ABSTRACT
The air transport system consists of airports, airlines, and air traffic control (ATC). Airports are considered the system infrastructure occupying a certain area of land. This can be roughly divided into (i) the airside area containing runways, taxiways, and the apron-gate complex and (ii) the landside area including passenger and cargo terminals; space, buildings, facilities, and equipment for airport/airline-related activities; as well as the overall infrastructure of the airport ground access systems. Most airports are confronted with challenges such as incompatibility of land use and a lack of free land to expand to accommodate growing demand efficiently, effectively, and safely. To adequately deal with these challenges, an effective and compatible plan of airport land use needs to be developed with components such as (i) the airport design and operational criteria; (ii) requirements for safety of flights and unique land-use provision(s); and (iii) performances of land use. This article deals with analyzing, modeling, and assessing the physical/spatial, operational, economic, social, and environmental performances of land use by airport airside and landside areas. For such a purpose, a convenient methodology based on the indicators and their measures of performances of land use is developed and applied to selected airport cases.
1. Introduction
Like other systems, sustainability of the transportation system can increase its contribution to overall social and economic welfare by satisfying medium- to long-term demand efficiently, effectively, and safely while mitigating or maintaining its impacts on society and environment within the prescribed limits/targets (Janic, Citation2007). In such a context, the social and economic effects usually include maintaining economic sustainability of particular transportation sector actors and their contributions to local, regional, and national gross domestic product (GDP), mainly through employment in both transportation- and non-transportation-related sectors. Impacts on society include congestion, noise, and traffic incidents/accidents, i.e., safety. The environmental impacts include energy/fuel consumption, emissions of greenhouse gases (GHG), and land use. Land use is considered as an environmental impact when agricultural land is taken and when infrastructure is built close to populated areas, thus exposing the local population to noise and emissions of GHG from transportation operations. In many cases, this infrastructure, particularly large transportation nodes such as ports, airports, and rail stations, stimulates further populating of the neighborhood, thus again exposing the population to excessive noise and emissions of GHG.
Despite sophisticated evaluation of the social and economic feasibility of new transport infrastructure projects respecting local land-use plans, after their implementation, nearby residents usually start complaining about the noise, emissions of GHG, and sometimes induced congestion. Usually, in many of these cases the overall benefits obtained just by using land for building transportation infrastructure, instead of something else, are neglected or forgotten.
In general, transportation and land use are closely interrelated. shows an example of the relationship between the area of total converted agricultural land and the area of such converted land intended for transportation infrastructure in Europe.
Figure 1. Relationship between the area of converted agricultural land and the area of such converted land into transport facilities in 39 European countries during the period 2000–2006 (http://eurostat.ec.europa.eu/statistics).
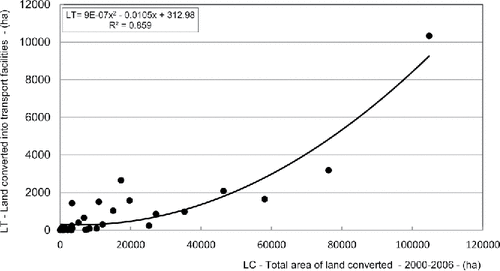
As can be seen, the share of area of land intended for transportation infrastructure has increased more than proportionally in the given case during the observed period.
Airports, as the infrastructure of the air transportation system including airlines and air traffic control (ATC), are no exception regarding these effects and impacts, i.e., sustainability. In particular, they sometimes occupy a substantial area of land. In the given context, airports occupy land directly and indirectly. Their direct occupation includes land used for aeronautical activities: accommodation of aircraft, passengers, and air cargo at the airport airside and landside area. The airside area includes runways, taxiways, and the apron/gate complex that enables handling of aircraft. The landside area consists of passenger and cargo terminal complexes and airport ground access transportation modes (usually road and different categories of rail) (Horonjeff & McKelvey, Citation1994). Indirect occupation includes land occupied by nonaeronautical activities around airports that otherwise would not be there. For example, these can be financial, retail, and logistics businesses, shopping malls, sport centers, etc. In some cases, particularly around large airports, these activities, together with the airports themselves, form urbanized entities called “airport cities” (Stevens & Baker, Citation2013; Reiss, Citation2007; Quinton, Citation2012).
Developments so far indicate that most airports have generally encountered, among others, two challenges related to land used for aeronautical activities. The first is the incompatibility of use of the already-allocated land and the second is the frequent lack of open or available land for expansion to accommodate the growing air transportation demand effectively, effectively, and safely. Both determine and at the same time influence the performances of land use by airports. In the given context, the term performances of land use means the transportation sustainability components (i.e., effects and impacts) of aeronautical activities (i.e., air transportation operations and related activities carried out on the airport's airside and landside areas) on society and the environment.
This article deals with analyzing, modeling, and assessing the physical/spatial, operational, economic, social, and environmental performances of land used by airports exclusively for aeronautical activities performed at their airside and landside areas. This means that the performances of land used for nonaeronautical activities around airports are not considered. For such a purpose, a methodology consisting of indicators and their measures of these performances is developed and applied to the selected airport cases. In such a context, particular indicators and measures of performances are also estimated across different and individual airports. In the latter case, two large European airports—Amsterdam Schiphol (AMS, the Netherlands) and London Heathrow (LHR, UK)—have been selected because of their differences in the patterns of aeronautical activities and the area of land taken. The idea behind the selection of particular cases is to demonstrate the generosity and flexibility of the proposed methodology, which can be applied to both types of cases.
In addition to this introductory section, the article consists of four other sections. Section 2 describes the main challenges related to the (in)compatibility of land use by airports. Section 3 describes the methodology, consisting of indicators and their measures of performances of land use. Section 4 describes an application of the proposed methodology. The last section summarizes some conclusions.
2. Airports and land use
2.1 Background
The area of land occupied by an airport is determined by its master plan (Horonjeff & McKelvey, Citation1994). In addition to airport-related elements, the master plan should fit the land-use policy of the region where the airport is located, and vice versa. Specifically, while allocating available land to other nonaviation purposes, the land-use policy should respect the presence of the existing and/or new airport and its prospective impacts on the local society and environment. This implies that allocation of land to and around an airport should not
stimulate the concentration of people (mainly housing) exposed to adverse aircraft noise, emissions of greenhouse gases (GHG), and risk of aircraft incidents/accidents; or
stimulate the development of activities and elements that could affect the airport's continuous operation and eventual future expansion.
Activities sensitive to excessive noise, the perceived risk of air traffic accidents, and those interfering with existing and prospective airport-aircraft-flight procedures, operational patterns, and navigational systems are potentially constraining. The constraining elements usually include excessively high buildings penetrating the airport's imaginary surfaces (ALUC, Citation1995; Horonjeff & McKelvey, Citation1994). Consequently, in addition to that occupied by the master plan (i.e., airside and landside area), the land where the nonairport or nonaeronautical activities and elements are restricted should also be considered as occupied by the given airport. Usually, this is the land below the airport imaginary approach, horizontal, departure, transition, and conical surface commonly used for the aircraft final approach and landing, as well as departure paths (Janic, Citation2013). Implementation of these requirements and principles of land-use policy enables compatibility of land use to be achieved; otherwise incompatibility generally causes impacts on the local population and the environment.
2.2 Incompatibility of land use
2.2.1 Some developments
Incompatibility of land use by airports occurs if some or all of these requirements and/or principles of compatible land-use policy are violated for any reason. For example, a recent study that surveyed 123 US commercial airports identified six types of potential land-use incompatibility scenarios, as given in Table 1.
These figures indicate that different types of land-use incompatibility are simultaneously present at the same airports (ACRP, Citation2010). The responsibilities for these incompatibilities are not always clear, i.e., if they arise as a result of the airport's impacts spreading toward areas already allocated to the other activities/purposes, the attractiveness of the airport's locations for aviation and nonaviation activities, or both.
2.2.2 Mitigating measures
In general, any existing incompatibility of land use by a given airport causes impacts/externalities on its neighborhood population and environment, which usually require some mitigating measures (CDT, Citation2011).
These can generally be divided into posterior and prior ones. Posterior measures are applicable to existing airports by imposing different restrictions on their operations such as the time, volume, and intensity of aircraft operations during the day, month, and/or year. Typically, constraints in the form of noise and/or air pollution caps on the volume of operations and related activities are imposed, usually affecting utilization of the airport's available operational capacity.
Prior mitigating measures apply while building new airports or expanding existing airports. They imply incorporating the airport development with all specificities (i.e., the master plan) into the local, regional, and frequently the national medium- to long-term plans of land use in combination with rather strict compliance with these plans. Such an approach enables reservation of sufficient land for new airports or expansion of the existing airports, in the latter case without increasing the incompatibilities already present.
