Abstract
Introduction
Edward Zillioux and James Newman, EBI Foundation, Inc., Ft. Pierce, Florida, USA
The cover design for the new international journal, Environmental Bioindicators (EBI), has been a joint effort of the staff of our publisher, Taylor and Francis, and the EBI editors, as well as several others we have gone to for their suggestions and criticisms on layout, color, and illustrations. We particularly would like to thank Mary Drabot, Andrew Moyer, and Kimberly Shigo of Taylor & Francis, Ivy Zillioux and Denis Newman for their artistic critiques, and the authors of the short species accounts that follow.
The background photograph was selected to depict a landscape with a variety of aquatic and terrestrial habitats where bioindicators might be applied to assess environmental health. Along the left border of the background photograph are a series of illustrations representing examples of a hierarchy of animal and plant bioindicators/biomarkers ranging from genetic/molecular to ecosystem/landscape that will be the subjects of research to be published in the EBI issues to come. Superimposed over the background and example indicators is a schematic globe that is meant to represent the worldwide importance of the use of bioindicators in environmental and contamination assessments of natural and human environments. Although many other examples could have been chosen, we believe that the specific illustrations selected are exceptionally good examples of indicators that have been applied successfully in many cases where an assessment of environmental health, integrating biological exposure and response with physical and chemical stessors, has been needed. We have invited experts who have been active in bioindication research with these selected bioindicators to contribute brief accounts of their use and value in this context. The following seven accounts are the result.
We would like to acknowledge and thank Daniel Poleschook, Jr. and Ginger Gumm for the photo of the Common Loon; Duane Raver, Jr. for the drawing of the largemouth bass; Tanja Mrak for the lichen photo; Jaroslav Bohác for the beetle drawing; the coral reef photo can be found at http://www.reefrelief.org/.
Abstract
1 Use of the Nucleic Acid Molecule as a Biomarker in Environmental Research
Patrick Larkin, EcoArray Inc., Alachua, Florida, USA
Since the discovery of the structure of deoxyribonucleic acid (DNA) in 1953 by James Watson and Francis Crick (Watson and Crick 1953) to the recent sequencing of the human genome in the early part of the 21st century (IHGS Consortium 2001; Venter et al., 2001), there have been tremendous advances in how genetic material is being used as a biomarker to measure the health of an ecosystem. The rationale for using genetic material as a biomarker to assess an ecosystem is based on the premise that changes in the normal physiology of an animal due to exposure of a compound or compounds are often first caused by changes at the genetic level. This brief introduction highlights some of the various uses of the nucleic acid molecule (DNA and mRNA) in the context of biomarkers.
The availability of sequence information for an organism can serve as an important benchmark in discussing how the nucleic acid molecule has been used in the past, how it is currently being used today, and how it will be used in the future as a biomarker to measure the health of an ecosystem (see ). For a number of years there was relatively little sequence information available for any one particular organism. Because of this, researchers had to rely on two different approaches for using DNA or mRNA as a biomarker to measure the health of an ecosystem. One approach, which is cellular based, involves examining the morphology of an animal's chromosomes following exposure of the animal to a test compound, either in the laboratory or in the field. These assays include measuring various attributes of the DNA molecule including strand breakage (comet assays), formation of DNA adducts, and alterations in chromosomal number. For a review of these approaches see Lyne et al. 1992; Dixon et al. 2002; and Speit and Henderson 2005.
Figure 1. Various approaches for using genetic material (DNA and mRNA) as a biomarker. mRNA = messenger ribonucleic acid.
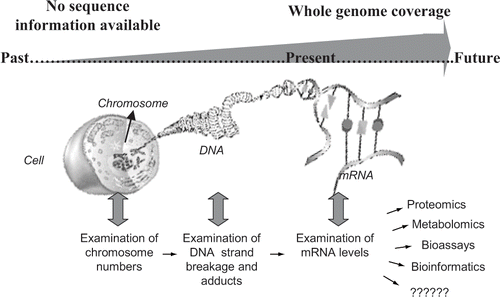
The second approach, which is molecular based, is a gene biomarker discovery method and involves identifying gene transcripts (mRNA sequences) that are differentially regulated following exposure of animals to compounds in the laboratory or the field. Once identified and properly validated, these mRNA sequences can be used as biomarkers to assess the health and recovery of an ecosystem following exposure to a compound or compounds. There are several different procedures that are commonly used to identify these genetic biomarkers including differential display analysis, subtraction libraries, and Serial Analysis of Gene Expression (for review see Denslow et al. in press).
Today, there is a massive amount of sequence data in public repositories like the National Center for Biotechnology Information (NCBI). The NCBI recently reached a major milestone of having 100 gigabases of sequence data deposited from 165,000 different organisms (Mehnert and Cravedi 2004). As mentioned on the NCBI website, this is roughly equivalent to the number of nerve cells in the human brain or the number of stars in the Milky Way. There is now enough sequence data available in a wide range of organisms for the use of more sophisticated tools to identify and use genetic biomarkers for environmental research. One of these tools, called microarrays, are made by spotting or synthesizing thousands of genes specific to an organism onto a solid support matrix. With this technology scientists can now broadly examine different mRNA sequences within an animal, thus enabling rapid identification of biomarkers for a range of compounds. These tools are widely used in toxicity studies, chemical screening, and field evaluation. For a review, see the Denslow and Larkin article in this issue.
In the short span of a little over 50 years, there has been a shift in the scale of how the nucleic acid molecule is being used for biomarker research in the ecotoxicology field: from morphological examination of an animal's chromosomes to a more comprehensive molecular based examination of mRNA sequences that are expressed within an animal using microarray technology.
With the more widespread use of these new tools to measure genetic material also comes several challenges. For example, while there are a lot of sequences available for many organisms, these data are oftentimes not well annotated and characterized. In addition, one should be careful not to over extrapolate and interpret genetic biomarker data in regards to assessing the health of an ecosystem. While correlations can be made between genetic biomarker data and various endpoints like Darwinian fitness traits, population effects, and ecosystem structure and function, these endpoints are oftentimes linked in an oversimplified manner without taking into consideration other factors that can influence either endpoint. In addition, more experiments need to be conducted to better define the changes in the gene expression with changes in other cellular components like proteins and metabolites. Nonetheless, genetic biomarkers will continue to be used in environmental research, both in the laboratory and in the field.
