Abstract
Metal artifacts from the Paleometal Epoch (ca. 1100 BC–400 AD) of the Primorye (Russian Far East) have shed new light on the introduction of the earliest bronzes into the Pacific coastal areas of prehistoric Eurasia. However, little is known about raw material circulation and the role of metal in the context of inter-regional exchange. This paper investigates 12 copper artifacts from major Paleometal settlements using alloy composition, trace elements, and lead isotopes to explore the metal sources and distribution networks. The results suggest that most objects are made of a copper-tin alloy, but some have arsenic as a significant minor element . Geologically, copper is unlikely to have come from local ore sources, but rather from the Liaoxi corridor and Liaodong Peninsula in Northeast China. This may indicate an inland route of metal trade across Northeast China or alternately, a coastal route via the northern Korean Peninsula. Archaeologically, the combined study of artifact typology and chemistry indicates two possible origins for the metal: the Upper Xiajiadian culture in Northeast China and Slab Grave culture in Mongolia/Transbaikal. Remarkably, the connection with Upper Xiajiadian communities parallels the transport route along which millet agriculture spread from Northeast China to the Primorye during the Neolithic.
Introduction
The rise and spread of early metal use and associated technologies has been a major focus of research on the archaeology of the Eurasian steppe (Chernykh Citation2008). Most studies have focused on the evolution of early metallurgy in regions where metal production was concentrated, such as the southern Urals (Hanks and Doonan Citation2009; Sharapov Citation2017), Kazakhstan (Stöllner et al. Citation2013), the Minusinsk Basin (Legrand Citation2004), and northern China (Youshimitsu Citation2001). By contrast, comparably little attention has been paid to the development of metallurgy among early societies on the peripheries of these influential production centers. This article addresses the beginnings of copper metallurgy in one such peripheral area: the Primorye of the Russian Far East. It thus presents the first comprehensive archaeometallurgical study of early copper in the easternmost part of the Eurasian continent (). Due to the close proximity to Manchuria and the Korean Peninsula, the archaeology of Primorye exhibits a high degree of cultural dynamism and long-distance interaction from the Late Neolithic period (ca. 5000–3300 cal BP) as evidenced by the diverse ceramics, textile production, subsistence strategies (particularly millet agriculture), and exotic artifacts, such as ornaments made from a jade-like mineral and stone replicas of metal daggers and spearheads (Nelson et al. Citation2020; Popov, Zhushchikhovskaya, and Nikitin Citation2019; Tao et al. Citation2020; Zhushchikhovskaya Citation2018).
Figure 1. Map of research area and archaeological sites of Paleometal period. Study sites: (1) Siny Gai A; (2) – Elizavetovka-1; (3) Dvoryanka-1; (4) Vetrodui. Sites with bronze or iron artifacts; (5) Barabash-3; (6) Peschanny; (7) Chapaevo; (8) Cherepakha-7; (9) Oleny (Maihe) – 1; (10) Malaya Podushechka; (11) Solontsovy-2; (12) Kievka; (13) Krounovka-1; (14) Korsakovskoe-2.
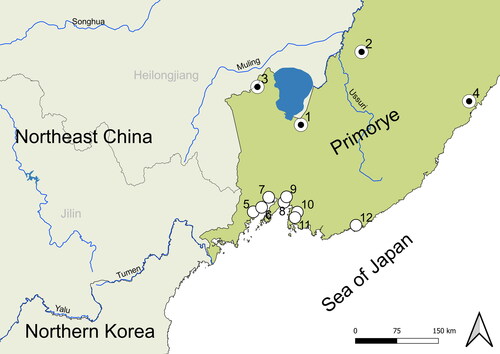
The earliest copper-based artifacts in the Russian Far East are found in settlements of the western Primorye dated to the late second millennium BC (Brodyansky Citation2013; Kon’kova Citation1989, 32–36; Nikitin Citation2012). This signifies the beginning of the Paleometal Epoch (ca. 1100 BC–400 AD), which is a transitional period characterized by the sporadic use of metal alongside stone tools before the widespread adoption of metal in the Iron Age (ca. fourth to seventh centuries AD) (Aikens, Zhushchikhovskaya, and Rhee Citation2009). A specific feature of early Primorye metallurgy is the rapid arrival of bronze and iron throughout the region, separated by a short chronological gap, allowing “Early Bronze” and “Early Iron” stages to be identified within the Paleometal period. The first bronze appeared between 1100 and 800 BC and the first iron between 700 and 500 BC, which has been taken as evidence for metallurgy having been transmitted to the region as a package rather than being invented locally (Popov, Zhushchikhovskaya, and Nikitin Citation2019). The idea that the first copper was introduced by new groups of people is supported by the simultaneous appearance of pottery tempered with crushed shell. Use of shell temper was not found in the Late Neolithic pottery of the Primorye, but it was practiced widely in parts of western and southern Siberia, Central Asia, and Northeast China from the Neolithic onward, meaning these groups potentially took the technique to the Primorye (Zhushchikhovskaya Citation1996, Citation2005, 128–133).
In addition, the coastal region of southern Primorye during the Paleometal period shows likely cultural contacts with the northern part of the Korean Peninsula. There are some similarities between the shapes and ornamentation of pottery assemblages from the sites of the Yankovskaya culture of the Primorye dated to around 9000–3000 BC and those at Yalu and Tumen river basins dated to the Mumun period of prehistoric Korea, ca. 1500–300 BC (Nelson Citation1993, 121–123). Furthermore, the Yankovskaya sites and middle and late Mumun sites contain a similar package of prestige goods, namely red-polished pottery, greenstone ornaments, and polished stone replicas of bronze daggers (Zhushchikhovskaya Citation2018). It is also important to note that in southern Primorye, a few examples of Korean-type bronze daggers and mirrors dated to the final Mumun period have been discovered (Kon’kova Citation1989, 44).
Overall, the Paleometal period in the Primorye is marked by distinctive archaeological communities, who were supposedly of different cultural backgrounds (Popov, Zhushchikhovskaya, and Nikitin Citation2019). The apparent cultural diversity represents a marked departure from the situation during the Neolithic. Rather than being the result of gradual local developments, the cultural and technological change seen at Paleometal sites likely testifies to external stimuli, probably involving the migration of people. The emergence of early copper in Primorye at this time may be positioned within the context of these cultural and demographic processes.
Previous studies
Initial evidence for the earliest metallurgy in the Primorye and neighboring areas was discovered in the 1960s (D’yakov Citation1989; Kon’kova Citation1989, 5–6; Okladnikov Citation1966). These were mostly single, occasional finds from non-stratified cultural layers that could not be reliably dated or associated with any particular archaeological context. The one exception was an assemblage of 21 copper-based artifacts that was reportedly unearthed from controlled excavation at the settlement of Siny Gai A and dated to the twelfth to ninth centuries BC (Brodyansky Citation1972).
