibody (1:5000; Bioworld Technology, BS10003). After washed three times, the PVDF membranes was for chemiluminescence using the Immobilon Western Chemiluminescent HRP Substrate Kit (Millipore, WBKLS0100).
Cell adhesion assays
Stromal cells after different treatments or lentivirus infection were collected, washed, and cultured in a 96-well plate (5 × 104 cells per well) with or without staining with PKH67 (Sigma-Aldrich, PKH67GL) for overnight. PKH26 (Sigma-Aldrich, PKH26GL)-labeled dNK cells were co-cultured with ESCs or DSCs for 24 h. Then, to remove unattached dNK cells, the media was removed, and cells were washed gently with PBS twice. Pictures were obtained under a fluorescent microscope (Olympus) at five random fields of view and showed as the ratio of dNK cells to the stromal cell layer.
Real time (RT)-PCR
Total RNA was extracted by the TRIzol® reagent (Invitrogen, 15596018). Then RNA was reverse-transcribed into cDNA using the Prime Script RT reagent Kit (Takara, RR036A). RT-PCR was performed using SYBR Premix Ex Taq (Takara, RR820A) and analyzed using an ABI Prism 7900 Fast Sequence Detection system (Thermo Fisher Scientific). The fold change in the transcriptional expression of the above genes was calculated using the 2− ΔΔCt method and each sample was analyzed in three replicate wells. Relative mRNA expression levels were normalized to GAPDH. The primer sequences of these genes are provided in Table S1.
Flow cytometry assays
Human and mouse antibodies for flow cytometry assays () were used for measurement of cell markers. Matched immunoglobulin G (IgG) antibodies were used as isotype controls. Flow cytometry assays were performed using according to the manufacturer’s instructions. Cell sorting was conducted with a Beckman CytoFLEX S flow cytometer (Beckman) using Becton CytExpert software. Data were analyzed using FlowJo V10 software.
Table 1. Antibodies for flow cytometry
Immunohistochemistry and immunofluorescence
Paraffin sections were technically supported by Wuhan Servicebio Technology Co., Ltd. (China). Uterus samples from pregnant or non-pregnant mice were incubated with rabbit anti-mouse LC3B antibody (1:200; Novus Biologicals, NB100-2220) or rabbit anti-mouse MTOR antibody (1:400; Cell Signaling Technology, 2983) overnight at 4°C in a humid chamber. After washing three times with TBS (Beyotime, ST661), the sections were incubated with dunkey anti-rabbit IgG H&L (Alexa Fluor® 594) preadsorbed antibody (1:400; Yeasen, 34212ES60). Placenta samples from NK cell depletion pregnant mice, low doses of rapamycin-treated pregnant mice or control mice were incubated with rabbit anti-mouse PECAM1/CD31 antibody (1:50; Abcam, ab28364) or mouse anti-mouse ACTA2/α-SMA antibody (0.034 µg/mL; Abcam, ab7817) overnight at 4°C in a humid chamber. After washing three times with TBS (Beyotime, ST661), the sections were incubated with goat anti-rabbit IgG H&L (Alexa Fluor® 488) preadsorbed antibody (1:400; Abcam, ab150077) and goat anti-mouse IgG H&L (Alexa Fluor® 594) preadsorbed antibody (1:400; Abcam, ab150116). And the nuclei were stained with 4ʹ,6-diamidino-2-phenylindole (DAPI; Beyotime, C1006).
Human decidua samples were incubated with mouse anti-human NCAM1/CD56 antibody (1:200; Abcam, ab9018) overnight at 4°C in a humid chamber and then were incubated goat anti-mouse IgG H&L (Alexa Fluor® 594) preadsorbed antibody (1:400; Abcam, ab150116) after washing with TBS (Beyotime, ST661). And the nuclei were stained with 4ʹ,6-diamidino-2-phenylindole (DAPI; Beyotime, C1006). Human decidua samples were incubated with rabbit anti-human TNFRSF14/HVEM antibody (1:1000; Abcam, ab185711) or goat anti-human TNFSF14/LIGHT antibody (1:1000; R&D Systems, AF664) overnight at 4°C in a humid chamber. After washing three times with TBS (Beyotime, ST661), the sections were incubated and the signal was visualized with GTvision TMIII Immunohistochemical Detection Kit (Gene Tech, GK500710). The sections were counterstained with hematoxylin (Well Biotech, WH2013).