References
Denslow ND, Knoebl I, Larkin P. In press. Approaches in proteomics and genomics for ecotoxicology. In: Biochemistry and molecular biology of fishes-environmental toxicology.
Dixon DR, Pruski AM, Dixon LR, Jha AN. 2002. Marine invertebrate eco-genotoxicology: a methodological overview. Mutagenesis 17:495–507.
International Human Genome Sequencing Consortium. 2001. Initial sequencing and analysis of the human genome. Nature 409:860–921.
Lyne TB, Bickham JW, Lamb T, Gibbons JW. 1992. The application of bioassays in risk assessment of environmental pollution. Risk Anal. 12:361–365.
Mehnert R, Cravedi K. 2004. Public collections of DNA and RNA sequence reach 100 gigabases, 2 pp. National Library of Medicine (available at: <http://www.ncbi.nlm.nih.gov>).
Speit G, Henderson L. 2005. Review of the in vivo genotoxicity tests performed with styrene. Mutat Res. 589:67–79.
Venter JC, et al. 2001. The sequence of the human genome. Science 291:1304–1351.
Watson JD, Crick FH. 1953. Molecular structure of nucleic acids; a structure for deoxyribose nucleic acid. Nature 171:737–738.
Abstract
2. Use of lichens as bioindicators, represented by Pseudevernia furfuracea (L.) Zopf
Tanja Mrak, Jožef Stefan Institute, Ljubljana, Slovenia
Due to their unique properties, lichens have been used as bioindicators for many years and in many applications. These properties include delicate symbiotic associations between lichen partners, lack of protective tissues and stomata, perrenial nature, slow growth, absence of roots, and ability to metabolize at low temperatures during winter. These result in high sensitivity to environmental changes, uptake and accumulation of many substances from the environment (e.g., heavy metals, fluorides, sulphur, nitrogen), and the ability to reveal long-term patterns of some pollutants. In addition, the low cost of monitoring with lichens adds to their usefulness and wide use for this purpose.
Mapping of all the species present in a specific area can be very effective in monitoring ecological response to stressors, since lichen diversity is an excellent indicator of pollution by phytotoxic gaseous substances, and as lichens are also sensitive to other types of environmental alteration, including eutrophication. Lichens also have been used to estimate ecological continuity of forests (i.e., to identify very old, undisturbed forest sites for determining conservation value) and to monitor climate change. Another example is the sampling of chosen lichen species and measurement of pollutants (e.g., heavy metals, radionuclides) that accumulate in the thallus, or by transplanting lichens from an uncontaminated area to a contaminated one, then measuring physiological changes (e.g., cell membrane permeability, chlorophyll integrity, ethylene production, photosynthesis), and/or evaluating the bioaccumulation of pollutants (Asta et al. 2002; Conti and Ceccheti 2001; Garty 2002).
Pseudevernia furfuracea (see cover illustration) is the only one of five mainly North American Pseudevernia species that occurs in Europe. It is characterized by a dorsiventrally built foliose-fruticose thallus (up to 10 cm in diameter) with a grey upper surface, which can be completely covered by numerous isidia, and a channelled lower surface with a single point of attachment. The lobes are strap shaped, 1–4 mm in width and dichotomously branched in one plane (Purvis et al. 1992; Wirth 1995).
P. furfuracea occurs on acidic bark of conifers and deciduous trees, on trunks and especially on twigs, on solitary trees and in forests, on wood, and in areas with high precipitation also on siliceous rocks (Purvis et al. 1992; Wirth 1995). It is a characteristic species of the Pseudevernietum community, favoured by the low bark pH of spruces, pines, and birches in the boreal coniferous zone, where P. furfuracea occurs together with species of Alectoria, Bryoria, Evernia, Hypogymnia, Parmelia (e.g. Parmelia saxatilis) and Usnea (Hawksworth and Hill 1984). This community is also found at higher altitudes in the rest of Europe. P. furfuracea avoids stronger eutrophication but tolerates moderate acidification, in the latter case migrating onto originally alkaline substrates (Wirth 1995).
In 1970, a qualitative scale for estimating sulphur dioxide air pollution in England and Wales was presented where P. furfuracea was grouped together with lichens typically showing concentrations of SO2 of about 50 µg/m3 (Hawksworth and Rose 1970). It was successfully used in explanting/transplanting experiments where lichen thalli collected in a clean environment were packed in bags and exposed for a certain period of time (maximally one year) to estimate atmospheric trace metal pollution (Bari et al. 2001; Giordano et al. 2005) and atmospheric depositions of sulphur, nitrogen, and carbon (Vigniani et al. 2004). Its application in these kinds of studies is favoured by (a) easy collection and cleaning of the thalli due to the thallus growth type, (b) frequent occurrence in the Pseudevernietum community, which allows collection of the quantities required for transplanting experiments, (c) moderate sensitivity to air pollution, (d) its ability to grow also in artificially acidified conditions, and (e) the large surface that comes into contact with air.
In a study of mapping ammonia pollution with epiphytic lichens (focusing on nitrophytes and acidophytes) in the Netherlands, P. furfuracea was one of the characteristic species of acidophytes observed to disappear in the presence of ammonia and ammonium (explained by the rise in pH and ammonium content of bark) (van Herk 1999).
References
Asta J, Erhardt W, Ferretti M, Fornasier F, Kirschbaum U, Nimis PL, Purvis OW, Pirintsos S, Scheidegger C, Van Haluwyn C, Wirth V. 2002. European guideline for mapping lichen diversity as an indicator of environmental stress. Available from: http://www.thebls.org.uk/eumap.pdf
Bari A, Rosso A, Minciardi MR, Troiani F, Piervittori R. 2001. Analysis of heavy metals in atmospheric particulates in relation to their bioaccumulation in explanted Pseudevernia furfuracea thalli. Environmental Monitoring and Assessment 69:205–220.
Conti ME, Ceccheti G. 2001. Biological monitoring: lichens as bioindicators of air pollution assessment—a review. Environmental Pollution 114: 471–492.
Garty J. 2002. Biomonitoring heavy metal pollution with lichens. In: Kranner I, Beckett RP, Varma AK, editors. Protocols in Lichenology. Culturing, Biochemistry, Ecophysiology and Use in Biomonitoring. Berlin, Heidelberg: Springer-Verlag. pp. 458–482.
Giordano S, Adamo P, Sorbo S, Vigniani S. 2005. Atmospheric trace metal pollution in the Naples urban area based on results from moss and lichen bags. Environmental Pollution 136:431–442.