An attempt to trace the origin of this assemblage was made in the late 1970s and 1980s by Lyudmila V. Kon’kova as a part of a general investigation of Far Eastern bronzes in prehistory (Kon’kova Citation1989). Based on artifact typology, chemical composition, and metallographic structure, she concluded that the morphological, functional, and chemical features of Primorye bronzes resembled the well-known Karasuk metallurgy of the Minusinsk Basin, which was spread widely across eastern Eurasia in the late second millennium BC. Early metals in Primorye are made of either copper-tin or copper-arsenic alloys, of which raw materials potentially originated from Siberia, specifically the Transbaikal (Zabaykal’ye) area. She suggested that initial bronzes and, probably, associated metal technology, were brought to the easternmost part of the Eurasian continent by new populations from the west, where large metal production centers had existed from the second millennium BC (Kon’kova Citation1989, 32–41 and 84). Later, Kon’kova employed lead isotopic analysis to pinpoint the geological ore sources for Primorye bronzes (Kon’kova, Fefelov, and Zarudneva Citation1990). The results pointed to the southern edge of the Siberian Platform, which encompasses the Altai-Sayan mountains, Kuznetsk Alatau range, the region of Krasnoyarsk, and the western part of the Transbaikal region. The bronze artifacts from Palaeometal sites in the Primorye were, therefore, likely rooted in the “Siberian tradition” of metal production (Kon’kova Citation1996, Citation2016, Citation2019).
From the 1990s onward, several settlements were excavated and yielded more evidence for the use of metals during the Paleometal Epoch (Nikitin Citation2012; Sidorenko Citation2007; Yanshina and Kluyev Citation2005). The majority of copper-based objects are concentrated in the settlements of the western and northwestern Primorye, all dated between the twelfth and eighth centuries BC. In contrast, sites in the central and eastern Primorye region produce single finds of metal from a later period: around 500 BC to 400 AD. The settlements associated with early metals do not show much cultural unity, as morphological and technological features of local pottery remain distinct (Nikitin Citation2012; Zhushchikhovskaya Citation2005, 128–133;). This may indicate that the process of early metal adoption in the Primorye was dictated by regional networks that were organized by several distinct communities rather than a single centralized production center.
Despite Kon’kova’s (Citation1989, Citation1996) scientific investigation, the Siberian origin of metallurgy in the Primorye was mainly based on limited archaeological and geological data. Over the past few decades, metal working remains have been excavated from numerous sites in Northeast China, which are not only contemporary with those in the Primorye, but also demonstrate cultural links to the region (Girchenko Citation2018; Nelson Citation1995, 147–250). Meanwhile, increasing numbers of lead isotope analyses on Russian and Chinese ore deposits have provided a large body of reference data that can be used to trace the geological sources of the ore used for the Primorye metals (Hsu and Sabatini Citation2019). The present study therefore represents a new phase in a scientific program that aims to analyze archaeological metals in relation to a comprehensive chemical and isotopic database of ores and relevant metal assemblages. In comparing the Primorye copper with this database, we characterize the origin, movement, and production of copper and its alloys in the Pacific region of Eurasia, which lies on routes that were crucial in the spread of domesticated crops and languages in prehistory (Nelson et al. Citation2020; Tao et al. Citation2020).
Materials and methods
A total of 12 copper-based artifacts were obtained from the Paleometal settlements of Siny Gai A (n = 7), Elizavetovka-1 (n = 3), Dvoryanka-1 (n = 1), and Vetrodui (n = 1) for the investigation of their chemical compositions and lead isotopic ratios (). Siny Gai A, Elizavetovka-1, and Dvoryanka-1 are spatially and temporally close to each other along the western part of the Primorye and date to ca. 1100–800 BC ( and ). These sites represent the earliest stage of the Paleometal Epoch. In contrast, Vetrodui in the eastern Primorye is relatively late, dating to the early first millennium AD, which represents the end of the period. A summary of archaeological information on the sites is presented in the online supplementary material (S1). It is important to note that those early sites in western Primorye represent the existence of various material cultures, as reflected by their distinctive pottery assemblages ().
Figure 3. (A) The average calibrated dates (“sum”) for each Paleometal site (OxCal v.4.4.2 [Bronk Ramsey Citation2009], calibration curve IntCal20 [Reimer et al. Citation2020]); and (B) chronology of main archaeological cultures discussed in this paper according to Svyatko et al. (Citation2009), Taylor et al. (Citation2019), Popov, Zhushchikhovskaya, and Nikitin (Citation2019), and Zhu (Citation1998).
![Figure 3. (A) The average calibrated dates (“sum”) for each Paleometal site (OxCal v.4.4.2 [Bronk Ramsey Citation2009], calibration curve IntCal20 [Reimer et al. Citation2020]); and (B) chronology of main archaeological cultures discussed in this paper according to Svyatko et al. (Citation2009), Taylor et al. (Citation2019), Popov, Zhushchikhovskaya, and Nikitin (Citation2019), and Zhu (Citation1998).](/cms/asset/8394681d-63ae-4f9a-8808-658a986f750f/uica_a_1958110_f0003_c.jpg)
The most typical metal artifact from the four study sites is a type of single-edged, perforated knife with a trailing point and semi-spherical buttons. These metal items were common among various groups located in Siberia, the Transbaikal, Central Asia, Northeast China, and the Korean Peninsula at the turn of the first millennium BC (Kon’kova Citation1989; Nelson Citation1993, 132–163, Citation1995, 147–250). A particularly distinctive item is a comma-shaped pendant from Vetrodui, which strongly resembles greenstone ornaments widely distributed over the Korean Peninsula, Japanese Archipelago, and other Paleometal sites in the early first millennium BC (Aikens and Higuchi Citation1982, 165, 173; Bale and Ko Citation2006; ; D’yakov Citation1989, 171; Nelson Citation1995, 132; Zhushchikhovskaya Citation2018). In addition to the artifacts mentioned above, there are some small items, including a fishhook and a ring-like item, which could have been manufactured locally.
In preparation for analysis, the samples were cut with a fine jeweler’s saw grade 6/0 blade (70 teeth per inch), then polished with 300-grit abrasive paper to remove remaining patina. To gauge the bulk composition, the specimens were dissolved in a 1:1 mixture of 7 N HNO3 and 6 N HCL, dried, and diluted with ultrapure water to a concentration of ca. 1000 mg/L. The diluted solutions were injected into a high resolution inductively coupled plasma mass spectrometer (HR-ICP-MS, Thermo Scientific Element XR™) at the Research Laboratory of the Deutsches Bergbau-Museum Bochum. Quantification was performed with external calibration. The solutions were diluted in 5% HNO3 to 1:100 for both main and minor elements then 1:10 for trace elements. The analyses were carried out with a SC-FAST automated sample introduction system ST5532 PFA μ-FLOW nebulizer, Peltier-cooled PFA spray chamber, and 1.8 mm sapphire injector in triple detector mode at all three mass resolutions (m/Δm) depending on the element of interest.
Measurements were controlled with compatible standards for the pure copper and copper-tin alloy, including BAM-376 (Bundesanstalt für Materialforschung und -prüfung) and BRONZE C (British Chemical Standards). Relative standard deviation for trace elements varied between 0.5 and 4.5%, and between 0.6 and 2% for main elements. As one ICP-MS analysis (SG5) yielded an erroneous analytical total (65%), the major elements of this sample were re-assessed using a Carl Zeiss SUPRA™ 40 field emission scanning electron microscope (FE-SEM) based on the procedures described by Hsu et al. (Citation2020).