Protein microarray
After transfection of ATG5 overexpression plasmid (ATG5over) or control plasmid (Ctrl) for 48 h, culture medium of ESCs was replaced with fresh medium containing 10% FBS (Gibco, 26140-079) and 1% tertiary antibody (Gibco, 15240-062). ESCs were cultured for another 24 h. The culture supernatant was collected and carefully drawn after centrifugation to 1.5 mL centrifuge tube, and further analyzed by Shanghai Wayen Biomedical Technology Co., Ltd. (China) using the proteomic microarray RayBio® L-Series Human Antibody Array 1000 (RayBiotech Inc.) according to our previous protocols [Citation30].
Dual luciferase reporter assays
The NC mimics, MITF overexpressed mimics, TNFESR14 and TNFRSF14 mutation luciferase reporter plasmids were constructed by Shanghai Genechem Co., Ltd. (China). Plasmids were transfected into HEK-293T cells using PolyFect Transfection Reagent (Qiagen, 301105). The luciferase activity was analyzed by the Dual-Luciferase® Reporter (DLR™) Assay (Promega, e1910).
Tube formation assays
HUVECs were purchased from ATCC (USA, CRL-1730). After 48-h treatment of recombinant human (rh)-LIGHT (0, 1, 10, 100 ng/mL; BioLegend, 702402) or rapamycin (0, 0.4, 2, 10 µM; Sigma-Aldrich, 53123-88-9), HUVECs were collected and labeled with PKH-26 dye (Sigma-Aldrich, PKH26GL). Matrigel Matrix (Corning, 356234) was placed on ice to dissolve, and a pre-chilled pipette tip was used to suck 100 µL of Matrigel (Corning, 356234) into the pre-chilled 96-well plate. After incubation at 37°C for 1 h for solidification, HUVECs were added to 96-well plates at 1.5 × 104 cells/50 µL. Tube formation pictures were taken by microscope after 4–6 h of cultivation. The tube length and numbers of branch points were analyzed with ImageJ (NIH).
Statistics analyses
All analyses were conducted by SPSS 25 (IBM). Between two groups, a paired or unpaired t test with or without Wilcoxon matched‐pairs signed‐rank test or a Mann‐Whitney U test was used according to the analysis of normal distribution and variance homogeneity. Among multiple groups, a one-way ANOVA test with or without a Kruskal-Wallis test according to the analysis of normal distribution and variance homogeneity. Each experiment was repeated 3 times, and data were presented as mean ± standard error (SEM) or median and quartile for normally distributed or other data. P < 0.05 was considered to indicate a statistically significant difference.
Supplemental Material
Download Zip (3.4 MB)Acknowledgments
We thank Dr. Feng-Qing Dong from Shanghai Genechem Co., LTD. for help with bioinformatics analysis, and Dr. Yu-Qing Qu in the Department of Pathology, Hospital of Obstetrics and Gynecology, Shanghai Medical School, Fudan University for helping with histological analysis. We are grateful to Dr. Jiang-Feng Ye from Division of Obstetrics and Gynecology, KK Women’s and Children’s Hospital, Singapore, for analyzing the data.
Disclosure statement
The authors declare that they have no competing interests.
Supplementary material
Supplemental data for this article can be accessed here.
Additional information
Funding
References
- Weselak M, Arbuckle TE, Walker MC, et al. The influence of the environment and other exogenous agents on spontaneous abortion risk. J Toxicol Environ Health B Crit Rev. 2008;11:221–241.
- Eschenbach DA. Treating spontaneous and induced septic abortions. Obstet Gynecol. 2015;125:1042–1048.
- Gellersen B, Brosens JJ. Cyclic decidualization of the human endometrium in reproductive health and failure. Endocr Rev. 2014;35:851–905.
- Lucas ES, Dyer NP, Murakami K, et al. Loss of endometrial plasticity in recurrent pregnancy loss. Stem Cells. 2016;34:346–356.