In general, allocation of already available open/free land to an airport or some other activities depends on the local, regional, and sometimes national land-use policy on the one hand and on the estimated economic and social demands and related benefits and costs on the other. The question usually emerges of whether it is overall more socially and economically feasible (in terms of the total externalities and associated benefits) to allocate the available land to an airport or, for example, to agricultural, housing, community, commercial, industrial, or recreational activities or to the natural environment (green area with intact flora and fauna). In all these mutually exclusive cases, the allocated land and its medium- to long-term impacts have certain monetary values, which can generally be economic (market based) and noneconomic. By comparing these values, the open/free land can be allocated in a way that maximizes the perceived social and economic welfare (Cidell, Citation2004; FDT, Citation2012; Janic, Citation2007).
3. Methodology for assessing the performances of land use by airports
3.1 Some related research
Airport land-use issues and related performances have been the subject of both academic and agency-consultancy research. Academic research specifically (or directly) related to the performance of land use by airports has been rather scarce mainly due to two reasons: First, the issue of land use in the given context has been frequently considered as one of the environmental performances at a more general level, through assessment of sustainability of airports (Janic, Citation2007; Citation2010). Second, despite the general international guidance, the land-use issue by airports has always been considered locally, on a case-by-case basis, mainly in terms of fitting into the local and regional plans through the concept of (in)compatibility. In such a context, the value of properties on the land affected by the aircraft/airport noise, guidance for planning land use, and estimation of the area covered by particular noise contours have mainly been considered (ACRP, Citation2010; Arron & Stevens, Citation2008; CAA, Citation2012; CDT, Citation2011; Cidell, Citation2004; ICAO, Citation2002; FDT, Citation2012; Nelson, Citation2004; Freestone & Baker, Citation2010). In particular, this academic research has been carried out at two levels, the general and case specific. The former research worthy of mention in this context has been focused on reviewing the models dealing with the two-way interaction between transportation and land use with respect to their theoretical, methodological, and operational characteristics (Chang, Citation2006). In addition, the research has been devoted to evaluation of existing computer models of the interrelationship between land use and transportation including the long-term effects in changing the transportation cost and travel demand in urban areas. Four types of models have been examined (regression, mathematical programming, aggregate spatial interaction, and individual choice), aiming at recommending the most appropriate to be used for future policy decisions (Mackett, Citation1985).
The latter case-specific research has been mainly focused on compatibility of land use around airports in terms of noise and safety, i.e., exposure to air traffic accidents. For example, some of this research has indicated that the land-use planning around airports often has not facilitated the usually conflicting objectives of particular actors/stakeholders involved, which consequently resulted in disjointed decision making. Therefore, considering airport planning and consequent compatibility of land use in the wider context such as so-called airport cities and the interface between the airport and the region it serves from the perspectives of particular stakeholders has been proposed (Stevens & Baker, Citation2013). Some research is related to developing different topologies of the airport-city urban forms. Consequently, six models of the airport-driven urban development (airfront, decoplex, airport city, airport corridor, aerotropolis, and airea) with respect to the airport location, lead actors, and key reference literature have been elaborated, including real-life examples. These models have been discussed and compared with respect to three dimensions of sustainability: environmental, social, and governance. The need for considering the long-term sustainable development to be achieved throughout close cooperation with the main stakeholders involved—airport, region, and surrounding population—has been pointed out (Baker & Freestone, Citation2011).
In addition, it has been pointed out that the conventional airport master planning applied to most airports to date has resulted in increasing incompatibility of land in use, in terms of flexibility to adapt to increasingly frequent changes of traffic patterns, mainly due to not appropriately including preferences of particular actors/stakeholders. Consequently, a conceptual decision support system (DSS) has been proposed to overcome these weaknesses in airport master planning (Wijnen, Walker, & Kwakkel, Citation2008). Furthermore, redefining the existing planning models to include the increasing volatility of airports' operational environment and increased public sensitivity, particularly related to their impacts, has been suggested (Goetz & Szyliowicz, Citation1997).
Last but not least, research on land-use planning around airports, including checking compatibility of land use with respect to noise through reaction of neighboring residents, has been carried out for future development (Sadr, Nassiri, Hosseini, Monavari, & Gharagozlou, Citation2014). In this context, airports of different sizes have been investigated with respect to the noise burden they impose on the nearby population. It has been shown that both bigger and smaller airports have been faced with the serious noise problems. One of the examples of the bigger airports is Zurich international airport (Switzerland). The research has considered redevelopment of the population settlements, with respect to the sustainability criteria, by considering noise as a crucial problem for the future development of airport regions. In this case, knowledge of the potential for the redevelopment, renovation, and reorganization of the affected (mostly housing) settlements has shown to be essential for mitigating nose from the airport (Scholl & Nebel, Citation2014). Other recent research has dealt with the comprehensive analysis and measurement of noise burden around the Athens international airport (Greece). The noise maps include estimation of the number of population affected, the effects of noise-mitigating measures at source and by operations, improvement of the noise monitoring system, and dissemination of information on the mitigation efforts to the affected population and other public (Vogiatzis, Citation2014). Another equally relevant example suggests relocating the second-largest Greek airport (Heraklion) 37 km away from the current site because of exposure of the nearby population to the excessive airport noise. The first step has generally been obtaining an acoustic characterization of the airport operation inside the study area by measurement, simulation, subjective perception of aircraft noise (qualitative and quantitative results from interviews), and sound identity of the area. Then, the next step has been assessing the mitigating effects of noise due to relocating the airport and consequent redevelopment of the economic and social/cultural activities at the previously noise-affected locations. The suitable noise regulation has been proposed to be in place anyway (Vogiatzis & Remy, Citation2014). Particularly interesting cases of smaller airports faced with the relatively big problems of noise are the Larnaka airport in Greece and the Pisa airport in Italy, which have been used simultaneously for civil and military flights. In the first case, the research has developed models for measuring and monitoring noise, including the effects of noise-mitigating measures at the source (aircraft type, volume, and pattern of using runways), insulation of houses of the currently affected residents, managing the land use around the airport, and dissemination of information to public (Larnaka airport in Greece) (Vogiatzis, Citation2012). In the second case, the airport is located close to the city, implying that possible expansion would require relocation of the most affected residential areas. Comprehensive noise measurement and setup of contours for noise exposure have been carried out and then used as the basis for implementing and assessing the effects of some of the noise-mitigating measures. These have included defining the parts of the airport and the time of operating the aircraft engines and auxiliary power units (APUs), setting up the physical noise barriers, making the structural changes/partial reconstructions in the airport airside area, and developing the new noise abatement procedures. This scenario approach has been used to assess the effects of these measures, indicating how the nearby population could be affected by noise just by being close to the airport (Licitra, Gagliardi, Fredianelli, & Simonetti, Citation2014).
Finally, some studies have developed an indicator system for evaluating the sustainability of airports. In this system, the land-use issue by airports has been considered as an impact of the given airport on the surrounding environment (Janic, Citation2007).
Agency-consultancy research has mainly focused on developing some generic and case-specific guidance on compatibility of land use by airports, which could be used by policy makers and partially serve as a basis for related planning regulations. One example is the guidance for conducting land-use compatibility planning according to the valid local and central regulations for all existing and new airports, aimed at setting standards to prevent excessive noise and safety hazards (CDT, Citation2011). The other is research on identifying the scope and content of compatibility of land use at airports as a tool for policy makers. This tool includes fundamentals of land use and implementation resources, compatibility concerns, roles and responsibilities of stakeholders, regulations and guidance, the economic cost of land-use incompatibility, aircraft noise and land-use incompatibility, aircraft accidents and safety considerations, and tools and techniques for land-use compatibility (TRB, Citation2010). In addition, research has focused on the management and control of incompatibility of land use by the selected set of airports based on noise-complaint patterns. The results have shown that the population closer to airports has tended to increase faster than other populations, but that a disproportionally greater number of complaints have come from a few households. This suggests that airports should prevent the approach of new residents (Ming Li, Eiff, Laffitte, & McDaniel, Citation2007). This has been the case particularly at smaller regional airports where the planning authorities have often not sufficiently recognized and respected their local importance while constraining land use to mitigate their environmental impacts (WSDT, Citation1999).
Last but not least, land use around airports has been the subject of regulation at the local, regional, national, and international scale. Such regulation has mainly aimed to specify the rules of land use by airports to mitigate impacts of noise and emissions of GHG on the local population by maintaining them within prescribed limits. For example, one of the most recent endeavors of such regulation on the international scale has been by the European Union (EU), which has considered land-use planning and management as one among four measures for mitigating noise around airports (EC, Citation2011).
Consequently, a consistent methodology for the systematic and complete analysis, modeling, and assessment of the performances of land use by airports has truly been lacking. This article intends to contribute to filling this gap.