The analysis of Pb isotopes started with the acid digestion and chromatographic separation as reported by Hsu et al. (Citation2020). The sample solutions were spiked with Tl isotopic standard NIST SRM 997 (National Institute of Science and Technology) and were measured with a Thermo Scientific NEPTUNE™ multi-collector inductively coupled plasma mass spectrometer (MC-ICP-MS) at Frankfurt Isotope and Element Research Center (FIERCE) of Goethe University Frankfurt. Except for the sample EP1, which had a higher experimental error (∼1% 2σ), the precision of the Pb isotope measurements was mostly less than 0.065%. Further details of the method can be found in Klein et al. (Citation2009) and Westner et al. (Citation2020). All results are reported in and .
Table 1. The lead isotopic results of the Primorye metal objects.
Table 2. The chemical composition of the Primorye metal objects.
Results and discussion
Characterization of the Primorye metals
The Primorye assemblage is mainly characterized by copper containing tin with two variants: tin-bronze and tin-bronze with arsenic as a significant minor element (). The first group contains less than 0.5% arsenic and comprises the objects from Siny Gai A and Dvoryanka-1 exclusively. The second group, with higher arsenic content, comprises the whole Elizavetovka-1 assemblage and two Siny Gai A objects. Copper-tin artifacts feature variable quantities of tin, typically ranging from ca. 1 to 12%. One exceptional item, a semi-spherical button found at Dvoryanka-1, comprises up to 41% tin. Conversely, lead content in the Primorye objects is normally present as an impurity below 1%, except for SG6, which has 1.4% lead. Previous optical emission spectroscopy (OES) analyses of Siny Gai A metals reported by Kon’kova (Citation1989) are consistent with the results of the present study, as both data sets show a similar spread of tin and arsenic. Unfortunately, the legacy data does not specify the archaeological context of each artifact, which precludes direct comparison of the new and old analyses.
Figure 4. (A) The tin and arsenic concentrations of the Primorye objects; and (B) trace element patterning of the Primorye objects. The shaded area represents 95% confidence level. The boxplot displays the legacy OES data from Kon’kova (Citation1989).
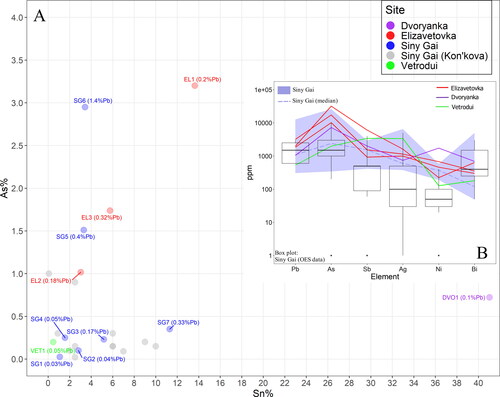
The trace element patterns allow the raw materials used at each site to be specified to an extent. The Elizavetovka-1 objects have elevated arsenic contents, which may be an impurity derived from the copper ore, while the Dvoryanka-1 button is rich in nickel (). Comparing these analyses with the old measurements reveals a discrepancy, as the OES data for Siny Gai A generally presents smaller quantities of antimony, silver, and nickel. This raises the issue of how legacy data should be treated in the discussion of metal provenance. A well-established problem with OES is that it suffers from poor precision and measurement repeatability due to unstable excitation conditions and development of the photographic plates (Pollard, Batt, and Young Citation2007, 48). Therefore, OES results tend to be less precise than those of recently developed analytical techniques. Due to these issues, this study does not consider trace elements from legacy data.
Although certain trace elements (e.g., arsenic, antimony, silver, bismuth, and nickel) could in principle indicate specific ore bodies that produced the copper used in certain artifacts (Pernicka Citation2014, 253), variation in element quantities is dependent on several parameters, such as the heterogeneity of the ores, smelting conditions, and whether metal has been recycled. Hence, lead isotopes can provide complementary evidence to determine provenance. Lead commonly occurs as a trace constituent in copper minerals and its isotopic composition is mostly unaffected by either geological weathering or pyrometallurgical processes, meaning that it is a good indicator for the source of raw material (Gale and Stos-Gale Citation1982).
Lead isotope ratios and their geological parameters (model age, 238U/204Pb, and 232Th/238U) for the Primorye objects are displayed in , in addition to the legacy data from Kon’kova, Fefelov, and Zarudneva (Citation1990) for the artifacts from Siny Gai A. The low concentration of lead in the samples indicates that the isotopic values principally reflect the composition of the original copper sources rather than the deliberate addition of lead during the manufacturing processes. A striking feature is that each site has a distinct distribution that can be clearly distinguished along the model age. The Siny Gai A objects yield a range of ages from ca. 500 to 700 Ma as opposed to the younger model ages of Elizavetovka-1 (ca. 350 Ma) and Vetrodui (ca. 150 Ma). There is even variation observable within a single site, as SG6 is readily distinguishable from the other Siny Gai A samples, which are also divided into two distinct clusters by the Th/U ratio. This variability, along with the distinct trace element patterns that define each site, suggest that metallurgy was practiced at the regional level, with each site having its own links with metal sources outside the region.
Figure 5. (A) Conventional plot of 206Pb/204Pb versus 207Pb/204Pb for the data from the present study and Kon’kova, Fefelov, and Zarudneva (Citation1990); (B) conventional plot of 206Pb/204Pb versus 208Pb/204Pb; (C) plot of the model age versus 238U/204Pb; and (D) plot of the model age versus 232Th/238U.
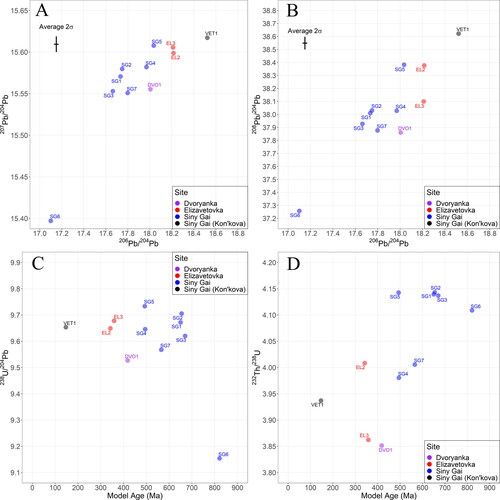
It is worth noting that the legacy data produced using thermal ionization mass spectrometry (TIMS) by Kon’kova, Fefelov, and Zarudneva (Citation1990) demonstrate systematically lower 207Pb/204Pb ratios than the present study’s results using HR-ICP-MS. Lead isotope studies conducted prior to the last two decades had difficulty measuring the less abundant isotope 204Pb accurately (Albarède, Desaulty, and Blichert-Toft Citation2012, 854). Additionally, before samples were routinely spiked with Tl, lead isotope analysis by mass spectrometry suffered from the significant effect of mass fractionation, which causes the measured isotopic compositions to be enriched with lighter isotopes (Chernyshev, Chugaev, and Shatagin Citation2007, 1066). The analytical errors of Kon’kova’s TIMS data range from 0.1 to 0.3%, which is far larger than in the present study. Consequently, the following discussion does not include the legacy data, as it is difficult to compare measurements of such different qualities.