Hawksworth DL, Hill DJ. 1984. The Lichen-Forming Fungi. Glasgow, Blackie & Son.
Hawksworth DL, Rose F. 1970. Qualitative scale for estimating sulphur dioxide air pollution in England and Wales using epiphytic lichens. Nature 227:145–148.
van Herk CM. 1999. Mapping of ammonia pollution with epiphytic lichens in the Netherlands. The Lichenologist 31:9–20.
Purvis OW, Coppins BJ, Hawksworth DL, James PW, Moore DM. 1992. The Lichen Flora of Great Britain and Ireland. London: British Lichen Society.
Vigniani S, Adamo P, Giordano S. 2004. Sulphur, nitrogen and carbon content of Sphagnum capillifolium and Pseudevernia furfuracea exposed in bags in the Naples urban area. Environmental Pollution 129:145–158.
Wirth V. 1995. Die Flechten Baden-Württembergs. Teil 1 und 2. Stuttgart: Ulmer.
Abstract
3. Carabids as a model bioindicator for landscape disturbance and management
Jaroslav Boháč, University of South Bohemia, České Budějovice, Czech Republic
Carabids are a family of insects that are sensitive to a number of environmental factors including global climate changes, landscape structure change, deforestation, unsuitable management in landscape, fragmentation of landscape, urbanization, overdrainage and the consequential desiccation of landscape, as well as unsuitable fertilizers and pesticide application. They demonstrate a flexible set of responses to these abiotic and biotic factors through changes in numbers, species composition, morphology, and chemical body burdens. For example, the increased frequency or absence of rare species or the species composition for natural or semi-natural habitats in community can indicate the high or low quality of biodiversity of the studied ecosystem (Boháč 2003).
As bioindicators, carabids have the added advantage of being better known taxonomically. In addition, most carabids are omnivorous feeders, mostly predators. The majority of Carabids are nocturnal and live at the surface of the ground. Some species live in the soil or in caves.
In general, ground-beetles show good dispersal capabilities and, as a result, are found in a variety of landscapes. Carabids are relatively easy to sample quantitatively, especially using pitfall traps.
The ecology of these Holarctic species is relatively well known (e.g., Lindroth 1985, 1986; Koch 1989; Larochelle and Lariviere 2003). Ground-beetles are commonly used to:
• | assess the biodiversity value of ecosystems (e.g., Sharova 1981, Bohác and Fuchs 1991, Desender, Dufrene, Loreau, Luff, and Maelfait 1994, Turin, Penev and Casale 2003); | ||||
• | indicate the impact of landscape changes (e.g., Ružicka and Bohác, 1990, Bohác and Fuchs 1991; Meissner and Janusch 1996; Luff 2002); | ||||
• | evaluate the level of ecosystem impact by man, predict the effect of climate changes, classify habitats for nature protection (e.g., Desender, Dufrene, Loreau, Luff, and Maelfait 1994; Turin, Penev, and Casale 2003); and | ||||
• | characterize soil-nutrient status in forestry (e.g., Lovei and Sunderland 1996; Brandmayr et al. 2000; Holland 2002). |
Carabids are the key group of organisms in ecosystems and their occurrence in the landscape or ecosystem can indicate the value of a landscape's or ecosystem's biodiversity (see Bohác and Fuchs 1991) For these reasons the interpretation of results from the field studies is relatively easy compared to other groups of insects where less is known about their ecology.
Carabus granulatus (L.) is the typical representative of ground beetles distributed in Europe and North Asia. They are an introduced species to some parts of North America. This species is widely distributed within the agricultural landscape in Europe (Holland 2002). It has been used for testing of pesticides as one of the model species of invertebrates. Since the species lives mainly in corridors and forest margins it is sensitive to changes in landscape structure. The species is also sensitive to other anthropogenic changes in habitat quality (desiccation, chemical pollution, etc.). C. granulatus is negatively affected by deep ploughing and enhanced by reduced tillage systems. No negative effects have been found for mechanical weed control and flaming. The species is positively affected by proper organic fertilization and green manuring, however, intensive nitrogen amendment might affect this species by altering crop density and microclimate (Kromp 1999; Holland 2002).
Because of the usefulness of Carabids as bioindicators, C. granulatus was selected to represent this group of terrestrial invertebrate bioindicators of ecosystem or landscape disturbance and mismanagement.
References
Bohác J. 2003. The effect of environmental factors on communities of carabid and staphylinid beetles (Coleoptera: Carabidae, Staphylinidae). In: Frouz J, ourková M, Frouzová J, editors. Soil physical properties and their interactions with soil organisms and roots of plants. Ceské Budejovice: Institute of soil biology AS CR. pp. 113–118.
Bohác J, Fuchs R. 1991. The structure of animal communities as bioindicators of landscape deteriorisation, In: Jeffrey D and Madden B, editors. Bioindicators and Environmental Management. San Diego: Academic Press. pp. 165–178.
Brandmayr P, Lovei GL, Brandmayr Z, Casale A, Vigna Taglianti A. 2000. Natural History and Applied Ecology of Carabid Beetles. Series Faunistica No. 19. Sofia, Moscow: Pensoft.
Desender K, Dufrene M, Loreau M, Luff MJ, Maelfait JP. 1994. Carabid beetles: Ecology and evolution. Dordrecht, Boston, London: Kluwer Academic Publishers.
Holland JM. 2002. The Agroecology of Carabid Beetles. Andover: Intercept.
Koch K. 1989. Die Kafer Mitteleuropas. Okologie. Band 1. Krefeld: Goecke & Evers.
Kromp B. 1999. Carabid beetles in sustainable agriculture: a review on pest control efficacy, cultivation impacts and enhancement. Agriculture, Ecosystems and Environment 74:187–228.
Larochelle A, Lariviere MC. 2003. A Natural History of the Ground Beetles (Coleoptera: Carabidae) of America North of Mexico. Sofia, Moscow: Pensoft Publishers.
Lindroth CH. 1985, 1986. The Carabidae (Coleoptera) of Fennoskandia and Denmark. Fauna Entomologica Scandinavica. Volume 15, Part 1 (1985):1–228, Part 2 (1986):229–497.
Lovei GL, Sunderland KD. 1996. Ecology and behaviour of ground beetles (Coleoptera, Carabidae). Annual Review of Entomology 41:231–256.
Luff ML. 2002. Carabid assemblages organization and species composition. In: Holland JM, editors. The Agroecology of Carabid Beetles. Andover, Hampshire: Intercept Ltd. pp. 41–79.