3.2 Objectives and requirements
As mentioned, the issue of land use has usually been dealt with within the scope of sustainability of airports and the entire air transportation system. In addition to direct energy/fuel consumption and emissions of GHG from aircraft operations and related activities, land use has been considered as an impact on the environment. At the same time, the effects in terms of social and economic benefits obtained by such land use, instead of alternate uses, have often been neglected. This has been mainly dependent on the interests of particular stakeholders involved; on one side is the air transportation industry—airports and prevailing local, regional, and national related policies—and on the other side is the attitude of local population, which is usually against any further expansion of airports while at the same time ignoring the direct benefits of their proximity to the airport. The objectives of this article are to develop and illustrate the potential application of a methodology for analyzing, modeling, and assessing the performances of land use by airports. In the given context, these performances relate only to impacts and effects of land taken exclusively by the airport airside and landside areas, accommodating aircraft and passengers, This implies that the performances of land used for a variety of supporting and commercial activities in the close proximity of an airport (access systems, various logistics activities, commercial and financial businesses, shopping malls, etc.) are not considered.
Therefore, the performances of direct land use by airports are categorized as physical/spatial, operational, economic, social, and environmental. As in many similar cases, these performances can be interrelated with each other as shown in (Janic, Citation2010, Citation2014; PIA, Citation2002),
As can be seen, an interrelation in both directions (top-down and bottom-up) can generally exist between particular performances.
The methodology consists of indicators and their measures reflecting the mentioned performances including their possible interrelationships; the indicators and measures should be, regarding the requirements, as follows:
sufficiently generous to be uniquely applicable to different airports;
relevant for the particular actors/stakeholders involved;
able to express particular performances as effects/benefits and impacts/costs;
as precise and clear as possible (in terms of being descriptive and quantitative, the latter in either absolute or relative terms by using the available data);
updatable over time to enable continuous monitoring of the specific and overall performances as well of land use by airports; and
relate exclusively to the land occupied by the airport airside and landside areas used for aeronautical activities, accommodating aircraft and passengers.
Specifically, the particular indicators and measures of performances can be qualitative and quantitative. The former are mainly descriptive and involve grading the performances as unsatisfactory, satisfactory, good, very good, and/or excellent as compared to the specified benchmarks. The latter are expressed in absolute and/or relative terms, i.e., by the specified quantity of particular effects/impacts per unit of occupied (taken) land under the given conditions during the specified period of time. As such, these indicators and measures appear to be relatively static, but they could be made dynamic if considered for a series of successive periods of time (for example, several consecutive months or years).
Consequently, the proposed methodology could be used by researchers as well as by the particular main stakeholders involved such as the planning/policy authorities at the local, regional, and national level, the airport operators, third parties represented by existing and prospective neighboring inhabitants, and airlines. The purposes can be as follows:
Assessment of the effects/benefits and impacts/costs, i.e., the overall feasibility, of allocating open/free land to an airport instead of to some other socioeconomic activities (this implies that a similar methodology for assessment of performances of land use by these activities is available).
Assessment of feasibility of expanding an existing airport if taking substantial new land (for example for building a new runway).
Monitoring and comparing the performances of land use at different airports, undertaking measures to mitigate incompatibilities, and refining existing requirements and principles of the corresponding land use policy in a systematic and unique way.
Using them as criteria while evaluating the overall sustainable development of particular airports.
Using them as an input when deciding whether to settle near an airport based on its current and prospective land use performances.
The stakeholders are usually interested in different performances. For example, the planning and policy authorities at different levels could be mostly interested in all performances including their prospective interrelationships both internally (at the level of a given airport) and externally (at the level of the neighboring populated areas, which can be a part of or the entire city, region, and in some cases country). Airport operators are mainly interested in the physical/spatial, operational, and economic performances. Third parties could be primarily focused on the social and environmental performances.
Airlines as the last stakeholders are usually under pressure from other stakeholders to make as minor as possible impacts on third parties—the neighboring population and the environment—and at the same time provide the expected efficiency, effectiveness, and safety of services to passengers and cargo demand.
3.3 Indicators of performances
3.3.1 Physical/spatial performances
The indicators and measures of physical/spatial performances of land use by airports and corresponding indicators and their measures are considered to be (i) the reference code usually specified in advance, i.e., before an airport has been built or later after being expanded; and (ii) the spatial layout, i.e., size and composition/configuration of the airport airside and landside area.
3.3.1.1 Reference code
The airport reference code reflects the airport size, type, and volume of air transportation demand expected to be accommodated under given conditions, and consequently the area of land to be taken. In general, an airport reference code defined by both the United States Federal Aviation Administration (US FAA) and the International Civil Aviation Organization (ICAO) is based on characteristics of the aircraft expected to operate there. The US FAA uses two terms, with the alphabetical letter reflecting the aircraft approach speed category (A, B, C, D, E) and the numerical term reflecting the aircraft design group (I, II, III, IV, V, VI). The ICAO uses a similar alphabetical (A, B, C, D, and E) and numerical (1, 2, 3, and 4) scheme. According to both codes, airports with longer alphabetical and higher numerical signs are spatially larger, implying their ability to accommodate larger aircraft serving larger volumes of air transportation demand (FAA, 2012; Horonjeff & McKelvey, Citation1994; ICAO, Citation1987).
3.3.1.2 Layout: The area and shape of land
The layout reflects the area and shape of land occupied by an airport of the specified category (reference code). This area consists of the ground and the airspace part. The ground part is composed of the airport airside and landside area. The airside area is characterized by the size and shape of land earmarked to become the runways, taxiways, and apron/gate complex. The landside area is characterized by the size and shape of land for constructing passenger (and cargo) terminals and the airport ground access systems, all with the corresponding supporting facilities and equipment. Under the given conditions, the airport layout needs to be as compact as possible, thus occupying an area of land as small as possible. shows the simplified scheme of six typical (theoretical) airport land footprints with their main geometrical parameters. These are (i) a single runway used for both landings and takeoffs; (ii) two parallel runways used both for landings and takeoffs; (iii) two parallel runways of which one is used for landings and the other for takeoffs; (iv) two converging runways each used for both landings and takeoffs depending on the prevailing wind; (v) two parallel plus one crossing runway each used for landings and takeoffs; and (vi) two pairs of parallel runways of which two outer runways are used for landings and two inner runways for takeoffs.
The minimal (standard) values of particular parameters are recommended by the ICAO (ICAO, Citation1987). For example, for airports accommodating the largest aircraft (categories D and E) these are as follows: d = 300 m; h = 500 m; l = 500 m; L = 4500 m; d0 = 2000 m; d01 = d02 = 1050 m. Consequently, the minimum area of occupied land can be A = 260 ha for configuration a, 1035 ha for configurations b and c, 878 ha for configuration d, 1,179 ha for configuration e, and 1,980 ha for configuration f (Horonjeff & McKelvey, Citation1994; Janic, Citation2013). However, in practice, most airports actually occupy a wider area of land than the theoretical calculations.
The airspace part consists of the safety areas and imaginary surfaces established around each airport's runway. These include the runway safety areas, the runway object-free areas, and the runway protection zones (RPZs). The land below these safety areas defined and implemented by the national aviation authorities is intended to enable safe, efficient, and effective operation of a given airport.
The first two safety areas are already contained in the ground part of the airport land use. The RPZ, also known as the clear zone, is originally established to define the land areas below the aircraft approach paths aiming at preventing developments of incompatible land use, and particularly for protecting the neighboring population and properties from air traffic incidents. The RPZ of a trapezoidal shape is located at both ends of the runway(s) and centered on its extended centerline(s). Its dimensions depend on the type of approach visibility minima. Typically, the area of RPZ for all aircraft preferred to be fully controlled by the airport could be about 19.2–30.9 ha (0.192–0.309 km2). The imaginary surfaces around each runway should be free of obstacles to avoid impeding aircraft safe approach, landing, takeoff, and departure. These are the primary, approach, transitional, horizontal, and conical surfaces. Their size mainly depends on the type of runway approach. For example, the approach surface for precision instrument approach has a trapezoidal shape with widths of 0.3 km (inner end) and 4.9 km (outer end) and a length of 15 km, with the area of land below this surface amounting to about 3,600 ha (36 km2) (ACRP, Citation2010; Horonjeff & McKelvey, Citation1994).
3.3.2 Operational performances
The operational performances of land use by airports and corresponding indicators and measures are considered to be (i) capacity, (ii) intensity, and (iii) effectiveness of land use.
3.3.2.1 Capacity of land use
The capacity of land use can be expressed by the maximum number of air transportation movements (ATMs) carried out on a unit of land occupied by a given airport during the specified period of time as follows:(1) where
C(T) | = | is the maximum number of ATMs (i.e., capacity) carried out at the airport during the time (T) (ATMs/year) (one ATM is equivalent to a landing or a takeoff) (ATMs/year) and |
A(T) | = | is the area of land occupied by a given airport during the time (T) (ha or km2). |
The variable C(T) in Eq. (Equation1(1) ) can be an operational, economic, or environmental capacity. The area of land A(T) can be either that within the airport boundaries (i.e., its airside and landside area) or a wider area equivalent to the airport noise footprints (Janic, Citation2007). This measure is preferred to be as high as possible.