Raw material sources for Primorye metalwork
There are three avenues by which people in the Primorye could have produced their bronzes: (1) primary production using local ore sources; (2) secondary production through the mixing and recycling of imported metals; and (3) direct import of finished objects from other metal-producing communities. Lead isotope geochemistry can be used to discriminate potential ore sources with the assistance of a referenced data base. Hence, a substantial lead isotope data set for sulfidic ores in the modern metallogenic districts of Northeast China, the Russian Far East, and Siberia, as well as analyses of copper-based artifacts from relevant archaeological communities, was collated to explore these possibilities. The distribution of referenced geological sources (classified as tectonic terranes) and metal artifacts are shown in , and the corresponding raw data and literature can be found in the online supplementary material (S2).
Figure 6. (A) Map showing major tectonic terranes in eastern Eurasia and occurrence of ore deposits from which lead isotope data were obtained, based on Nokleberg (Citation2010); and (B) map showing the distribution of sites with copper-based artifacts from which chemical and lead isotope data were collected.
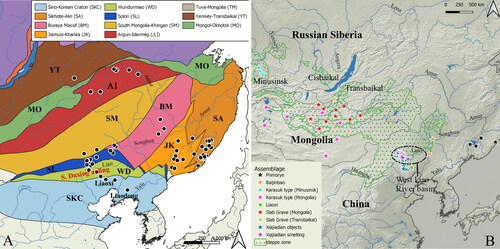
The Primorye is situated in a contact zone between the terranes of the Jiamusi-Khanka Massif (JK) and East Sikhote Alin (SA). Base metals in these units are hosted in hydrothermal vein and skarn deposits, which could have sustained local metal production (Rasskazov et al. Citation2002). Despite the presence of these local ore bodies, lead isotope ratios from the JK and SA deviate markedly from the signatures of the Primorye artifacts, as they have younger model ages (<300 Ma) and lower U/Pb ratios (<9.5) (). Hence, the possibility that raw materials from within the Primorye were used can be excluded, meaning that the appearance of bronze metalwork in this region derived from non-local sources.
Figure 7. (A) Conventional plot of 206Pb/204Pb versus 207Pb/204Pb for the data from the present study and ore samples in the vicinity of the Primorye (Rasskazov et al. Citation2002); and (B) plot of the model age versus 238U/204Pb.
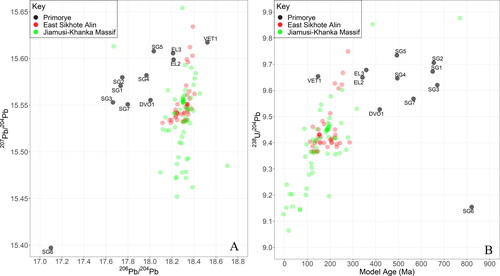
A supply network that relies on external metal sources would require the existence of an exchange network that connected the Primorye with other ore-rich regions. There were two potential routes along which metal could have flowed during the Paleometal period: (1) an inland route across Northeast China; and (2) a steppe route from the Transbaikal/Mongolia to the Amur River basin. The inland route has been highlighted as the principal path for the dispersal of millet agriculture from the West Liao River basin in Northeast China to the Russian Far East during the Neolithic (Tao et al. Citation2020). The West Liao River area sits within the southern Daxing’anling metallogenic belt, which is characterized by abundant polymetallic deposits (Niu et al. Citation2007). South of the Daxing’anling belt is the Sino-Korean Craton (SKC), which hosts rich gold and lead-zinc ore mineralization, particularly in the Liaoxi corridor and Liaodong Peninsula (Li and Santosh Citation2014). shows that, while the isotopic array of the Daxing’anling ores does not overlap with any artifacts, the Liaodong and Liaoxi data overlap with SG1, SG2, SG3, and SG6 from the Siny Gai A assemblage. Among these, SG6 is the only specimen that contains >1% lead, and its overlap with the Liaoxi ores might suggest the mixing of lead ores from this area. Overall, it is highly likely that an inland route across Northeast China fed Primorye metallurgy, particularly at the settlement of Siny Gai A. Alternatively, copper from the Liaodong could have been transferred along a coastal route via the northern part of the Korean Peninsula, but this route remains hypothetical due to a lack of published archaeological research from North Korea.
Figure 8. (A) Conventional plot of 206Pb/204Pb versus 207Pb/204Pb for the data from the present study and ore samples along the inland transportation route. The circled areas correspond to the kernel density estimation at 95% confidence levels; and (B) conventional plot of 206Pb/204Pb versus 208Pb/204Pb.
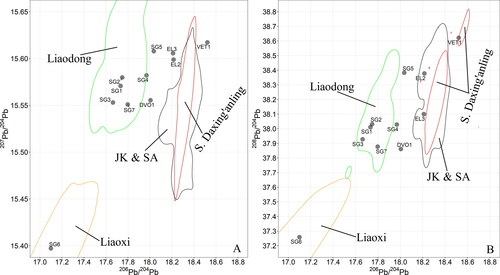
In addition, the SKC is one of eastern Eurasia’s major Archean cratons; therefore, the ore-forming process in this geological setting involved sources of lead older than those in other orogenic tectonic terranes, such as the Daxing’anling, JK, and SA. This is evidenced by the lower radiogenic lead signature, which corresponds to an older model age of ore bodies from the SKC in comparison with the terranes presented above.
The other route, here designated as the “steppe” route, lies along the Amur River valley and it could have linked the Primorye with the steppe zone of Siberia. A series of ore deposits hosted in the superterranes of the Bureya Massif (BM) and Argun-Idermeg (AI) are located between the Primorye and contemporary steppe communities, such as the Slab-Grave culture in central/eastern Mongolia and the Transbaikal. However, modern ores from the BM and AI exhibit a more radiogenic component, which is characteristic of younger lead sources (). Consequently, there appears to be no direct link between these modern ore-rich steppe areas and the Primorye metalwork.
Figure 9. (A) Conventional plot of 206Pb/204Pb versus 207Pb/204Pb for the data from the present study and ore samples along the steppe route. The circled areas correspond to the kernel density estimation at 95% confidence levels; and (B) conventional plot of 206Pb/204Pb versus 208Pb/204Pb.
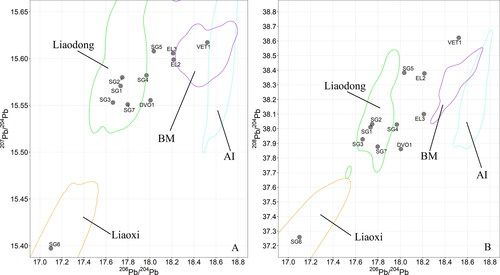
One of the most challenging tasks in the study of ancient metal is identifying potential recycling and mixing of metal, activities that are indicative of complex systems of metal circulation rather than a simple pathway between the origin and the deposition of artifacts (Pollard et al. Citation2018, 46). It is likely that the Primorye objects (SG4, SG5, DVO1, EL3, and EL2) that do not overlap with the modern ore signatures arrived in the region as the result of complex inter-regional interactions between archaeological communities instead of directly from any geological deposit.