Meissner A, Janusch G. 1996. Untersuchung und Bewertung der Korrelationen von Arthropodengesellschaften mit Makro- und Mikrostrukturen in Verschiedenen Landschaftselementen. TU Berlin: Institut fur Biologie.
Ružicka V, Bohác J. 1990. The utilization of epigeic invertebrate communities as bioindicators of terrestrial environmental quality. In: Salanki J, Jeffrey D and Hughes GM, editors. Biological Monitoring of the Environment, a Manual of Methods. Walingford: CAB International. pp. 79–86.
Sharova IK. 1981. The life forms of Carabids. Moscow: Nauka.
Turin H, Penev L, Casale A. 2003. The genus Carabus in Europe. A synthesis. Fauna Europaea Evertebrata No 2. Sofia-Moscow: Pensoft.
Abstract
4. Coral reefs as indicators of long-term ecosystem change at the regional and global scales
Paul Sammarco, Louisiana Universities Marine Consortium, Chauvin, LA, USA and Jennifer Wheaton, Florida Marine Research Institute, St. Petersburg, Florida, USA
Coral reefs are the only geological structures in the world built entirely by living organisms. They are confined to the tropics and sub-tropics and have changed in character and geographic range repeatedly through geological time. The hermatypic corals which are responsible for secreting much of the calcium carbonate that defines their growth have evolved in a stenohaline, oligotrophic marine environment and within a highly restricted temperature regime.
Human activities have caused changes in many environmental factors which in turn, affected corals and their associated fauna and flora. Resulting impacts are characterized by a loss of coral cover over large areas, changes in dominant groups from corals to algae, loss of reef fish, increased bioerosion of reef substratum, etc. It is therefore important to identify factors leading to the demise of corals and related organisms, and also the manner in which these factors interact in causing mortality or disease and eventually changes in community structure. It is also important to identify suitable bioindicators (environmental, physiological, or molecular), or physical or chemical environmental indicators, that may describe or predict such changes. The common brain coral, Diploria strigosa depicted on the cover of this journal, has been the subject of many studies regarding environmental effects on corals and reefs, including effects of increased sea surface temperatures (SSTs), increased ammonium concentrations, disease, bleaching, hurricanes, oil spills, sedimentation, etc.
The critical need for the acquisition of scientific information in this area is underscored by recent studies performed in the NOAA Florida Keys National Marine Sanctuary. There, a decline in coral species diversity and cover has been observed over the past two decades. For example, between 1984 and 1991, coral cover has been reduced by 43.9% (Porter et al. 1993) and 60% (Porter et al. 2001) at certain sites along the reef tract. Between 1997 and 1999, coral cover declined further at some sites from 11.4% to 7.4%. By 2004, mean coral cover was reduced to 6.6%.
There is a general consensus that multiple stressors acting at local, regional, and global scales are responsible for negative impacts on coral reefs around the world, including the Florida Keys. The U.S. Coral Reef Task Force (http://coralreef.gov/threats.cfm) considers the following anthropogenic activities to be major threats to corals worldwide: human population increases; shoreline development; trampling by tourists and divers; ship groundings; pollution via sewerage discharge and agricultural runoff; sedimentation due to deforestation and river runoff; overfishing; use of destructive fishing practices such as poisons, explosives, and fish-pots that destroy coral habitat and deplete fish stocks indiscriminately with respect to species, trophic level, and at all stages of development; etc. (see Sammarco 1996; McClanahan et al. in press). Accumulating evidence indicates that increased SSTs due to increased concentrations of greenhouse gases in the atmosphere (e.g., Mortsch and Quinn 1996; Beardall and Raven 2004; Oreskes 2004) are causing deleterious effects on the stability of coral reef ecosystems by inducing mass bleaching on a large scale throughout the geographic range of coral reefs around the world (Hoegh-Guldberg 1999; Souter and Linden 2000; Glynn et al. 2001; Wilkinson 2002; Licuanan and Gomez 2002; Aeby et al. 2003; McClanahan et al. in press). These stressors are inter-twined and aggravated by other natural perturbations such as hurricanes and disease that cause further reef degradation. For example, elevated SSTs in 1997 and 1998 caused increased stress, bleaching, and mortality in corals in the Florida Keys (Edmunds et al. 2003), followed by physical damage to the reefs and coral populations from Hurricane Georges in 1998 (see Rogers and Miller 2001). A similar series of events happened to Feather Reef, Great Barrier Reef, Australia in the mid-1980s when the eye of a strong cyclone passed directly over the reef two years after coral cover was decimated by a Crown-of-Thorns starfish (Acanthaster planci) population explosion (PWS, pers. obs.). Coral cover has also declined on reefs of the western Caribbean and the southern Gulf of Mexico in recent years. Hurricanes Mitch (1998), Keith (2000), Iris (2001), and Isidore (2002) had major impacts on reefs along Belize, Honduras, and the Mexican Yucatan. Almanda-Villela et al (2002) reported that coral cover on some reefs in Belize declined by 75%. The most recent effects of Hurricanes Katrina, Rita, and Wilma on reefs in the Florida Keys and Dry Tortugas have yet to be documented.
The major emphasis of coral reef research worldwide at this point in time is to identify the causes of coral decline, assess the synergistic impact of these causes on global, regional, and local scales, and identify active means by which to mitigate and hopefully reverse this degradation.
References
Aeby GS, Kenyon JC, Maragos JE, Potts DC. 2003. First record of mass coral bleaching in the northwestern Hawaiian Islands. Coral Reefs 22:256.
Almanda-Villela P, McField M, Kramer P, Kramer PR, Arias-Gonzalez E. 2002. Status of coral reefs of Mesoamerica- Mexico, Belize, Guatemala, Honduras, Nicaragua and El Salvador. In: Wilkinson C, editor. Status of the coral reefs of the world: 2002. Global Coral Reef Monitoring Network, Australian Institute of Marine. pp. 303–323.
Beardal J, Raven JA. 2004. The potential effects of global climate change on microalgal photosynthesis, growth, and ecology. Phycologia 43:26–50.
Edmunds PJ, Gates RD, Gleason DF. 2003. The tissue composition of Montastraea franksi during a natural bleaching event in the Florida Keys. Coral Reefs 22:54–62.
