ABSTRACT
Inflammation of adipose tissue is believed to be a contributing factor to many chronic diseases associated with obesity. Vitamin D (VD) is now known to limit this metabolic inflammation by decreasing inflammatory marker expression and leukocyte infiltration in adipose tissue. In this study, we investigated the impact of VD on microRNA (miR) expression in inflammatory conditions in human and mouse adipocytes, using high-throughput methodology (miRNA PCR arrays). Firstly, we identified three miRs (miR-146a, miR-150, and miR-155) positively regulated by TNFα in human adipocytes. Interestingly, the expression of these miRs was strongly prevented by 1,25(OH)2D preincubation. These results were partly confirmed in 3T3-L1 adipocytes (for miR-146a and miR-150). The ability of VD to control the expression of these miRs was confirmed in diet-induced obese mice: the levels of the three miRs were increased following high fat (HF) diet in epididymal white adipose tissue and reduced in HF diet fed mice supplemented with VD. The involvement of NF-κB signaling in the induction of these miRs was confirmed in vitro and in vivo using aP2-p65 transgenic mice. Finally, the ability of VD to deactivate NF-κB signaling, via p65 and IκB phosphorylation inhibition in murine adipocyte, was observed and could constitute a driving molecular mechanism. This study demonstrated for the first time that VD modulates the expression of miRs in adipocytes in vitro and in adipose tissue in vivo through its impact on NF-κB signaling pathway, which could represent a new mechanism of regulation of inflammation by VD.
Introduction
Adipose tissue was originally considered as a passive reservoir for energy storage, mechanical and heat insulation, and participating to the regulation of thermogenesis [Citation1]. Now, this tissue, and especially visceral adipose tissue, is also known to synthesize and secrete a large variety of pro-inflammatory and anti-inflammatory adipokines, cytokines, and chemokines [Citation2] that act at both autocrine/paracrine and endocrine level [Citation3].
Other markers such as microRNAs (miRs) have been associated with inflammatory response in adipose tissue during obesity [Citation4–6]. miRs are small noncoding RNAs that can modulate transcriptional networks, influencing most of biological processes. miRs regulate gene expression by degrading complementary mRNA targets or by inhibiting translation, and their function depends on the complementarity of sequence with the target mRNA [Citation7]. Several miRs are involved in adipocyte differentiation [Citation8], fat cell behavior [Citation9], and oxidative stress [Citation4]. The relevance of miRs in adipose tissue biology is increasingly recognized, being intrinsically linked to different pathways, including obesity-related inflammation. This is notably the case for miR-155, which was reported to be increased in inflamed adipocytes [Citation10], but also in subcutaneous adipose tissue biopsies of obese subjects [Citation11], and which displays a strong impact on the regulation of inflammatory tone and adipocyte differentiation [Citation11].
Similar to the increased pro-inflammatory markers, a low vitamin D (VD) status is common and well documented in obesity [Citation12]. Whether low VD status is the consequence of obesity, or whether this factor accelerates the development of obesity in humans is presently unknown. However, VD insufficiency could influence the development of obesity-related diseases, including inflammation and insulin resistance [Citation12–16]. Indeed, it was shown that 1,25(OH)2D significantly decreased the release of cytokines and chemokines by human preadipocytes [Citation17] and adipocytes [Citation18]. We also demonstrated the anti-inflammatory properties of 1,25(OH)2D in murine and human adipocytes, in both basal and TNFα-stimulated conditions [Citation19]. Similarly, 1,25(OH)2D reduced the expression of cytokines in human adipose tissue biopsies submitted to IL-1β stimulation in vitro [Citation20]. The molecular mechanisms have been investigated, and vitamin D receptor (VDR) and NF-κB signaling pathways and p38 MAP kinases were shown to be involved in 3T3-L1 adipocytes [Citation19] and in human adipocytes [Citation21]. Interestingly, Zoico et al. recently demonstrated that VD, similarly to 1,25(OH)2D, was able to blunt the LPS-mediated pro-inflammatory effect in human adipocytes [Citation22]. Using a microarray approach, we recently demonstrated that 1,25(OH)2D was able to downregulate a large set of chemokines in human and murine adipocytes, and consequently reduced macrophage migration mediated by adipocyte-conditioned medium [Citation23]. Finally, we demonstrated for the first time this effect in vivo, in acute and chronic models of adipose inflammation [Citation23].