The flow of metal artifacts along supply networks
While modern ore data can hint at the origins of prehistoric materials, it cannot provide a true picture of ancient exploitation, as modern ore geology focuses primarily on large economic deposits that were potentially not accessible to ancient miners. Moreover, provenance reflects only one episode of metal circulation and simply establishing the origin of ore does not explain how the people of the Primorye region engaged with metal. It is possible that people were unaware that some of their metal came from ore deposits several thousand kilometers away in modern Northeast China. More probably, they dealt with intermediaries who had immediate access to the mines and procured the metal for them. Such an exchange network would be based on the circulation of finished or semi-finished products that could be easily reworked into the desired form. To explore this, we compared the data produced in this study with metal objects from contemporary communities, with a particular goal of identifying source regions and their distribution among the Primorye assemblage. The metal-production powerhouses that could have supplied metal to the Primorye include the archaeological communities referred to as Upper Xiajiadian (1000–600 BC), Baijinbao (1100–800 BC), Karasuk (1400–900 BC), and the Slab Grave culture (1000–300 BC).
The first step in establishing connections between metal assemblages is the classification of alloy practices, as different combinations of alloy elements cause color variation in the final product, which prehistoric peoples will have been aware of and made choices accordingly. Primorye metallurgy shows a preference for copper-tin alloys with traces of arsenic (), which is fairly consistent with the Slab Grave artifacts from Mongolia and the Transbaikal. The compositions of metal artifacts from both regions are also observable in the trace element patterns (. This stands in stark contrast to the arsenical copper used by the Karasuk communities in the Minusinsk Basin. Although L. Kon’kova (Citation1989) suggested that metallurgy in the Primorye was probably adopted from the Karasuk culture, the chemical results presented here do not support this hypothesis. Instead, a close connection with Mongolia and the Transbaikal region is more likely, though it is difficult to establish whether the Primorye objects arrived in the region as finished products or were made locally from recycled metal.
Figure 10. (A) Ternary diagram Sn + Pb + As of metal assemblages from the Primorye, Karasuk, and Slab Grave cultures. The plot displays reactions, not exact values; (B) comparison of trace element signatures for artifacts from the Primorye (Siny Gai and Elizavetovka) and Slab Grave (Transbaikal) sites; and (C) comparison of trace element signatures for artifacts from the Primorye (Siny Gai and Elizavetovka) and Slab Grave (Mongolia) sites.
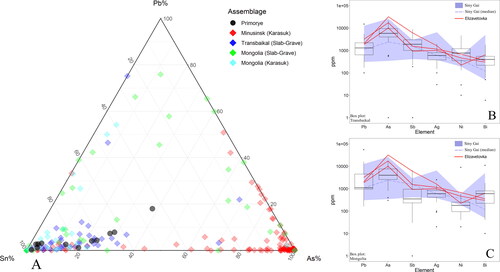
Other candidates that potentially contributed to alloy practices in the Primorye are archaeological communities in Northeast China, including the Upper Xiajiadian culture in the West Liao River region (Jaffe Citation2020) and the Baijinbao culture in present-day Jilin and Heilongjiang provinces (IAHP and DAJU Citation2009; IAJP Citation2011; Nelson Citation1995, 228–231). In Northeast China, the Upper Xiajiadian culture is the most significant late Bronze Age metallurgical center identified so far, and a full spectrum of metallurgical activity is attested, from the extraction of metal to the manufacture of finished products (Dong Citation2012). As shown in , Upper Xiajiadian assemblages comprise a variety of copper alloys, including copper-tin, copper-tin-lead, copper-arsenic, and copper-tin-lead-arsenic, which has been suggested as being indicative of the smelting of polymetallic sulfidic ores (Yang Citation2015). Upper Xiajiadian knives and buttons are characterized by different proportions of tin and arsenic, resembling the general trend in Primorye metallurgy. Similarly, metal objects from some Baijinbao sites are composed of a copper-tin alloy with arsenic. This indicates a connection between the Upper Xiajiadian culture and the Primorye metalwork due to: (1) direct import of finished metal products from Northeast China through trade and/or population migration; or (2) the mixing of imported scrap metal from various regions. The second scenario seems more probable, as the lead isotope results suggest that the Primorye metal had at least two origins (). The combined Upper Xiajiadian, Baijinbao, and Slab Grave isotopic field either covers the extent of the Primorye objects’ distribution or exhibits a similar alignment (exceptions are SG6 and VET1). The alignments of Primorye and Baijinbao with the Slab Grave metals, characterized by lower radiogenic lead signatures, is particularly striking.
Figure 11. (A) Ternary diagram Sn + Pb + As of metal assemblages from the Primorye, Upper Xiajiadian, Baijinbao, and Slab Grave cultures. The plot shows relations, not exact values; (B) conventional plot of 206Pb/204Pb versus 207Pb/204Pb for the data from the present study and artifacts from the Upper Xiajiadian, Baijinbao and Slab Grave cultures; and (C) conventional plot of 206Pb/204Pb versus 208Pb/204Pb.
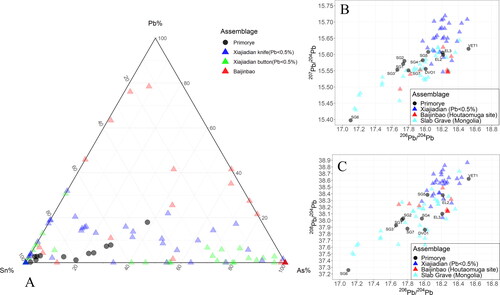
So far, archaeometallurgical investigation has allowed us to broadly identify two source regions for Primorye copper metallurgy: the West Liao River basin in Northeast China and the steppe region of Mongolia/Transbaikal. The Upper Xiajiadian culture in the West Liao River area has a varied bronze inventory, particularly notched, single-bladed knives and buttons that are typologically similar to their counterparts in the Primorye (). These types of metal objects also appear at Baijinbao culture sites, attesting to the influence of Upper Xiajiadian metalwork in other regions. The mismatch of isotopic compositions of Xiajiadian-type objects in the Primorye (SG1, SG2, SG3, SG7, and DVO1), however, suggests local people may have reproduced similar knives and buttons using raw material from other regions, probably from Mongolia/Transbaikal (see discussion below). The typological similarity between Primorye and Xiajiadian metalwork may therefore represent the movement of ideas rather than the import of metal objects finished completely in the West Liao River basin.
Figure 12. Typological comparison of copper-based knives and buttons of the Primorye, Minusinsk Basin, and Northeast China (Drawn after Chlenova Citation1972; Yang Citation2015; IAJP (Institute of Archaeology of Jilin Province) Citation2011; Yang, Hao, and Li Citation1990).