Glynn PL, Mate JL, Baker AC, Calderon MO. 2001. Coral bleaching and mortality in Panama and Ecuador during the 1997–1998 El Nino–Southern Oscillation event: Spatial/temporal patterns and comparisons with the 1982–1983 event. Bull. Mar. Sci. 69:79–109.
Hoegh-Guldberg O. 1999. Climate change, coral bleaching, and the future of the world's coral reefs. Mar. Freshwat. Res. 50:839–866.
Licuanan WY, Gomez ED. 2002. Philippine coral reefs: Status and the role of the academe to improve their management. Proc. 9th Int. Coral Reef Symp., Bali, 2000, Vol. 2, 835–840.
McClanahan T, Hoegh-Guldberg O, Buddemeier RW, Sammarco PW. (in press). Trajectory and predictions for coral reefs. In: Polunin N, editor. Proc. 5th Int. Conference on the Environment Future (ICEF), Zurich, Switzerland. EAWAG.
Mortsch LD, Quinn FH. 1996. Climate change scenarios for Great Lakes Basin ecosystem studies. Limnol. Oceanogr. 41:903–911.
Oreskes N. 2004. The scientific consensus on climate change. Science 306:1686.
Porter JW, Meier OW, Walton Smith FG. 1993. Case Histories for the colloquium and forum. Global aspects of coral reefs: Health hazards and history. Miami, FL: University of Maimi.
Porter JW, Dustan P, Jaap WC, Patterson KL, Kosmynin V, Meier OW, Patterson ME, Parsons M. 2001. Hydrobiologia 460:1–24.
Rogers CS, Miller J. 2001. Coral bleaching, hurricane damage, and benthic cover on coral reefs in St. John, U.S. Virgin Islands: A comparison of surveys with the chain transect method and videography. Bull. Mar. Sci. 69:459–470.
Sammarco PW. 1996. Comments on coral reef regeneration, bioerosion, biogeography, and chemical ecology: Future directions. J. Exp. Mar. Biol. Ecol. 200:135–168.
Souter DW, Linden O. 2000. The health and future of coral reef systems. Ocean Coast. Manage. 43:657–688.
Wilkinson C, editor. 2002. Status of coral reefs of the world: 2002. Townsville, Qld., Australia: Australian Institute of Marine Science.
Abstract
5. Largemouth bass as Bioindicators of Mercury Bioaccumulation in Florida
Ted Lange, Florida Fish and Wildlife Conservation Commission, Eustis, Florida, USA
Of the seven species of black bass, the largemouth bass (Micropterus salmoides) has the widest geographical distribution: the species' range extends from Canada to South America (Lee et al. 1980). Due to their widespread distribution, fast growth rates, and ability to reach trophy size, largemouth bass have become the principal warmwater predatory sport fish in most parts of the United States, and in Florida they play an important ecological role as a top predator in most water bodies. This species is also important recreationally and economically in Florida, generating 12.2 million days of angling in 2001 (DOI 2002).
Their ubiquitous distribution, popularity as a sport fish, scientific importance, and propensity to bioaccumulate mercury have made largemouth bass a potentially good indicator of spatial and temporal trends in mercury bioaccumulation. Mercury levels in largemouth bass have been used extensively in risk assessments, trend monitoring, and predictive modeling in Florida over the past 20 years.
In 1982, fisheries scientists investigating a suite of inorganic and organic contaminants in fish tissue from fish downstream of a derelict battery salvage plant on the Chipola River found only moderate mercury levels in largemouth bass tissue (Ware et al. 1990). Conversely, extremely elevated levels of mercury were observed from a reference site in the pristine Sante Fe River, demonstrating mercury's ability to contaminate seemingly pristine waterbodies. Subsequent surveys have revealed toxicologically significant levels of mercury in largemouth bass throughout Florida. Fish-consumption advisories for largemouth bass have been established throughout Florida, urging limited or in some cases no consumption of largemouth bass from lakes, rivers, and marshes (FLDOH 2005).
To further evaluate spatial and temporal distributions of mercury in largemouth bass, studies were initiated to examine factors influencing variability in mercury bioaccumulation in Florida lakes, rivers, and marshes. The age-standardized mercury concentration in bass (EHg3) has provided a useful tool for these purposes (Lange et al. 1993).
Largemouth bass serve as a primary tool for monitoring the effects of restoration projects on mercury bioaccumulation in the Everglades. Restoration projects, required by the Everglades Forever Act, require implementation of best-management practices for agriculture and creation of man-made wetlands to reduce phosphorus concentrations in surface water entering the Everglades. Projects are also being implemented to modify water movements to more closely mimic historical hydrologic regimes. Fifteen years of long-term monitoring of EHg3 at 10 sites in the Everglades have provided an extensive record of mercury bioaccumulation in this important ecosystem during implementation of these projects. Recent declines in EHg3 have been evident in the water conservation areas of the northern Everglades (for example, see ); however, restoration-induced modifications to water quality and quantity may be causing increases in largemouth bass mercury bioaccumulation farther south in Everglades National Park (SFWMD 2005).
Figure 2. Age-3 standardized total mercury concentration in largemouth bass muscle tissue (EHg3) collected in the L-67A Canal in Water Conservation Area 3 of the Florida Everglades between 1990 and 2004.
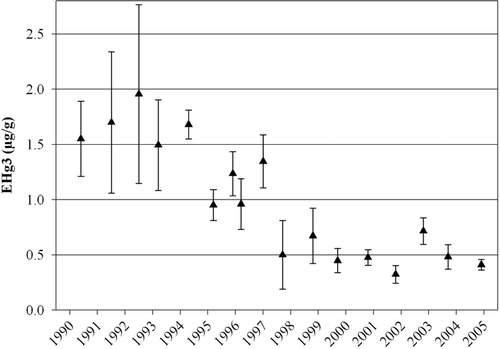
Studies characterizing environmental parameters that influence mercury bioaccumulation have been conducted in Florida lakes and rivers by using monitoring and synoptic surveys. Early studies in Florida lakes revealed that mercury bioaccumulation in largemouth bass varied widely, with pH accounting for 41% of the variation in EHg3 in a study of 53 diverse lakes (Lange et al. 1993). Current studies demonstrate strong relationships between EHg3 and measures of lake ionic strength, productivity, and wetlands influence in lakes and with river stage in the Suwannee River.
The descriptive approaches used in these studies in which largemouth bass are considered to be indicators of mercury bioaccumulation not only provide data on the distribution of mercury in aquatic systems for risk assessment but also provide a basis for evaluating local, regional, and national trends in mercury pollution as well as insight into options that may act to ameliorate the mercury problem.