3.3.2.2 Intensity of land use
The intensity of land use can be expressed by the number of workload units (WLUs) accommodated per unit of land occupied by an airport during the specified period of time as follows:(2) where
WLU(T) | = | is the number of WLUs accommodated at given airport during the time (T) (one WLU is the equivalent of one passenger and his/her baggage or 100 kg of air cargo. |
The intensity in Eq. (Equation2
(2) ) expressed in the number of WLU/ha/year can also be expressed in the volumes of p-km or t-km realized per unit of land occupied by a given airport. For such a purpose, the length of incoming and outgoing flights, and the number of passengers and the volumes of freight on board of each flight need to be taken into account. In addition, similarly as in Eq. (Equation1
(1) ), the number of WLU(T) can be prescribed in advance to keep the volumes of airport traffic under the prescribed constraints (Eriksen, Citation2000). This measure is preferred to be as high as possible.
3.3.2.3 Effectiveness of land use
The effectiveness of land use can be expressed by the punctuality of ATMs carried out per unit area of land A(T) occupied by a given airport during the specified period of time. Usually, punctuality is expressed by the proportion of on-time arriving and departing flights (i.e., exactly according to schedule and delayed less than or up to 15 min). Under such conditions, the area of land does not directly influence this rate since other demand management measures are usually used to balance demand with the airport capacity, which in turn is influenced by the area of occupied land. Consequently, this measure can be expressed as follows:(3) where
pa(T), pd[pa(T)] | = | are the proportion of on-time arriving and departing flights during the time (T) (dimensionless); and |
Na(T), Nd(T) | = | are the total number of arriving and departing flights during the time (T), respectively (number of units). |
The total number of flights Na(T) and Nd(T) in Eq. (Equation3(3) ) can be at the level of corresponding airport capacities [Eq. (Equation1
(1) )]. In addition, the proportion of flights departing on time can generally depend on that of the arriving flights, and vice versa. Effectiveness
is expressed by the number of flights/ha/year. The other symbols are as in the previous equations. This measure is preferred to be as high as possible.
3.3.3 Economic performances
The economic performances of land use by airports and corresponding indicators and measures are considered to be (i) economies and (ii) diseconomies of land use.
3.3.3.1 Economies of land use
The economies of land use can be measured in terms of (i) profits, (ii) employment, and (iii) contribution to the overall local, regional, and national social and economic welfare achieved per unit of land occupied by the given airport. This measure can be expressed as follows:(4) where
PR(T) | = | is the net profit achieved by operating the airport during the time (T) (€ or $US/year); |
E(T) | = | is the direct (and indirect) employment by the airport during time (T) (number/year); and |
GDP(T) | = | is GDP from operating an airport during the time (T) (€ or $US/year). |
In general, the profits P(T) represent the difference between the airport's operational revenues and costs. Employment E(T) is the sum of direct and indirect employment by the airport. Its contribution to the overall social and economic welfare is the GDP(T) created by the given airport (Janic, Citation2007). The variables PR(T), E(T), and GDP(T) in Eq. (4) can be expressed either in absolute (an amount per period of time, usually 1 year) or in relative terms (an amount per unit of accommodated demand per period of time, usually 1 year). If these submeasures are positive, then they are preferred to be as high as possible; otherwise they should be as low as possible.
3.3.3.2 Diseconomies of land use
The diseconomies of land use are considered through the changes to the value of properties near a given airport exclusively due its impacts on the local population and environment such as aircraft noise and emissions of GHG. For example, in the case of the impact of aircraft noise, this measure can be expressed as follows:(5) where
Dp(Ap,T) | = | is the density of (housing) properties within the area of land (Ap) affected by aircraft noise during the time (T) (property units/km2); |
Vp(T) | = | is the value of property unit exposed to the impact of aircraft noise during the time (T) (€/property unit); |
rlvp(T) | = | is the rate at which a property unit loses value due to the incremental increase in the aircraft noise during the time (T) (%/1dB/year); |
ΔFn(T) | = | is the marginal increase in the aircraft noise due to increasing of the number of ATMs during the time (T) (dB/year). |
The marginal increase in aircraft noise ΔFn(T) in Eq. (5) can be determined as the difference between the factors of increase in the airport noise due to increasing of the number of ATMs in two successive time periods (T) and (T – 1) as follows: ΔFn(T) = Fn[N(T)] – Fn[N(T – 1)], where Fn[N(*)] = 10 log10 N(*).
The loss of the value of properties is expressed in monetary terms per unit of affected land, i.e., €/km2. This measure is preferred to be as low as possible (Janic, Citation2007; Smith, Citation1989).
3.3.4 Social performances
The social performances of land use by airports and corresponding indicators and measures are considered to be (i) the intensity of noise exposure and (ii) the third-party exposure to the risk of fatal aircraft accidents.
3.3.4.1 Intensity of noise exposure
The intensity of noise exposure is based on the area of a given noise contour(s) Ap(T) and the number of inhabitants P(T) within it. The noise contours represent different levels of continuous noise during the specified time (T). In general, durable noise is usually measured by Leq (equivalent continuous sound level), which takes into account the sound exposure level by individual events and the number of these events during the specified period of time (Leq is usually used with a dB A-weighted scale and expressed as LAeq, during the day or night hours, or their combination). Usually, this is the 57LAeq/16h and 57LAeq/8h contours during the day and night, respectively) (AC, Citation2013). This measure can be expressed as follows:(6) where
= | is the number of inhabitants within the noise contour |
The other symbols are analogous to those in the previous equations. The variable N(T) in Eq. (Equation6(6) ) can also be replaced by the airport capacity C(T) [Eq. (Equation1
(1) )]. This measure is expressed by the number of inhabitants within a given noise contour per ATM accommodated at a given airport during the specified time period: As such, it is preferred to be as low as possible and constant. Theoretically, the former can be achieved by depopulating the area without increasing the number of ATMs; the latter can be achieved by simultaneously balancing both influencing variables—decreasing the population and increasing the number of ATMs, or vice versa (AC, Citation2013; Nelson, 2003).
3.3.4.2 Third-party risk from fatal aircraft accidents
An increased concentration of inhabitants residing nearby the runway approach and departure paths increases their exposure to the risk of a fatal aircraft accidents (ACRP, Citation2010). This exposure risk of the population within the unit area of exposed land can be expressed as follows:(7) where
rfac(T) | = | is the rate of fatal aircraft accidents during the time (T) (events/ATM); |
pfac/ap(T) | = | is the probability that a fatal accident will occur in the direct vicinity of the given airport during the time (T); |
P(Ac, T) | = | is the number of inhabitants residing within the potential aircraft accident risk area Ac(T) around a given airport during the time (T) (persons); and |
Ac(T) | = | is the area of land exposed to fatal aircraft accidents (ha). |
The other symbols are analogous to those in the previous equations. In Eq. (Equation7(7) ), it is assumed that the population is uniformly distributed within the area of land Ac(T). In addition, the variable pac/ap(T) denotes the cumulative probability of a fatal accident occurring during aircraft approach, landing, takeoff, and the initial climb phase of flight (Boeing, Citation2013). This measure, expressed by fatal accidents-persons/km2, is preferred to be as low as possible.
3.3.5 Environmental performances
The environmental performances of land use by airports and related indicators and measures are considered to be (i) intensity of the energy consumption and (ii) intensity of the related emissions of GHG.
3.3.5.1 Intensity of energy and fuel consumption
The energy and fuel consumed by a given airport can be broadly divided into that consumed by operations in the airport airside and landside area and that consumed for aviation and nonaviation support activities. In the airport's airside area, this refers to the fuel consumed during the aircraft landing and takeoff (LTO) cyclesFootnote1 and servicing at the apron/gate complex. In the airport landside area, the main consumers of energy are the passenger and cargo terminals, other buildings at the airport, and the airport ground access systems (Janic, Citation2007).
The intensity of fuel consumption in the airport airside area by the aircraft LTO cycles can be estimated as follows:(8) where
N (T) | = | is the number of LTO cycles carried out during the time (T) and |
FCi/LTO(T) | = | is the fuel consumption per LTO cycle carried out by the aircraft of category (i) during the time (T) (tons/LTO cycle/year). |
The fuel consumption FCi/LTO(T) in Eq. (Equation8(8) ) usually depends on the aircraft size and the actual length of the LTO cycles, whose number can also equal the airport capacity [Eq. (Equation1
(1) )]. This measure is expressed in tons of fuel/km2. Experience so far indicates that the energy consumed by other operations and activities in the airport airside and landside area can be easily allocated to that of LTO cycles to arrive at the total energy consumption under the given conditions. This measure is preferred to be as low as possible.