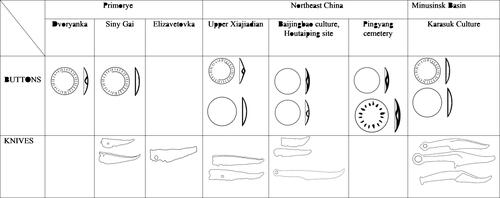
Primorye cultural groups can be linked to archaeological communities in Northeast China through two main types of evidence apart from metal. The first is pottery, as assemblages from Elizavetovka-1 and Baijinbao-type sites are similar (IAHP and DAJU 2009; IAJP Citation2011; Nikitin Citation2012). Shared features include the vessel shape, characterized by a relatively narrow, defined neck, a bulging body with concave shoulders, and a pointed, narrow base. The neck of the vessel has a tube-like shape that sometimes widens slightly at its base. In some cases, a pair of handles is attached at the level of the neck base.
The other type of archaeological evidence connecting the Primorye with Northeast China is the appearance of stone-built burials. At present, only one such burial has been found in the Paleometal period of Primorye at Dvoryanka-1, where it was used for a secondary burial. Such graves, however, are more characteristic of funerary practices at Xituanshan and Baoshan sites in neighboring Jilin Province of Northeast China (Nelson Citation1995, 147–250). Primorye’s links to Northeast China echo the intensive population movement occurring around the same time due to climatic changes and subsistence adaptation processes in Northeast Asia more broadly (Ning et al. Citation2020; Zhao Citation2012). The Primorye, at least the western part, was involved in drastic social transformations that caused the appearance of varied material culture, which included metallurgy.
Alternatively, the connection with the Eurasian steppe, specifically the Slab Grave community in Mongolia/Transbaikal, is another likely source from which Primorye metal could have come. Nevertheless, there is so far no archaeological evidence that explicitly links the steppe and the Primorye during the Paleometal period. Diagnostic elements of steppe cultures (e.g., horse domestication and zoomorphic motifs) are totally absent from all the excavated Primorye sites. Even though some metal knives and buttons from the Slab Grave and Karasuk cultures somewhat resemble those in the Primorye assemblages (), a connection with the metallurgical traditions of Northeast China seems more likely. However, the process by which the metal with chemical and isotopic signatures closer to that of the Slab Grave culture arrived in the Primorye remains unclear, particularly as there is no discernable steppe influence in Paleometal sites. Alternatively, steppe metalwork could have been transferred to the Primorye through the archaeological communities in Northeast China, where bronze objects decorated with zoomorphic figures were a typical artistic display of the mobile pastoralists in the Eurasian steppe during the first millennium BC (Nelson Citation1995, 147–250; Yang, Hao, and Li Citation1990).
Conclusions
Both the elemental and isotopic compositions of copper alloys from the Primorye region attest to a non-local origin for metallurgy during the Paleometal period in the Russian Far East. Variation is discernable between the different sites, and Siny Gai A, Elizavetovka-1, Dvoryanka-1, and Vetrodui each form a distinct group that supposedly had its own supply network showing individual contact with external communities. The uniqueness of each site’s metal consumption and use corresponds to the cultural diversity of Paleometal societies.
The major source region for Primorye metallurgy is Northeast China, specifically the West Liao River basin, which features the densest concentration of sites attributed to the contemporary Upper Xiajiadian culture. Using ore deposits exploited in the southern Daxing’anling, Liaoxi corridor, and on the Liaodong Peninsula, the Xiajiadian people forged an extensive metal network that reached communities belonging to the Baijinbao culture and in the Primorye, which is suggested by the production of Xiajiadian-type buttons and knives in the Primorye. This route of technological transfer may have followed the same path along which millet was spread in the Neolithic, a process that would involve the movement of people with the technological capabilities to make metal artifacts. The deviation of lead isotope ratios in the Primorye knives and buttons from similar Xiajiadian counterparts hints at the imitation of artifact types, where metals from different sources, mainly the Slab Grave culture in Mongolia and the Transbaikal region, were used to make objects seen in the Xiajiadian culture. Another possibility for the transmission of metal could have been a route via the coastal areas of Northeast China and the northern part of the Korean Peninsula as shown by the presence of Liaodong ore’s isotopic signatures in the Primorye metal objects. However, more archeometallurgical evidence of northern Korean ores and artifacts will be needed to verify this hypothetical connection. Finally, despite a lack of direct evidence for metal production in the early Paleometal settlements investigated in this study, it is clear that the Primorye was a dynamic “metallurgical landscape” (Radivojević et al. Citation2019), with multiple concurrent metal flows feeding the diverse metallurgical practices observed in this study.
Supplemental Material
Download Zip (4.1 MB)Acknowledgements
This study was carried out at the Deutsches Bergbau-Museum Bochum (DBM) and received great support from Professor Thomas Stöllner’s project “Resources in Societies,” funded by the Leibniz-Gemeinschaft (SAW-2017-DBM-3). We wish to thank Dr Michael Bode and Jan Sessing for their assistance during analytical work in the materials laboratory of the DBM. We also appreciate the constructive comments from two anonymous reviewers, which were helpful in improving the article.
Disclosure statement
No potential conflict of interest was reported by the authors.
Additional information
Funding
References
- Aikens, C. M., and T. Higuchi. 1982. Prehistory of Japan. New York: Academic Press.
- Aikens, C. M., I. S. Zhushchikhovskaya, and S.-N. Rhee. 2009. Environment, ecology, and interaction in Japan, Korea, and the Russian Far East: The millennial history of a Japan Sea oikumene. Asian Perspectives 48 (2):207–48.
- Albarède, F., A. M. Desaulty, and J. Blichert-Toft. 2012. A geological perspective on the use of Pb isotopes in archaeometry. Archaeometry 54 (5):853–67. doi:10.1111/j.1475-4754.2011.00653.x
- Bale, M. N., and M.-J. Ko. 2006. Craft production and social change in Mumun period Korea. Asian Perspectives 45 (2):159–87. doi:10.1353/asi.2006.0019
- Brodyansky, D. L. 1972. Raskopki u sela Siniy Gay v Primor’ye [The excavations at Siny Gai village in the Primor’ye]. In Archaeological discoveries of 1971, ed. B. A. Rybakov, 270–71. Moscow: Nauka.
- Brodyansky, D. L. 2013. Siniy Gay: Poseleniya Neolita i bronzovogo veka v Primor’ye [Siny Gai: Neolithic and Bronze Age settlements in the Primor’ye]. Vladivostok: Far Eastern Federal University Press.
- Bronk Ramsey, C. 2009. Bayesian analysis of radiocarbon dates. Radiocarbon 51 (1):337–60. doi:10.1017/S0033822200033865
- Chernykh, E. N. 2008. Formation of the Eurasian ‘steppe belt’ of stockbreeding cultures: Viewed through the prism of archaeometallurgy and radiocarbon dating. Archaeology, Ethnology, and Anthropology of Eurasia 35 (3):36–53. doi:10.1016/j.aeae.2008.11.003
- Chernyshev, I. V., A. V. Chugaev, and K. N. Shatagin. 2007. High-precision Pb isotope analysis by multicollector-ICP-mass-spectrometry using 205Tl/203Tl normalization: Optimization and calibration of the method for the studies of Pb isotope variations. Geochemistry International 45 (11):1065–76. doi:10.1134/S0016702907110018
- Chlenova, N. L. 1972. Khronologiya pamyatnikov karasukskoy epokhi [The chronology of Karasuk epoch sites]. Moscow: Nauka.