References
Florida Department of Health (FLDOH). 2005. Florida fish consumption advisories (Internet). May 2005. Available from: http://www.doh.state.fl.us/floridafishadvice.
Lange TR, Royals HE, Connor LL. 1993. Influence of water chemistry on mercury concentration in largemouth bass from Florida lakes. Trans. Am. Fish. Soc. 122:74–84.
Lee DS, Gilbert CR, Hocutt CH, Jenkins RE, McAllister DE, Stauffer JR, Jr. 1980. Atlas of North American freshwater fishes. Raleigh, NC: North Carolina Biological Survey.
South Florida Water Management District (SFWMD). 2005 South Florida environmental report, Chapter 2B: mercury monitoring, research and environmental assessment in south Florida. West Palm Beach, FL: South Florida Water Management District.
US Department of the Interior (DOI). 2002 National survey of fishing, hunting, and wildlife associated recreation, state overview. Washington, DC: US Department of the Interior.
Ware FJ, Royals H, Lange T. 1990. Mercury contamination in Florida largemouth bass. Proc Annu Conf Southeast Assoc FishWildl Agencies 44:5–12.
Abstract
6. Raccoons as Bioindicators
Joanna Burger, Rutgers University, Piscataway, New Jersey, USA
Evaluating ecosystem health requires the use of bioindicators of exposure and effects. To be most useful, a bioindicator must be developed in such a way that it is not only useful to reflect current biological status, but can be used to examine long-term trends. Indicators selected should (1) be biologically, methodologically and societally relevant, (2) exhibit changes in response to a stressor, but not be so sensitive that changes occur when there is no cause for concern (no lasting reproductive, survival, or population effects), (3) be sufficiently common and widespread as to have general use, and (4) reflect population, community, or ecosystem effects (Suter 1990; EPA 1997; Burger and Gochfeld 2004).
Raccoons (Procyon lotor) are ideal bioindicators because they are widespread and abundant in the United States, occupy both rural and urban environments, have been used extensively for monitoring contaminant levels, and are of interest to the general public. They are an omnivore and predator that eats a complex range of plants and animals, and in turn are eaten by many different animals (Burger 1999), including people (Burger et al. 1999; Gaines et al. 2000). Further, they are terrestrial animals that eat prey both on land and from the water. Males can travel a few km in their daily movements, making them useful for monitoring off-site movement of pollutants from contaminated sites, such as Superfund sites, or those owned by the Department of Defense or Department of Energy (Gaines et al. 2000; Burger et al. 2000, 2002).
Raccoons can be used as bioindicators for different levels of biological organization, and for different kinds of stressors, including chemical (e.g., contaminants), physical (e.g., habitat disruption), and biological (e.g., rabies and other diseases). Understanding contaminant levels in their tissues can provide information on the health of raccoon populations themselves, on their predators or scavengers, on other organisms that occupy the same trophic level, and on other parts of the ecosystem, including the ultimate decomposition of their carcasses. Other stressors, such as habitat changes, can lead to population changes, with associated changes on other organisms within their food web. As with any bioindicator, the accumulation of long-term data on raccoons makes them even more useful for comparative purposes, across temporal and spatial scales. Because hair can be analyzed for some contaminants, such as metals, museum collections can provide reference samples for temporal analyses (Porcella et al. 2004).
Raccoons have been used extensively to monitor both fate and effects of contaminants, primarily metals such as mercury (Burger et al. 2002; Porcella et al. 2004), although organochlorines (Herbert and Peterle 1990) and radionuclides (Gaines et al. 2000) have also been measured. Metal levels have been measured directly in internal tissues (Burger et al. 2002) and in hair as an indicator of internal exposure (Lord et al. 2002), as well as by using biomarkers of exposure, such as metalothionein (Burger et al. 2000). Hair mercury levels correlate significantly with other tissues, although the correlations are not always high (Cumbie 1975; Lord et al. 2002). This may relate to both the time of sampling, and whether sampling occurred during the greatest biouptake period; this relationship requires further study. The difficulties of interpreting metal levels in raccoons because of their omnivorous diet can be ameliorated with the use of stable isotopes to identify the trophic level of particular individuals (Gaines et al. 2002).
One of the advantages of raccoons is that movement data and contaminants data can be combined in a GIS format to provide information on exposure pathways and risk to other ecoreceptors (Gaines et al. 2005). Increasingly, it will be essential to be able to monitor and map animal populations in a dynamic matrix with chemicals and other stressors. Raccoons provide that opportunity.
References
Burger J. 1999. Animals in towns and cities. Dubuque, IA: Kendall-Hunt Publ. Co.
Burger J, Sanchez J, Gibbons JW, Benson T, Ondrof J, Ramos R, McMahon MJ, Gaines K, Lord C, Fulmer M, Gochfeld M. 1999. Attitudes and perceptions about ecological resources and hazards of people living around the Savannah River site. Environ. Monit. Assess. 57:195–211.
Burger J, Gochfeld M. 2004. Bioindicators for assessing human and ecological health. In: Wiersma GB, editor. Environmental Monitoring Boca Raton, FL: CRC Press.
Burger J, Lord CG, McGrath L, Gaines KF, Brisbin IL Jr., Gochfeld M, Yurkow EJ. 2000. Metals and metallothionein in the liver of raccoons: utility for environmental assessment and monitoring. J. Toxicol. Environ. Health 60:243–261.
Burger J, Gaines KF, Lord C, Shukla C, Gochfeld M. 2002. Metal levels in raccoon tissues: differences on and off the Department of Energy's Savannah River Site in South Carolina. Environ. Monit. Assess. 74:67–84.
Cumbie PM. 1975. Mercury in hair of bobcats and raccoons. J. Wildl. Manage. 39:419–425.
Environmental Protection Agency (EPA). 1997. Ecological indicators: evaluation criteria. Washington, D.C: Environmental Protection Agency.
Gaines KF, Lord CG, Brisbin Jr. IL, Boring CS, Gochfeld M, Burger J. 2000. Radiocesium in Raccoons: population differences and potential human risks. J. Wildl. Manage. 64:199–208.
Gaines KF, Romanek CS, Boring CS, Lord CG, Gochfeld M, Burger J. 2002. Using raccoons as an indicator species for metal accumulation across trophic levels: a stable isotope approach. J. Wildl. Manage. 66:811–821.