3.3.5.2 Intensity of emissions of GHG
The energy consumption during LTO cycles and other supporting activities generates GHG.Footnote2
The GHG emitted during the LTO cycles are mainly shown to be the greatest relative contributor to the total emissions of GHG by a given airport (about 60%), followed by the ground aircraft servicing at the apron/gate complex (about 20%), the airport ground access systems/modes (15%), and electricity consumption in the airport buildings (about 5%) (Janic, Citation2007). Based on Eq. (Equation8(8) ), the intensity of emissions of GHG can be estimated as follows:
(9)
Where
ei/LTO(CO2e) | = | is the emission rate of CO2e (carbon dioxide equivalents) per LTO cycle carried out by the aircraft category (i) (kgCO2e/kg of fuel). |
The other symbols are analogous to those in Eq. (Equation8(8) ). The emission rate ei/LTO(CO2e) is based on the emission rates and relative proportions of particular air pollutants in the total emitted GHG. Expressed in tons of CO2/km2, and thus expressing GHG concentration over the area of a given airport, this measure is preferred to be as low as possible.
3.3.6 Summary and relationships of performances and their indicators and measures
The indicators and measures of performances of land use by airports are summarized in .
Table 1. Incompatibility of land use at US airports.
Table 2. Summary of land-use performance indicators of airports.
The sign + denotes that the preferred value of the given indicator and its measures is as high as possible and the sign – indicates that the preferred value is as low as possible.
As mentioned, the particular performances of land use by airports in are inherently interrelated as shown in . Qualitatively, according to the top-down approach at the level of airport planning and design, the possible interrelationships can be as follows:
Area of land taken = f (number and configuration of runways)
Number and configuration of runways = f (required capacity)
Required capacity = f (expected air passenger and cargo demand to be served)
Expected air passenger and cargo demand = f (demand driving social and economic factors)
shows some typical examples of the interrelationships between the area of land taken and the number and configurations of runways. gives some examples of the interrelationships between the number and configuration of runways and the airport airside capacity from US planning practice.
Figure 2. Generic scheme of the interrelationships between particular performances of land use by airports (Janic, Citation2014).
Table 3. Interrelationship between the airside capacity and the number and configuration of runways.
Again qualitatively, but this time according to the bottom-up approach, the interrelationship between particular measures of performances of land use by airports accommodating given demand can ultimately be as follows:
Operational performances
Capacity of land use = f (number of runways)
Intensity of land use = f (capacity of land use; number of runways)
Number of runways = f (area of land taken)
Economic performances
Airport profit/employment/contribution to GDP = f (accommodated passenger and cargo demand)
Accommodated demand = f (capacity)
Capacity = f (number of runways)
Number of runways = f (area of land taken)
Social performances
Exposure to noise / third-party risk = f (number of aircraft operations)
Number of aircraft operations = f (capacity)
Capacity = f (number of runways)
Number of runways = f (area of land taken)
Environmental performances
Energy/fuel consumption and emissions of GHG = f (number of aircraft operations)
Number of aircraft operations = f (capacity)
Capacity = f (number of runways)
Number of runways = f (area of land taken)
As can be noticed, all measures of each performance are actually directly or indirectly dependent on the area of land occupied by the given airport, and vice versa.
4. Application of the methodology
The proposed methodology for estimating performances of land use by airports is applied to illustrate its generosity and flexibility across different airports and the specific case airports as well, in the latter case, Amsterdam Schiphol (the Netherlands) and London Heathrow (UK).
4.1 Physical/spatial performances
4.1.1 Airport reference code and layout
shows the simplified layout (configuration) of Amsterdam Schiphol (the Netherlands) and London Heathrow airport (UK), which both belong to the highest FAA and ICAO design codes.
shows the simplified layout of Amsterdam Schiphol airport (AMS), which currently occupies 2,787 ha of land. The airport operates six runways, of which three are parallel and spaced sufficiently to enable their independent operation under instrumental meteorological conditions (ICM) and instrument flight rules (IFR). During the incoming peak demand, two of these runways are simultaneously used for landings and one for takeoffs. During the outgoing peak demand, the use of runways is the opposite—one is used for landings and two simultaneously for takeoffs. In addition, there are 195 aircraft parking stands. The landside area contains one passenger finger/pier-type terminal and seven air cargo terminals. The passenger terminal is surrounded by four runways, which enables balanced taxiing time after arrivals and before departures from any of these runways. However, because of its relative dislocation from the main runway system, the taxiing time to/from runway 18R/36L “Polder” can be substantial, sometimes even about 20–30 min. Currently the airport accommodates about 466,000 ATM and 46 million passengers per year (Janic, Citation2014).
shows a simplified scheme of London Heathrow airport (LHR), which is the largest airport in London and the United Kingdom and is one of the largest in the world. The airport occupies 1,227 ha of land. The airside area consists of two parallel runways operating in the segregated mode (one exclusively for landings and the other for takeoffs) due to noise constrains. The apron/gate complex contains 186 aircraft parking stands. The landside area contains five separate passenger terminals located between parallel runways. In addition to restrictions on the use of the existing pair of runways, additional land for expansion, i.e., building a new third parallel runway, is also restricted. However, if this (land) use restriction were lifted, a new (third) parallel runway taking about 260–300 ha of land could be built. Currently the airport accommodates about 475,000 ATMs and 67–68 million passengers per year (Center for Aviation, n.d.).
Additional evidence for the 30 largest airports worldwide in terms of the annual number of passengers (enplaned, deplaned, transit/transfer) indicates that they all occupy an area of land of about 58,150 ha, which is more or less equivalent to the land occupied by Singapore city. The average area of land taken by one of these airports is 1,938 ha (ACI, Citation2012; Wikipedia, n.d.).
4.2 Operational performances
4.2.1 Capacity and intensity of land use
show examples of the capacity and intensity of land use by airports.
shows that the capacity of land use based on the maximum number of ATMs carried out under IFR decreases more than proportionally with increasing of the area of land occupied by airports. In some sense, this indicates diseconomies of land use in light of the airport operational capacity. shows the actual intensity of land use across the world's 30 largest (and busiest) airports in 2012. As can again be seen, the intensity of land use expressed by the annual number of passengers accommodated per unit of occupied land generally decreases more than proportionally with increasing of the area of land. Both figures indicate that, in the given context, more sizeable (spacious) airports are generally less efficient users of land than their smaller counterparts.
shows an example of the capacity and intensity of land use at London Heathrow airport (the airport occupies a land area of 1,227 ha).
As can be seen, over the past 20 years (1991–2012), the capacity of land use has been constant due to the constrained land for the airport expansion and the cap imposed on the maximum annual number of ATMs (480,000) aimed to constrain the noise burden on the local population. The intensity of land use has changed over time in line with changing of the annual demand accommodated at the airport (ATMs). Since the intensity of land use has almost reached capacity, the latter will need to be increased either by changing the runway current operating mode from the segregated into mixed mode or by building a new (third) parallel runway. If an additional (third) parallel runway was built, the area of directly used new land would increase by an additional 250–300 ha, and the total area of land occupied by the airport would be about 1,477–1,527ha. Such an increase would contribute to increasing the runway system capacity by about 260,000 ATMs/year, to a total of 480,000 + 260,000 = 740,000 ATMs/year (i.e., by about 36%). Consequently, the capacity of land use would be 740,000 / 1,477 (1,527) = 501/485 ATMs/ha/year, which, compared to the present 391 ATMs/ha/year, is an increase of about 28% and 24%, respectively (Janic, Citation2010; Heathrow, Citationn.d.).
4.2.2 Effectiveness of land use
show examples of the effectiveness of land use at Amsterdam Schiphol airport.
shows that the effectiveness of land use expressed by the number of on-time ATMs/ha/year has slightly increased with increasing of the annual number of ATMs at an average annual rate of 0.227. At the same time, the intensity of land use expressed by the total number of ATMs/ha/year has also increased at a slightly higher annual rate of 0.354. The former relationship implies that the effectiveness of land use has slightly increased despite increasing of demand, mainly thanks to applying more sophisticated air traffic control/management tactical and operational measures. The latter relationship implies that the available airport land has been used more intensively, i.e., in line with increasing demand. Nevertheless, it still amounts to about 60–75% of the capacity of land use.
shows the average delay per ATM depending on the utilization of runway system capacity during each hour of a typical day of July 2011 under conditions when (i) additional land (about 260–300 ha) was taken for building the new (sixth) runway (Polder ban) in 2003; and (ii) this runway had not yet been built (Janic, Citation2014a). As can be seen, the average delay of an incoming and an outgoing flight has increased more than proportionally with increasing of the utilization of runway system capacity operating in the configuration (2+1 and 1+2, respectively) (with the new sixth runway) [see ]. However, if the runway system operated in the configuration 1+1 (i.e., if the Polder runway had not been built), the average delay of both incoming and outgoing flights under the given level of demand (June 2011) would dramatically increase with an average of 15–45 min and 15–35 min for each landing and taking-off flight, respectively. Such developments justify taking additional land for the new runway in the given case.