- D’yakov, V. I. 1989. Epokha bronzy v Primor’ye [The Bronze Epoch in Primor’ye]. Vladivostok: Far Eastern State University Press.
- Dong, L. 2012. Xiajiadian Shangcheng wenhua kuangye yizhi de kaocha yanjiu [Investigation and study on mining and smelting sites of the Upper Xiajiadian culture]. PhD diss., University of Science and Technology Beijing.
- Gale, N. H., and Z. A. Stos-Gale. 1982. Bronze Age copper sources in the Mediterranean: A new approach. Science 216 (4541):11–9. doi:10.1126/science.216.4541.11
- Girchenko, E. A. 2018. Keramika stoyanki Baytszin’bao – opornogo panyatnika epokhi bronzy v provintsii Kheyluntszyan [The pottery of the Baijinbao settlement – a diagnostic site of the Bronze Epoch in Heilongjiang Province]. Bulletin of Novosibirsk State University, Series - History, Philology 17:9–15.
- Hanks, B., and R. Doonan. 2009. From scale to practice: A new agenda for the study of early metallurgy on the Eurasian steppe. Journal of World Prehistory 22 (4):329–56. doi:10.1007/s10963-009-9031-5
- Hsu, Y.-K., and B. J. Sabatini. 2019. A geochemical characterization of lead ores in China: An isotope database for provenancing archaeological materials. PLoS One 14 (4):e0215973. doi:10.1371/journal.pone.0215973
- Hsu, Y.-K., B. J. Sabatini, N. Bayarkhuu, T. Turbat, P. Giscard, and S. Klein. 2020. Discerning social interaction and cultural influence in Early Iron Age Mongolia through archaeometallurgical investigation. Archaeological and Anthropological Sciences 12: 11 . doi:10.1007/s12520-019-00952-y
- IAHP (Institute of Archaeology of Heilongjiang Province), and DAJU (Department of Archaeology of Jilin University). 2009. Zhaoyuan Baijinbao: Nenjiang xiayou yichu qingtong shidai yizhi de jieshi [Baijinbao in Zhaoyuan county: Investigations of a Bronze Age site on the lower reaches of the Nonni River in Heilongjiang province]. Beijing: Kexue.
- IAJP (Institute of Archaeology of Jilin Province). 2011. Houtaiping: Dongliaohe xiayou you’an yi qingtong shidai yicun weizhu de diaocha yu fajue [Houtaiping: Examination and excavation of Bronze Age Sites on the right bank of the lower Dongliaohe]. Bejing: Wenwu.
- Jaffe, Y. Y. 2020. A grave matter: Linking pastoral economies and identities in the Upper Xiajiadian culture (1200-600 BCE), China. World Archaeology 52 (1):133–46. doi:10.1080/00438243.2019.1741437
- Klein, S., C. Domergue, Y. Lahaye, G. P. Brey, and H.-M. von Kaenel. 2009. The lead and copper isotopic composition of copper ores from the Sierra Morena (Spain) - Análisis de los isótopos de plomo y de cobre de los minerales de cobre de Sierra Morena (España). Journal of Iberian Geology 35 (1):59–68.
- Kon’kova, L. V. 1989. Bronzoliteynoye proizvodstvo na yuge Dal'nego Vostoka SSSR. Rubezh II-I tyc. do. n.e. – XIII vek n.e. [Bronze-casting production in the south of the USSR Far East. The border in the 2nd–1st millennium BC – 13th century AD]. Leningrad: Nauka.
- Kon’kova, L. V. 1996. The earliest bronze artifacts from the Soviet Far East. Bulletin of the Museum of Metals 26:1–20.
- Kon’kova, L. V. 2016. Drevniye I srednevekovyye dal’nevostochnyye bronzy i etnokul’turnoye vzaimodeystviye v Vostochnoy Azii [Prehistoric and Medieval Far Eastern bronzes and ethno-cultural Interaction in East Asia]. Historic Journal: Research Studies 6 (36):786–91. doi:10.7256/2222-1972.2016.6.19199
- Kon’kova, L. V. 2019. Epokha rannego metalla v Vostochnoy Evrazii: Problema kul’turnykh vzaimodeystviy [The Early Metal Age in eastern Eurasia: The issue of cultural relationships]. Brief Communications of the Institute of Archaeology of Russian Academy of Sciences 257:134–45. doi:10.25681/IARAS.0130-2620.256.134-135
- Kon’kova, L. V., N. N. Fefelov, and N. V. Zarudneva. 1990. The isotope composition of lead in bronzes from archaeological sites in the south of the Soviet Far East. Bulletin of the Metals Museum 15:41–4.
- Legrand, S. 2004. Karasuk metallurgy: Technological development of regional Influence. In Metallurgy in ancient eastern Eurasia from the Urals to the Yellow River, ed. K. M. Linduff, 139–56. Lewiston: Edwin Mellen Press.
- Li, S., and M. Santosh. 2014. Metallogeny and craton destruction: Records from the North China Craton. Ore Geology Reviews 56:376–414. doi:10.1016/j.oregeorev.2013.03.002
- Nelson, S., I. Zhushchikhovskaya, T. Li, M. Hudson, and M. Robbeets. 2020. Tracing population movements in ancient East Asia through the linguistics and archaeology of textile production. Evolutionary Human Science 2: E5. doi:10.1017/ehs.2020.4
- Nelson, S. M. 1993. The archaeology of Korea. Cambridge: Cambridge University Press.
- Nelson, S. M. 1995. The archaeology of Northeast China: Beyond the Great Wall. New York: Routledge.
- Nikitin, Y. G. 2012. Predvaritel’nyye rezul’taty issledovaniya poseeniya Elizavetovka-1 v Primor’ye [Preliminary results of the Elizavetovka-1 site investigations]. In The antiquities of Far East and Siberia, ed. A. P. Derevyanko, 41–50. Novosibirsk: Institute of Archaeology and Ethnography SO RAN Press.
- Ning, C., T.-J. Li, K. Wang, F. Zhang, L. Tao, X.-Y. Wu, S.-Z. Gao, Q.-C. Zhang, H. Zhang, M. J. Hudson, et al. 2020. Ancient genomes from northern China suggest links between subsistence changes and human migrations. Nature Communications 11: 2700. doi:10.1038/s41467-020-16557-2 10.10.38/s41467-020-16557-2.
- Niu, S., A. Sun, B. Wang, J. Liu, L. Guo, H. Hu, and C. Xu. 2007. On the metallogenesis of Dajing Cu-Sn polymetallic ore deposit, Inner Mongolia, China. Chinese Journal of Geochemistry 26 (4):394–401. doi:10.1007/s11631-007-0394-y
- Nokleberg, W. J, ed. 2010. Metallogenesis and tectonics of Northeast Asia. Reston: U.S. Geological Survey.