- Kajihara T, Tanaka K, Oguro T, et al. Androgens modulate the morphological characteristics of human endometrial stromal cells decidualized in vitro. Reprod Sci. 2014;21:372–380.
- Devis-Jauregui L, Eritja N, Davis ML, et al. Autophagy in the physiological endometrium and cancer. Autophagy. 2020:1–19. DOI:https://doi.org/10.1080/15548627.2020.1752548
- Mizushima N, Komatsu M. Autophagy: renovation of cells and tissues. Cell. 2011;147:728–741.
- Choi J, Jo M, Lee E, et al. The role of autophagy in human endometrium. Biol Reprod. 2012;86:70.
- Su Y, Zhang JJ, He JL, et al. Endometrial autophagy is essential for embryo implantation during early pregnancy. J Mol Med (Berl). 2020;98:555–567.
- Mestre Citrinovitz AC, Strowitzki T, Germeyer A. Decreased autophagy impairs decidualization of human endometrial stromal cells: a role for ATG proteins in endometrial physiology. Int J Mol Sci. 2019;20:3066.
- Chen Q, Gao R, Geng Y, et al. Decreased autophagy was implicated in the decreased apoptosis during decidualization in early pregnant mice. J Mol Histol. 2018;49:589–597.
- Erlebacher A. Immunology of the maternal-fetal interface. Annu Rev Immunol. 2013;31:387–411.
- Vacca P, Moretta L, Moretta A, et al. Origin, phenotype and function of human natural killer cells in pregnancy. Trends Immunol. 2011;32:517–523.
- Gamliel M, Goldman-Wohl D, Isaacson B, et al. Trained memory of human uterine NK cells enhances their function in subsequent pregnancies. Immunity. 2018;48:951–962.e5.
- Fu B, Zhou Y, Ni X, et al. Natural killer cells promote fetal development through the secretion of growth-promoting factors. Immunity. 2017;47:1100–1113.e6.
- Santoni A, Carlino C, Stabile H, et al. Mechanisms underlying recruitment and accumulation of decidual NK cells in uterus during pregnancy. Am J Reprod Immunol. 2008;59:417–424.
- Castriconi R, Carrega P, Dondero A, et al. Molecular mechanisms directing migration and retention of natural killer cells in human tissues. Front Immunol. 2018;9:2324.
- Mokhtar NM, Cheng CW, Cook E, et al. Progestin regulates chemokine (C-X-C motif) ligand 14 transcript level in human endometrium. Mol Hum Reprod. 2010;16:170–177.
- Godbole G, Modi D. Regulation of decidualization, interleukin-11 and interleukin-15 by homeobox A 10 in endometrial stromal cells. J Reprod Immunol. 2010;85:130–139.
- Jones RL, Hannan NJ, Kaitu’u TJ, et al. Identification of chemokines important for leukocyte recruitment to the human endometrium at the times of embryo implantation and menstruation. J Clin Endocrinol Metab. 2004;89:6155–6167.
- Croy BA, van den Heuvel MJ, Borzychowski AM, et al. Uterine natural killer cells: a specialized differentiation regulated by ovarian hormones. Immunol Rev. 2006;214:161–185.
- Holmes TD, Wilson EB, Black EV, et al. Licensed human natural killer cells aid dendritic cell maturation via TNFSF14/LIGHT. Proc Natl Acad Sci U S A. 2014;111:E5688–E5696.
- He B, Jabouille A, Steri V, et al. Vascular targeting of LIGHT normalizes blood vessels in primary brain cancer and induces intratumoural high endothelial venules. J Pathol. 2018;245:209–221.
- Fuchs A, Vermi W, Lee JS, et al. Intraepithelial type 1 innate lymphoid cells are a unique subset of IL-12- and IL-15-responsive IFN-γ-producing cells. Immunity. 2013;38:769–781.
- Vacca P, Montaldo E, Croxatto D, et al. Identification of diverse innate lymphoid cells in human decidua. Mucosal Immunol. 2015;8:254–264.
- Doisne JM, Balmas E, Boulenouar S, et al. Composition, development, and function of uterine innate lymphoid cells. J Immunol. 2015;195:3937–3945.