Figure 3. Simplified schemes of different airport land footprints (Horonjeff & McKelvey, Citation1994; Janic, Citation2013).
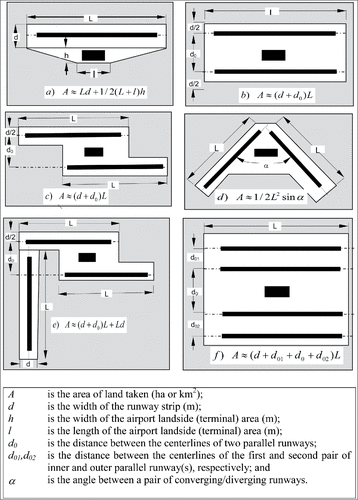
Figure 4. Simplified layouts of the selected (large) airports: (a) Amsterdam Schiphol airport (AMS), current layout, and (b) London Heathrow airport (LHR), current and prospective layouts (http://www.bbc.co.uk/news/uk-19570653).
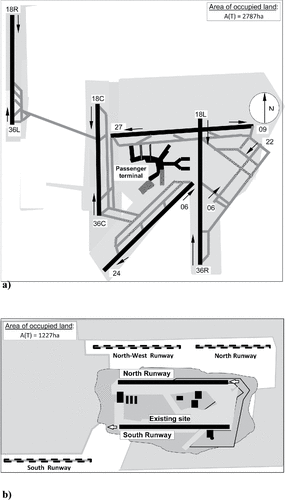
Figure 5. Examples of the capacity and intensity of land use by airports. (a) Capacity of land use vs. the area of land occupied by an airport (Horronjeff & Mckelvey, 1994; Janic, Citation2007). (b) Intensity of land use vs. the area of land occupied by an airport (case of 30 selected airports) (ACI, 2011; http://en.wikipedia.org/wiki/World's_busiest_airports_by_passenger_traffic).
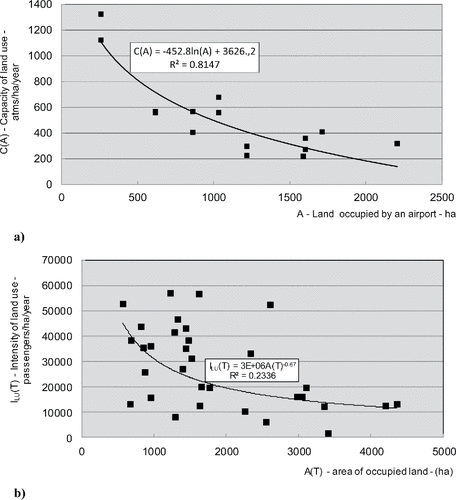
Figure 6. Capacity and intensity of land use at London Heathrow airport (LHR), UK, over time (http://www.heathrowairport.com).
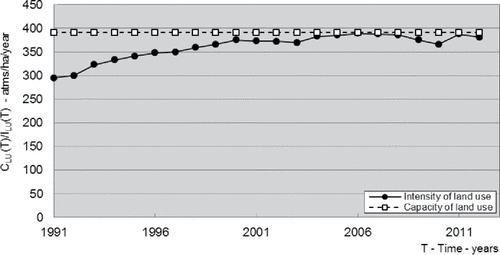
Figure 7. Effectiveness of land use at Amsterdam Schiphol airport (AMS), the Netherlands. (a) Effectiveness of land use vs. the annual number of ATMs (2001–2012) (http://www.schiphol.nl/SchipholGroup/AnnualReports.htm). (b) Average delay per ATM vs. utilization of the runway system capacity (Janic, Citation2014a).
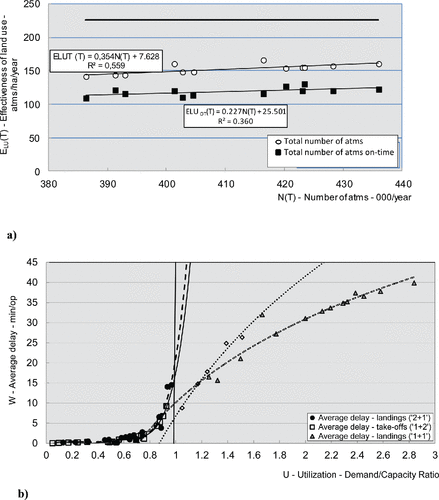
4.3 Economic performances
4.3.1 Economies of land use
show examples of the economics of land use at London Heathrow and Amsterdam Schiphol airports.
shows that the economies of land use have increased with increasing of the annual volumes of accommodated demand (WLUs) at both airports. The main reason is increased charges for aeronautical services and managing the related costs. In addition, the annual volumes of accommodated demand and corresponding economies of land use are about two times higher at Heathrow than at Schiphol airport. shows the development of the economies of land use over time at both airports. As can be seen, the dynamism has been similar mainly because they both have been affected by similar local and global impacts. At the same time, it has been higher at Heathrow than at Schiphol airport during the observed period. One of the main reasons for this seems to be that the latter airport occupies about twice the area of land of the former.
Figure 8. Economics of land use at the given airports: Amsterdam Schiphol (AMS), the Netherlands, and London Heathrow (LHR), UK. (a) Economies of land use vs. the volume of accommodated demand (2006–2012) (HAL, 2013; SG, 2013). (b) Economies of land use over time (2006–2012) (HAL, Citation2013; SG, Citation2013).
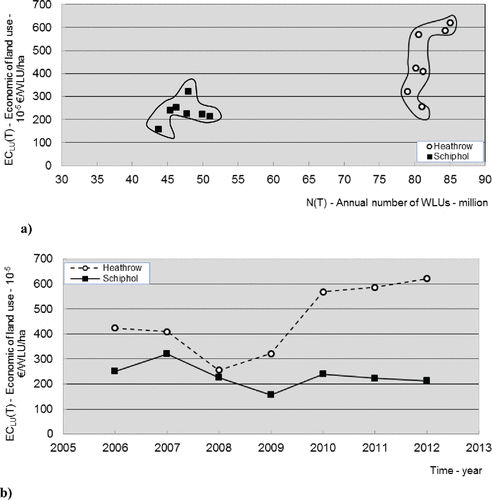
Figure 9. Relative losses of property values over time around the new (third) runway at London Heathrow airport (LHR) (EC, Citation2005; http://www.heathrowairport.com).
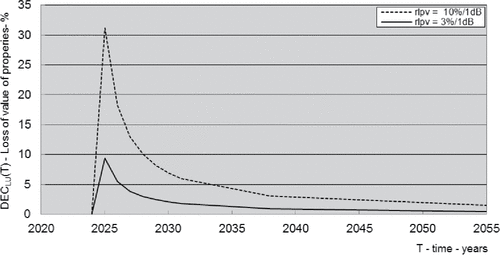
4.3.2 Diseconomies of land use
shows the example of diseconomies of land use for the case of building a new (third) runway at London Heathrow. The runway would be implemented in 2025. Over the next 30–35 years, i.e., by 2055–65, annual demand is expected to steadily grow up to the runway's full capacity of 260,000 ATMs/year. Consequently, the value of properties (houses) will be influenced by noise from these ATMs (EC, Citation2005).
As can be seen, under such conditions, the losses of property value during each year of the observed period (i.e., until the annual number of ATMs reaches the runway capacity) would be generally higher for the higher incremental increase in noise (1 dB). These losses would be the highest just after implementation of the new runway and then decrease more than proportionally over time. This indicates the diminishing of the marginal increase in noise with the increasing of the annual numbers of ATMs and the related total noise. Multiplying these relative values by the actual value of properties and their density enables estimation of the total losses in the value of properties per unit of area of occupied land.
4.4 Social performances
4.4.1 Intensity of noise exposure
show examples of the intensity of exposure to noise around London Heathrow airport.
Figure 10. Intensity of noise exposure vs. the annual number of ATMs at London Heathrow airport (LHR), UK (2001–2011). (a) Number of ATMs per exposed person within the 57Leq/16h noise contour. (b) Number of WLUs per exposed person within the 57Leq/16h noise contour. (c) Area of given noise contour and population.