- Okladnikov, A. P. 1966. Arkheologiya doliny r. Zei i Srednego Amura [The archaeology of the Zeya River and Middle Amur valleys]. Soviet Archaeology 1:32–41.
- Pernicka, E. 2014. Provenance determination of archaeological metal objects. In Archaeometallurgy in global perspective: Methods and syntheses, ed. B. W. Roberts and C. P. Thornton, 239–68. New York: Springer.
- Pollard, A. M., C. M. Batt, and S. M. M. Young. 2007. Analytical chemistry in archaeology. Cambridge: Cambridge University Press.
- Pollard, A. M., P. Bray, A. Cuénod, P. Hommel, Y.-K. Hsu, R. Liu, L. Perucchetti, J. Pouncett, and M. Saunders. 2018. Beyond provenance: New approaches to interpreting the chemistry of archaeological copper alloys. Leuven: Leuven University Press.
- Popov, A. N., I. S. Zhushchikhovskaya, and Y. G. Nikitin. 2019. Paleometal epoch in the Primorye (south of the Far East of Russia). World Archaeology 51 (3):382–407. doi:10.1080/00438243.2019.1722737
- Rasskazov, S. V., V. V. Ivanov, A. I. Khanchuk, A. A. Chashchin, N. N. Fefelov, and E. V. Saranina. 2002. Isotopic heterogeneity of lead in galena from ore deposits in the Primor’e region. Doklady Earth Sciences 387:1083–7.
- Radivojević, M, B. W. Benjamin, E. Pernicka, Z. Stos-Gale, M. Martinón-Torres, T. Rehren, P. Bray, D. Brandherm, J. Ling, J. -J. Mei, H. Vandkilde, K. Kristiansen, S. J. Shennan and C. Broodbank. 2019. The provenance, use, and circulation of metals in the European Bronze Age: The state of debate. Journal of Archaeological Research 27: 131–185. doi:10.1007/s10814-018-9123-9
- Reimer, P., W. Austin, E. Bard, A. Bayliss, P. Blackwell, C. Bronk Ramsey, M. Butzin, H. Cheng, R. Edwards, M. Friedrich, et al. 2020. The IntCal20 northern hemisphere radiocarbon age calibration curve (0–55 Cal KBP). Radiocarbon 62 (4):725–57. doi:10.1017/RDC.2020.41
- Sharapov, D. V. 2017. Bronze Age settlement patterns and the development of complex societies in the southern Ural steppes (3500-1400 BC). PhD diss., University of Pittsburgh.
- Sidorenko, E. V. 2007. Severo-vostochnoye Primor’ye v epokhu paleometalla [North-east Primor’ye during the Palaeometal period]. Vladivostok: Dal’nauka.
- Stöllner, T., Z. Samašev, B. Sergej, J. Cierny, M. Doll, J. Garner, A. Gontscharov, A. Gorelik, A. Hauptmann, R. Herd, et al. 2013,. Zinn und Kupfer aus dem Osten Kasachstans: Ergebnisse eines deutsch-kasachischen Projektes 2003-2008 [Tin and copper from eastern Kazakhstan: Results of a German-Kazakh project 2003–2008]. In Unbekanntes Kasachstan: Archäologie im Herzen Asiens, ed. T. Stöllner and Z. Samašev, 357–82. Bochum: Deutsches Bergbau-Museum Bochum.
- Svyatko, S. V., J. P. Mallory, E. M. Murphy, A. V. Polyakov, P. J. Reimer, and R. J. Schulting. 2009. New radiocarbon dates and a review of the chronology of prehistoric populations from the Minusinsk basin, southern Siberia, Russia. Radiocarbon 51 (1):243–73. doi:10.1017/S0033822200033798
- Tao, L., C. Ning, I. S. Zhushchikhovskaya, M. J. Hudson, and M. Robbeets. 2020. Millet agriculture dispersed from Northeast China to the Russian Far East: Integrating archaeology, genetics, and linguistics. Archaeological Research in Asia 20: 100177. doi:10.1016/j.ara.2020.100177
- Taylor, W., S. Wilkin, J. Wright, M. Dee, M. Erden, J. Clark, T. Tuvshinjargal, J. Bayarsaikhan, W. Fitzhugh, and N. Boivin. 2019. Radiocarbon dating and cultural dynamics across Mongolia's early pastoral transition. PLoS One 14 (11):e0224241. doi:10.1371/journal.pone.0224241
- Westner, K. J., T. Birch, F. Kemmers, S. Klein, H. E. Höfer, and H.-M. Seitz. 2020. Rome’s rise to power: Geochemical analysis of silver coinage from the western Mediterranean (4th to 2nd Centuries BCE). Archaeometry 62 (3):577–92. doi:10.1111/arcm.12547
- Yang, J. 2015. Chifeng diqu qingtong shidai wanqi tongqi de kexue fenxi yanjiu [Scientific analysis of bronzes from the Late Bronze Age of Chifeng area]. PhD diss., University of Science and Technology Beijing.
- Yang, Z. J., S. Hao, and C. Q. Li. 1990. Pingyang muzang [Pingyang cemetery]. Beijing: Cultural Relics Publishing House.
- Yanshina, O. V., and N. A. Kluyev. 2005. Pozdniy neolit i ranniy paleometall Primor’ya: kriterii vydeleniya i kharakteristika arkheologicheskikh kompleksov [Late Neolithic and Early Paleometal of the Primor’ye: Criteria of determination and characteristics of archaeological complexes]. In The Russian Far East in prehistory and the Middle Ages: Discoveries, problems, hypotheses, ed. Zh. Andreeva, and N. Kradin, 187–233. Vladivostok: Dal’nauka.
- Youshimitsu, H. ed. 2001. Kodai Higashi Ajia seido no ryutsu [Circulation of ancient eastern Asian bronzes]. Tokyo: Kakuzando Shuppanbu.
- Zhao, B. 2012. Dongbei qingtong shidai kaogxue wenhua puxi geju de yanjiu [Research on the system of archaeological cultures in the Bronze Age Northeast China]. Bianjiang Kaogu Yanjiu 2:127–47.
- Zhu, Y. G. 1998. Dongbei qingtong wenhua de fazhan jieduan yu wenhua quxi [Development and cultural system of the Bronze Age cultures in Northeast China]. Kaogu Xuebao 2:133–52.
- Zhushchikhovskaya, I. S. 1996. On the systematization of Bronze Age sites in the Primorye region (pottery analysis). In Archaeology of northern Pacifica, ed. A. L. Ivliev, N. N. Kradin, and I. S. Zhushchikhovskaya, 317–21. Vladivostok: Dal’nauka.
- Zhushchikhovskaya, I. S. 2005. Prehistoric pottery-making of the Russian Far East. BAR International Series 1434. Oxford: Archaeopress.
- Zhushchikhovskaya, I. S. 2018. A non-ordinary goods complex of the Paleometal period in the southern Russian Far East. Archaeological Research in Asia 16:34–45. doi:10.1016/j.ara.2018.02.001