Gaines KR, Boring CS, Porter DW. 2005. The development of a spatially explicit model to estimate radiocesium body burdens in raccoons (Procyon lotor) for ecological risk assessment. Sci. Total Environ. 34:15–31.
Herbert GB, Peterle TJ. 1990. Heavy metal and organochlorine compound concentrations in tissues of raccoons from East-Central Michigan. Bull. Environ. Contam. Toxicol. 44:331–338.
Lord CG, Gaines KF, Boring CS, Brisbin IL Jr., Gochfeld M, Burger J. 2002. Raccoon (Procyon lotor) as a bioindicator of mercury contamination at the U.S. Department of Energy's Savannah River Site. Arch. Environ. Contam. Toxicol. 43:356–363.
Porcella DB, Zillioux EJ, Grieb TM, Newman JR, West GB. 2004. Retrospective study of mercury in raccoons (Procyon lotor) in south Florida. Ecotoxicol. 13:207–221.
Suter GW II. 1990. Endpoints for regional ecological risk assessment. Environ. Manage. 14:9–23.
Abstract
7. Loons as biosentinels of aquatic integrity
David C. Evers, BioDiversity Research Institute, Gorham, Maine, USA
Loons, in particular the Common Loon (Gavia immer — see cover illustration), are appropriate indicators of acute and chronic stressors that disrupt aquatic integrity. As long-lived, upper trophic level species that have low annual fecundity, loons are susceptible to abnormal adult mortality or slight changes in behavior and reproductive success that may be affected by persistent bioaccumulative toxins, water quality degradation, changes in predator-prey dynamics, catastrophic ecological events, and even direct and local recreational impacts.
Persistent bioaccumulative toxicants (PBTs) include well-known synthetic compounds such as polychlorinated biphynels (PCBs), organochlorines including DDT and its derivatives as well as less-described ecotoxicants including flame retardants such as polybrominated diphenyl ether (PBDE). Remobilization of some heavy metals that are PBTs, such as mercury, also can have reverberating disruptions along longer food chains. Investigations of organochlorines in loons defined exposure (Frank et al. 1983; Burgess et al. 2005), but efforts toward understanding mercury toxicity have been the most instrumental for using the loon as an indicator of environmental contamination.
Efforts since the early 1990s have established the common loon as the primary avian indicator for evaluating the exposure and effects of methylmercury availability in freshwater lakes of North America (Evers 2006). The common loon's elevated stature is based on well-defined geographic exposure profiles (Evers et al. 1998, 2003; Scheuhammer 2001), established lowest observed adverse effect levels (Barr 1986; Nocera and Taylor 1998; Burgess et al. 1998; Evers et al. 2003, 2004), growing knowledge of pharmacokinetics (Fournier et al. 2002; Kenow et al. 2003), confounding factors such as prey availability (Merrill et al. 2005), and risk assessment models (Nacci et al. 2005). Instead of individual-based laboratory studies that were formerly used to extrapolate potential avian effects (Heinz 1979), recent risk assessment efforts with the common loon combine laboratory (Fournier et al. 2002; Kenow et al. 2003) and field studies (Evers et al. 2004; Burgess et al. 2005) with developing population models (Nacci et al. 2005; Evers 2006). This body of knowledge is the basis for the common loon to be identified as a principal indicator of methylmercury availability for freshwater piscivores for developing wildlife criterion values (Evers et al. 2004), and within a national mercury monitoring program (Mason et al. 2005).
Degradation of water quality is a common problem across lakes and nearshore marine waters in North America and can take many forms. Airborne and waterborne pollution by PBTs are noted direct problems. However, many other threats are ubiquitous including oil spills and their associated elevated polycyclic aromatic hydrocarbon (PAHs) levels. Wintering loons are particularly vulnerable to coastal oil spills and, because they are conspicuously large birds, are commonly used as indicators (dead or alive) for restoration needs. Sperduto et al. (2003) emphasized a scaling restoration effort that focused on the common loon as a representative of general loss of birds from an oil spill in Rhode Island. Because loon demographic information is well established, scaling calculations were able to quantify the number of loon-years lost and number of nests needed to replace those years.
Predator-prey relationships are dynamic phenomena that evolve over time. However, introduced species can have rapid, cascading effects on bird populations. One such example is botulism type E (Clostridium botulinum) and its influence on the common loon. Loons were important indicators of botulism outbreaks in the upper Great Lakes in the 1960s (Fay 1966) and 1980s (Brand et al. 1988) but it is the outbreaks in the past several years in Lake Erie that are most challenging (Roblee 2002). Two introduced species, the quagga mussel (Dreissena bugensis) and the round goby (Neogobius melanostomus), have provided a long-term pathway for natural levels of botulism to be dramatically bioconcentrated and for high levels of the bacteria to enter the foodweb of upper trophic level species. Over 10,000 migrant loons died from this specific outbreak between 2000 and 2003.
Catastrophic events are generally related to winter dieoffs of loons in marine environments (Alexander 1991; Spitzer 1995), that can exceed 10,000 individuals (Forrester et al. 1997). Reasons for dieoffs are not completely known but have been related to unusual weather events that impact prey availability. For example, in the early and mid 1980s, large numbers of beached and dead loons were connected to a string of cascading ecological events triggered by long stretches of inclement weather. Storm-induced turbidity of coastal waters and cold weather in the Gulf of Mexico may have forced loons to focus their foraging behavior on benthic invertebrates such as crabs (Forrester et al. 1997). Crabs have lower nutritional quality and greater parasite loads, and most likely were responsible for a weakened physiological condition called emaciation syndrome in loons. Loons in an emaciated state may have remobilized methylmercury body burdens, which further reduced the ability to capture prey; therefore, individuals were not able to maintain dietary requirements. These scenarios are particularly important for wintering adult loons that are undergoing remigial molts because they have limited mobility and are under increased physiological demands. Spatiotemporal alterations of marine system dynamics related to changes in climate may therefore be indicated by the health of midwinter adult loons.
Disturbance by human intrusion is a complex impact that is difficult to quantitatively measure because loons can acclimate to some changes. There are at least 14 studies that have documented increasing rates of recreational use and development with decreasing loon productivity and presence while 12 other studies demonstrate the loon's ability to adopt adaptive strategies to offset changes (Evers 2006). However, lead poisoning resulting from the ingestion of lead fishing tackle is a threat that defies habituation (Scheuhammer and Norris 1996; Sidor et al. 2003). Intensive national studies of multiple bird species found that the common loon had the highest incidence of lead ingestion (Franson et al. 2003). In a long-term New England study, Sidor et al. (2003) documented 44% of breeding adults died of lead toxicosis.