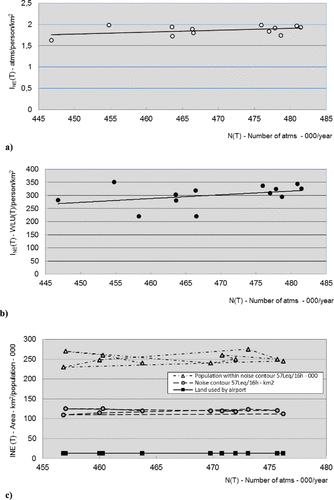
shows that the intensity of noise exposure (burden) per person by ATMs in the given example has slightly increased from 1.75 to 2.0 ATMs/person/km2 with increasing of the annual number of ATMs. shows a similar increase in the intensity of noise exposure with increasing annual volumes of WLUs—from about 275 to 350 WLUs/person/km2 (AC, Citation2013). Both relationships have seemingly been caused by the faster increase in the number of ATMs and WLUs (greater number of larger and fuller aircraft in operation) than the decrease in the area of given noise contour and the number of affected inhabitants within it. shows that the area of the 57Leq/16 noise contour is about 10 times greater than the land occupied by the airport itself, thus indicating that it should certainly be considered as the area of land actually occupied by the airport. The area of affected land has remained constant during the observed period (2001–2012) because the airport layout has not changed despite increasing ATM numbers. Furthermore, the population within the noise contour 57Leq/16h has also remained relatively constant—about 250,000 inhabitants—despite increased annual numbers of ATMs. These figures indicate how the intensity of noise exposure has remained relatively constant despite the growth in airport traffic. Obviously, noise-mitigating procedures in combination with the cap on the number of ATMs and use of less noisy aircraft have contributed to this.
4.4.2 Third-party risk from fatal aircraft accidents
shows examples of the third-party risk from a fatal aircraft accident within the area of the 57Leq/16h noise contour around London Heathrow [] and below the imaginary surfaces of the six runways of Amsterdam Schiphol airport [].
Figure 11. Third-party risks over time at London Heathrow and Amsterdam Schiphol airports (2001–2012) (EASA, Citation2013).
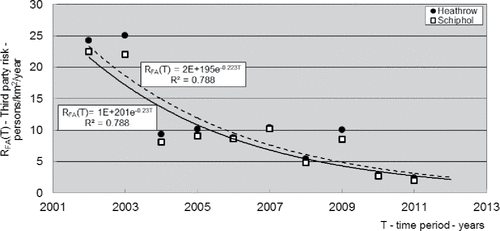
As can be seen, at both airports this risk has been diminishing more than proportionally during the observed period (2002–2012). The main reasons include the decreasing of (i) the overall rate of fatal aircraft accidents in Europe (from 2.5 to 0.5 per 10 million ATMs), (ii) the proportion of fatal aircraft accidents at and around European airports (from about 12.5% to 4.3%), and (iii) the density of population around both airports (all during the 2002–2012 period). The annual number of ATMs has changed more intensively at Schiphol (390–430,000) than at Heathrow airport (460–480,000) during the observed period. As such, both airports have become increasingly safer during the past decade regarding the exposure of third parties to the risk of loss of life due to a fatal aircraft accident.
4.5 Environmental performances
4.5.1 Intensity of energy consumption and emissions of GHG
shows an example of the intensity of energy consumption and related emissions of GHG at London Heathrow airport.
Figure 12. Intensity of fuel consumption and related emissions of GHG vs. the annual number of ATMs at London Heathrow airport, UK (2001–2012) (http://www.acl-uk.org/).
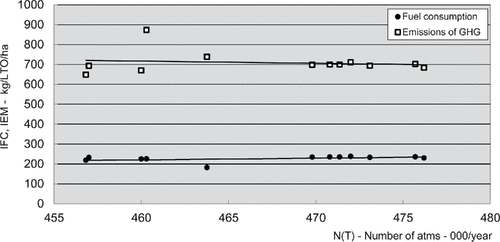
As can be seen, with increasing of the annual number of ATMs, the intensity of fuel consumption and related emissions of GHG has stabilized at the relatively constant level of about 250 and 700 tons / LTO cycle / ha, respectively, despite increasing volumes of accommodated demand on a constant area of land (1,227 ha) during the observed period. This indicates an improvement of carrying out the LTO cycles on the one hand and increased use of more fuel-efficient aircraft on the other.
4.6 Interrelationship of particular indicators and measures of performances
Based on the estimation and analysis of particular indicators and measures of performances of land use by airports, two approaches are used to explain their potential interrelationships shown in : the top-down and bottom-up approach.
4.6.1 Top-down approach
In general, indicators and measures of physical/spatial performances reflect the category of the given airport, its layout, area of land used, and the volumes of expected or existing traffic. Among other factors, the location and size of land used depend on the local land-use policy. Under such conditions, according to the top-down approach, the operational performances are considered to influence the other performances of land use by airports, and all them by traffic growth or stagnation. Thus, indicators and measures of the operational performances are influenced by traffic growth differently. For example, the capacity and intensity of land use improves, while the effectiveness deteriorates. When additional land is taken, for example, for a new runway, the two former indicators deteriorate and the latter improves, particularly if the traffic growth is not as immediate as expected. Operational performances influence the economic performances differently. Under current conditions, traffic growth contributes to improving economies and deterioration to diseconomies of land use. If additional land is taken, the economies of land use deteriorate and the diseconomies of land use improve, if traffic growth is not as immediate as expected. Quite the opposite happens when the traffic growth is as expected. However, improvement of particular indicators and measures of operational and consequently economic performances causes deterioration of all indicators and measures of social and economic performances. In summary, under conditions of traffic growth at the present or expanded configuration of the given airport, three indicators and measures of land use performance improve—capacity, intensity, and economies—and the other six—effectiveness, diseconomies, noise, traffic incidents/accidents, energy consumption and related emissions of GHG—deteriorate. In case of the lack of traffic growth, the influence on particular indicators and measures of performances is quite the opposite. The case of Amsterdam Schiphol airport represents an illustrative example of these relationships.
4.6.2 Bottom-up approach
According to the bottom-up approach, social or environmental performances usually influence the operational and economic performances. For example, limiting the noise exposure of the local population around the given airport improves or maintains under the prescribed limits the remaining measures of social and environmental performances, as well as diseconomies and effectiveness of land use. At the same time, by constraining traffic growth, it deteriorates the capacity, intensity, and economies of land use. In addition, limiting any other indicator and measure of social or environmental performances would produce a similar influence on the other indicators and measures of performances, all if the potential for traffic growth exists, which is in this case constrained. In this case additional land is also not taken simply because the traffic is kept within the prescribed limits. London Heathrow airport is a representative example of the described scenario.
Consequently, it can be said that according to the top-down approach, operational performances usually drive other performances with different influences on their particular indicators and measures under conditions of traffic growth or stagnation. According to the bottom-up approach, social or environmental performances drive the others, usually under conditions of constrained traffic growth under the prescribed limits. Expansion of the airports by, for example, adding a new runway and taking a relatively substantial area of land deteriorates particularly operational (capacity, intensity) and economic (economies and diseconomies) performances of land use, particularly if the expected traffic growth does not begin immediately after the runway implementation.
5. Conclusions
This article has described a methodology for analyzing, modeling, and assessing the performances of land use exclusively by aeronautical activities of airports. These relate to accommodating aircraft, passengers, and air cargo under given conditions. The methodology consists of indicators and measures of physical/spatial, operational, economic, environmental, and social performances of land use. The indicators and measures express the particular performances in relative terms, i.e., per unit of area of occupied land, which enables application to different airports and consequently their comparison. The methodology has been applied to illustrate its generosity and flexibility across different airports and to specific airports, in this case Amsterdam Schiphol (The Netherlands) and London Heathrow (UK) airports. Such application of the methodology has indicated that more spacious airports have generally experienced lower capacity and intensity of land use in terms of the accommodated ATMs (air transportation movements) and/or WLUs (workload units) per unit of occupied land, respectively. Constrained airports have increased the intensity of use of the already occupied land by increasing the volumes of accommodated traffic. The additional land taken for airport expansion has affected the intensity of land use, which has diminished and then again recovered as a result of traffic growth. The effectiveness of land use has shown to be compromised by constraining the taking of additional land for expansion of the airport airside (and landside) capacity.
The economies of land use mainly depend on the volumes and economic characteristics of demand (ATMs, passengers, WLUs) accommodated at the airport during the observed period. The diseconomies of land use expressed by the marginal losses of the value of properties exposed to airport noise diminish as the traffic volumes increase. The intensity of exposure to noise does not change if the area of land used does not change despite an increase in the volumes of accommodated demand. The third-party risk has decreased due to a reduction of the rate of fatal aircraft accidents and due to stagnating/decreasing population density around airports. This also applies to the intensity of fuel consumption and related emissions of GHG.
In addition to a qualitative analysis, an analysis of the potential relationships between particular performances of land use by airports has been carried out based on their estimated indicators and measures. This analysis has confirmed the basic findings from the qualitative analysis. In particular, it has also shown that according to the top-down approach, the operational performances of land use mainly influence other performances, albeit differently depending also on the traffic growth. According to the bottom-up approach, the social or environmental performances of land use usually influence other performances (again differently) in terms of constraints on traffic growth.