- Sun Y, Han Y, Qian M, et al. Defending effects of iodide transfer in placental barrier against maternal iodine deficiency. Thyroid. 2020. DOI:https://doi.org/10.1089/thy.2020.0510
- Yang S, Wang H, Li D, et al. Role of endometrial autophagy in physiological and pathophysiological processes. J Cancer. 2019;10:3459–3471.
- Avagliano L, Terraneo L, Virgili E, et al. Autophagy in normal and abnormal early human pregnancies. Reprod Sci. 2015;22:838–844.
- Choi S, Shin H, Song H, et al. Suppression of autophagic activation in the mouse uterus by estrogen and progesterone. J Endocrinol. 2014;221:39–50.
- Yamanaka-Tatematsu M, Nakashima A, Fujita N, et al. Autophagy induced by HIF1alpha overexpression supports trophoblast invasion by supplying cellular energy. PLoS One. 2013;8:e76605.
- de Andrade Ramos BR, Witkin SS. The influence of oxidative stress and autophagy cross regulation on pregnancy outcome. Cell Stress Chaperones. 2016;21:755–762.
- Baginska J, Viry E, Berchem G, et al. Granzyme B degradation by autophagy decreases tumor cell susceptibility to natural killer-mediated lysis under hypoxia. Proc Natl Acad Sci U S A. 2013;110:17450–17455.
- Mei J, Zhu XY, Jin LP, et al. Estrogen promotes the survival of human secretory phase endometrial stromal cells via CXCL12/CXCR4 up-regulation-mediated autophagy inhibition. Hum Reprod. 2015;30:1677–1689.
- Mei J, Zhou WJ, Zhu XY, et al. Suppression of autophagy and HCK signaling promotes PTGS2high FCGR3− NK cell differentiation triggered by ectopic endometrial stromal cells. Autophagy. 2018;14:1376–1397.
- Zhang B, Zhou WJ, Gu CJ, et al. The ginsenoside PPD exerts anti-endometriosis effects by suppressing estrogen receptor-mediated inhibition of endometrial stromal cell autophagy and NK cell cytotoxicity. Cell Death Dis. 2018;9:574.
- Hanna J, Wald O, Goldman-Wohl D, et al. CXCL12 expression by invasive trophoblasts induces the specific migration of CD16− human natural killer cells. Blood. 2003;102:1569–1577.
- Lu H, Jin LP, Huang HL, et al. Trophoblast-derived CXCL12 promotes CD56bright CD82− CD29+ NK cell enrichment in the decidua. Am J Reprod Immunol. 2020.
- Sardiello M, Palmieri M, Di Ronza A, et al. A gene network regulating lysosomal biogenesis and function. Science. 2009;325:473–477.
- Settembre C, Di Malta C, Polito VA, et al. TFEB links autophagy to lysosomal biogenesis. Science. 2011;332:1429–1433.
- Rodriguez-Barbosa JI, Schneider P, Weigert A, et al. HVEM, a cosignaling molecular switch, and its interactions with BTLA, CD160 and LIGHT. Cell Mol Immunol. 2019;16:679–682.
- Plaks V, Rinkenberger J, Dai J, et al. Matrix metalloproteinase-9 deficiency phenocopies features of preeclampsia and intrauterine growth restriction. Proc Natl Acad Sci U S A. 2013;110:11109–11114.
- Qiu HN, Wong CK, Dong J, et al. Effect of tumor necrosis factor family member LIGHT (TNFSF14) on the activation of basophils and eosinophils interacting with bronchial epithelial cells. Mediators Inflamm. 2014;2014:136463.
- Pierer M, Brentano F, Rethage J, et al. The TNF superfamily member LIGHT contributes to survival and activation of synovial fibroblasts in rheumatoid arthritis. Rheumatology (Oxford). 2007;46:1063–1070.
- Tsukamoto S, Kuma A, Murakami M, et al. Autophagy is essential for preimplantation development of mouse embryos. Science. 2008;321:117–120.
- Chen X, He J, Ding Y, et al. The role of MTOR in mouse uterus during embryo implantation. Reproduction. 2009;138:351–356.
- Yang HL, Zhou WJ, Lu H, et al. Decidual stromal cells promote the differentiation of CD56brightCD16−NK cells by secreting IL-24 in early pregnancy. Am J Reprod Immunol. 2019;81:e13110.