The common loon and other loon species are suitable indicators of short- and long-term environmental changes at multiple spatial scales. Loons may indicate changes of direct recreational pressure at the lake level to subtle but far-reaching global changes related to the long-range transfer of atmospheric pollutants. The multiplicity and synergistic mix of stressors at various spatiotemporal scales therefore demands well-defined and carefully chosen indicators. As such, the ecological criteria that make loons good integrating indicators is furthered by their charismatic appeal to the public, which places them in good standing with decision-makers as a suitable basis for policy and regulatory changes.
References
Alexander LL. 1991. Patterns of mortality among Common Loons wintering in the northeastern Gulf of Mexico. Fla. Field Nat. 19:73–79.
Barr JF. 1986. Population dynamics of the Common Loon (Gavia immer) associated with mercury-contaminated waters in northwestern Ontario. Occ. Paper 56, Canadian Wildl. Serv., Ottawa, Ontario.
Brand CJ, Schmitt S, Duncas RM, Cooley TM. 1988. An outbreak of Type E botulism among Common Loons (Gavia immer) in Michigan's Upper Peninsula. J. Wildl. Dis. 24:471–476.
Burgess NM, Evers DC, Kaplan JD, Duggan M, Kerekes JJ. 1998. Mercury and reproductive success of Common Loons breeding in the Maritimes. In: Mercury in Atlantic Canada: A progress report. Sackville, NB: Environ. Canada. pp. 104–109.
Burgess NM, Evers DO, Kaplan JD. 2005. Mercury and other contaminants in common loons breeding in Atlantic Canada. Ecotoxicology 14:193–222.
Evers DC. 2006. Status assessment and conservation plan for the Common Loon (Gavia immer) in North America. U.S. Fish Wildl. Serv., Tech. Rept. Ser. No., Denver, CO.
Evers DC, Taylor KM, Major A, Taylor RJ, Poppenga RH, Scheuhammer AM. 2003. Common Loon eggs as indicators of methylmercury availability in North America. Ecotoxicology 12:69–81.
Evers DC, Lane OP, Savoy L, Goodale W. 2004. Assessing the impacts of methylmercury on piscivorous using a wildlife criterion value based on the Common Loon, 1998-2003. Report BRI 2004–05 submitted to the Maine Dept. Environ. Protection. BioDiversity Res. Inst., Falmouth, ME.
Evers DC, Kaplan JD, Meyer MW, Reaman PS, Braselton WE, Major A, Burgess N, Scheuhammer AM. 1998. Geographic trend in mercury measured in Common Loon feathers and blood. Environ. Tox. Chem. 17:173–183.
Fay LD. 1966. Type E botulism in Great Lakes water birds. Trans. 31st N. Am. Wildl. Nat. Res. Conf. 1:139–149.
Forrester DJ, Davidson WR, Lange Jr RE, Stroud RK, Alexander LL, Franson JC, Haseltine SD, Littell RC, Nesbitt SA. 1997. Winter mortality of Common Loons in Florida coastal waters. J. Wildl. Dis. 33:833–847.
Fournier F, Karasov WH, Kenow KP, Meyer MW, Hines RK. 2002. The oral bioavailability and toxicokinetics of methylmercury in common loon (Gavia immer) chicks. Comp. Biochem. Physiol. Part A 133:703–714.
Frank R, Lunsden H, Barr JF, Braun HE. 1983. Residues of organochlorine insecticides, industrial chemicals, and mercury in eggs and in tissues taken from healthy and emaciated common loons, Ontario, Canada, 1968–1980. Arch. Environ. Contam. Tox. 12:641–654.
Franson JC, Hansen SP, Creekmore TE, Brand CJ, Evers DC, Duerr AE, Destefano S. 2003. Lead fishing weights, other fishing tackle, and ingested spent shot in selected waterbirds. Waterbirds 26:345–352.
Heinz GH. 1979. Methylmercury: reproductive and behavioral effects on three generations of Mallard ducks. J. Wildl. Manage. 43:394–401.
Kenow KP, Gutretuer S, Hines RK, Meyer MW, Fournier F, Karasov WH. 2003. Effects of methyl mercury exposure on the growth of juvenile common loons. Ecotoxicology 12:171–182.
Mason R, Abbot M, Bodaly D, Bullock R, Driscoll C, Evers D, Lindberg S, Murray M, Swain E. 2005. Monitoring the environmental response to changes in mercury contamination from the atmosphere: A multi-media challenge. Environ. Sci. Technol. 39:15A–22A.
Merrill EH, Hartigan JJ, Meyer MW. 2005. Does prey biomass of mercury exposure affect loon chick survival in Wisconsin? J. Wildl. Manage. 69:57–67.
Nacci D, Pelletier M, Lake J, Bennett R, Nichols J, Haebler R, Grear J, Kuhn A, Copeland J, Nicholson M, Walters S, Munns Jr WR. 2005. An approach to predict risks to wildlife populations from mercury and other stressors. Ecotoxicology 14:283–293.
Nocera J, Taylor P. 1998. In situ behavioral response of common loons associated with elevated mercury exposure. Conservation Ecology 2(2):10.
Roblee K. 2002. Botulism caused waterbird mortality in New York waters of Lake Erie — 2001. In: Sea Grant Ohio. Botulism in Lake Erie, workshop proceedings. Meeting on 28 February, 2002 at Buffalo, NY. pp. 28–31.
Scheuhammer AM, Norris SL. 1996. The ecotoxicity of lead shot and lead fishing weights. Ecotoxicology 5:279–295.
Scheuhammer AM, Perrault JA, Bond DE. 2001. Mercury, methylmercury, and selenium concentrations in eggs of Common Loons (Gavia immer) from Canada. Environ. Monitor. Assess. 72:79–84.
Sidor IF, Pokras MA, Major AR, Poppenga RH, Taylor KM, Miconi RM. 2003. Mortality of common loons in New England, 1987 to 2000. J. Wildlife Diseases 39:306–315.
Sperduto MB, Powers SP, Donlan M. 2003. Scaling restoration to achieve quantitative enhancement of loon, seaduck and other seabird populations. Marine Eco. Prog. Ser. 264:221–232.
Spitzer PR. 1995. Common Loon mortality in marine habitats. Environ. Rev. 3:223–229.