Future research could be carried out along two paths: the first is to further apply the proposed methodology to more airports in order to additionally verify its claimed generosity and flexibility; the other is to develop a complement to the existing methodology containing the indicators and measures of performances of land use by airport nonaeronautical activities. In such case it would be possible to establish relationships between the indicators and measures of performances of land use by both aeronautical and nonaeronautical activities of an airport. Consequently, this would enable estimation of the performances of land use by airport cities or aviapolises, which could be useful for a range of evaluation purposes at the local, regional, national, and international scale.
Notes
1 Despite the actual differences at particular airports, a LTO cycle has been standardized by ICAO in terms of four time-based components as follows: 0.7 min for takeoff, 2.2 min for climbing, 4.0 min for approach and landing, and 26.0 min for taxiing/idle phase of operation(s) (ICAO 1993).
2 The main GHGs are CO2 (carbon dioxide), NOx (mitrogen oxides), SO2 (sulfur dioxide), and H2O (water vapor).
References
- AC (Airports Commission). (2013). Aviation noise (Discussion Paper 5). London, UK: Author.
- ACI (Airport Council International). (2012). Annual traffic data. Retrieved from Airport Statistics and Data Centre, http://www.aci.aero/Data-Centre
- ACRP (Airport Cooperative Research Program). (2010). Enhancing airport land use compatibility. Volume 1: Land use fundamentals and implementation resources. Washington, DC: US Federal Aviation Administration.
- ALUC (Airport Land Use Commission for Orange County). (1995). Airport environs land use plan. Costa Mesa, CA: Author.
- Arron, R. W., & Stevens, J. N. (2008, 14–15 October). Airport city developments in Australia: Land use classification and analyses. Paper presented at the 10th TRAIL Congress and Knowledge Market, Rotterdam, the Netherlands.
- Baker, D., & Freestone, R. (2011). Spatial planning models of airport-driven urban development. Journal of Planning Literature, 26 (3), 263–279.
- Boeing. (2013). Statistical summary of commercial jet airplane accidents worldwide operations 1959–2012. Seattle, WA: Author.
- CAA (Civil Aviation Authority). (2012). Noise exposure contours for Heathrow Airport 2011 (Environmental Research and Consultancy Department Report 1201). London, UK: Directorate of Airspace Policy.
- CDT (California Department of Transportation, Caltrans). (2011, October). California airport land use planning handbook. Sacramento, CA: CDT Division of Aeronautics.
- Center for Aviation. (n.d.). Retrieved from http://centreforaviation.com
- Chang, S. J. (2006). Models of the relationship between transport and land use: A review. Transport Reviews, 26(3), 325–350.
- Cidell, L. J. 2004. Scales of airport expansion: Globalization, regionalization, and local land use. Minneapolis, MN: Center for Transportation Studies, Department of Geography.
- Goetz, R. A., & Szyliowicz, S. J. (1997). Revisiting transportation planning and decision making theory: The case of Denver International Airport. Transportation Research A, 31(4), 263–280.
- EASA (European Aviation Safety Agency). (2013). Annual safety review 2012. Cologne, Germany: EASA Safety Analysis and Research Department.
- EC (European Commission). (2005). ExternE: Externalities of energy, methodology 2005 updates. Luxembourg: Author.
- EC. (2011). Regulation of the European Parliament and of the council, on the establishment of rules and procedures with regard to the introduction of noise related operating restrictions at union airports within a balanced approach and repealing Directive 2002/30/EC of the European Parliament and of the council (COM(2011) 828 final). Brussels, Belgium: Author.
- Eriksen, K. S. (2000). Calculating external costs of transportation in Norway: Principles and results. European Journal of Transport and Infrastructure Research (EJTIR), 0(0), 9–25.
- FAA (Federal Aviation Administration). (2007). Airport master plan (Advisory Circular No. 150/5070-6BC). Washington, DC: Author.
- FDT (Florida Department of Transportation). (2012). Guidelines for determining market value and market rent of airport property. Tampa, FL: Centre for Urban Transportation Research, University of South Florida.
- Freestone, R., & Baker, D. (2010). Challenges in land use planning around Australian airports. Journal of Air Transport Management, 16(5), 264–271.
- Goetz, A. R., & Szyliowicz, J. S. (1997). Revisiting transportation planning and decision making: The case of Denver International Airport. Transportation Research Part A, 31(4), 263–280.
- HAL (Heathrow Airport Limited). (2013). Heathrow financial accounts. Hounslow, Middlesex, UK: Author.
- Heathrow. (n.d.) Retrieved from http://www.heathrowairport.com/
- Horonjeff, R., & McKelvey, F. X. (1994). Planning and design of airports, 4th ed. New York, NY: McGraw-Hill.
- ICAO (International Civil Aviation Organization). (1987). Airport planning manual, part 1: Master planning, 2nd ed. Montreal, Canada: Author.
- ICAO. (2002). Airport planning manual, part 2: Land use and environmental control, 3rd ed. Montreal, Canada: Author.
- Janic, M. (2007). The sustainability of air transportation: A quantitative analysis and assessment. Aldershot, UK: Ashgate.
- Janic, M. (2010). Developing an indicator system for monitoring, analyzing, and assessing airport sustainability. European Journal of Transport and Infrastructure Research, 3(10), 206–229.
- Janic, M. (2013). Airport analysis, planning, and design: Demand, capacity, and congestion. New York, NY: Nova.
- Janic, M. (2014a). Advanced transport systems: Analysis, modelling, and assessment of performances. London, UK: Springer.
- Janic, M. (2014b). Investigating utilization of the runway system capacity at large airports. Journal of Airport Management, 8(1), 71–88.
- Licitra, G., Gagliardi, P., Fredianelli, L., & Simonetti, D. (2014). Noise mitigation action plan of Pisa civil and military airport and its effects on people exposure. Applied Acoustic, 84, 25–36.
- Mackett, L. M. (1985). Integrated land use: Transport models. Transport Reviews, 5(4), 325–343.
- Ming Li, K., Eiff, G., Laffitte, J., & McDaniel, D. (2007). Land use management and airport controls trends and indicators of incompatible land use (Final Report). Cambridge, MA: Partnership for Air Transportation Noise and Emissions Reduction, Massachusetts Institute of Technology (MIT).
- Nelson, P. J. (2004). Meta-analysis of airport noise and hedonic property values: Problems and prospects. Journal of Transport Economics and Policy, 38(1), 1–28.
- PIA (Philadelphia International Airport). (2002). Land use assessment methodology: Appendix D (FAR Part 150 Noise Compatibility Study). Philadelphia, PA: Author.
- Quinton, A. (2012). What is an airport city? Retrieved from http://www.globalairportcities.com/airports-and-partners/what-is-an-airport-city
- Reiss, B. (2007). Maximizing non-aviation revenue for airports: Developing airport cities to optimise real estate and capitalise on land development opportunities. Journal of Airport Management, 1(3), 284–293.
- Sadr, K. M., Nassiri, P., Hosseini, M., Monavari, M., & Gharagozlou, A. (2014). Assessment of land use compatibility and noise pollution at Imam Khomeini International Airport. Journal of Air Transport Management, 34, 49–56.
- SG (Schiphol Group). (2013). Annual Results 2006–2012. Amsterdam, the Netherlands: Author.
- Scholl, B., & Nebel, R. (2014). Urban transformation in airport regions. disP – The Planning Review, 50(2), 65–75.
- Smith, M. J. T. (1989). Aircraft noise. Cambridge, UK: Cambridge University Press.
- Stevens, N., & Baker, D. (2013). Land use conflict across the airport fence: Competing urban policy, planning and priority in Australia. Urban Policy and Research, 31(3), 301–324.
- TRB (Transportation Research Board). (2010). Enhancing airport land use compatibility, Volume 1: Land use fundamentals and implementation resources (ACRP Report 27). Washington, DC: Author.
- Vogiatzis, K. (2012). Airport environmental noise mapping and land use management as an environmental protection action policy tool: The case of the Larnaka International Airport. Science of the Total Environment, 424, 162–173.
- Vogiatzis, K. (2014). Assessment of environmental noise due to aircraft operation at the Athens International Airport according to the 2002/49/EC directive and the new Greek national legislation. Applied Acoustics, 84, 37–46.
- Vogiatzis, K., & Remy, N. (2014). Strategic noise mapping of Heraklion: The aircraft noise impact as a factor of the international airport relocation. Noise Map, 1, 15–31.
- Wijnen, R. A. A., Walker, E. W., & Kwakkel, H. J. (2008). Decision support for airport strategic planning. Transportation Planning and Technology, 31(1), 11–34.
- Wikipedia. (n.d.). List of the world's busiest airports by passenger traffic. Retrieved from http://en.wikipedia.org/wiki/World's_busiest_airports_by_passenger_traffic
- WSDT (Washington State Department of Transportation). (1999). Airports and compatible land use, Volume 1: An introduction and overview for decision makers. Seattle, WA: Author.