In the present study, we aimed to explore the impact of VD on adipose inflammation associated miRs. To this end, we evaluated the impact of 1,25(OH)2D on miR expression in inflammatory conditions in human and mouse adipocytes, using a high-throughput methodology (miRNA PCR arrays). We also confirmed the anti-inflammatory effects of VD in vivo, in a chronic mice model of adipose tissue inflammation, and identified molecular mechanisms involved in miR regulation by VD.
Results
1,25(OH)2D modulates microRNA expression in human and murine adipocytes
To examine, in detail, the impact of 1,25(OH)2D on the modulation of miRs in human adipocytes submitted to inflammatory stress, human adipocytes primary cell cultures were preincubated with or without 1,25(OH)2D for 24 h followed by incubation with TNFα. Using a miRNA PCR array approach, we quantified 84 miRs and 6 housekeeping genes. Analysis of the results showed that 76 miRs were expressed in adipocytes in basal condition. Among these miRs, only 3 were positively regulated by TNFα in our conditions. As shown in , the inflammatory response mediated by TNFα significantly increased the expression levels of miR-146a, miR-150, and miR-155 (4.3-, 1.9-, and 2.1-fold, respectively; ). Interestingly, their expression in adipocytes preincubated with 1,25(OH)2D in inflammatory conditions only increased by 1.8-, 0.9-, and 1.3-fold, respectively, compared with control condition ().
Figure 1. 1,25(OH)2D limits microRNA expression levels in human adipocytes. Human adipocytes were preincubated with 1,25(OH)2D (100 nM) for 24 h followed by a second 24-h incubation with TNFα (15 ng/mL). miR expression levels were quantified through miRNA PCR arrays approach. SNORD68 was used as the endogenous control. The values are presented as means ± SEM. Bars not sharing the same letters are significantly different (P < 0.05). Experiments were performed in triplicate, at least 3 independent times.
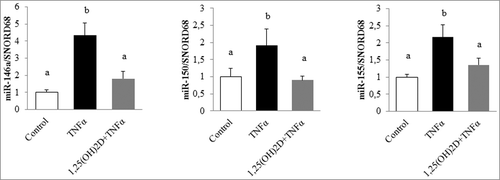
Our results were partly confirmed in 3T3-L1 adipocytes, where the levels of miR-146a and miR-155 increased in TNFα-treated cells (by 6.7- and 8-fold, respectively) and in the adipocytes pretreated with 1,25(OH)2D and incubated with TNFα (by 2.1- and 6.5-fold, respectively; ). No effect on miR-150 was observed in this cell model.
Figure 2. 1,25(OH)2D limits microRNA expression levels in murine adipocytes. 3T3-L1 adipocytes were preincubated with 1,25(OH)2D (100 nM) for 24 h followed by a second 24-h incubation with TNFα (15 ng/mL). Expression levels of miR-146a and miR-155 were quantified by qPCR in 3T3-L1 adipocytes. SNORD68 was used as the endogenous control. Values are presented as means ± SEM. Bars not sharing the same letters are significantly different (P < 0.05). Experiments were performed in triplicate, at least 3 independent times.
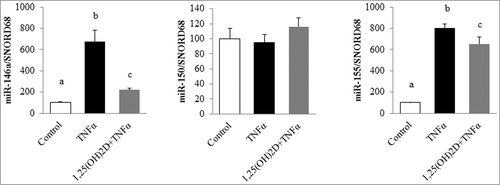
Vitamin D3 supplementation limits microRNA expression in epididymal white adipose tissue
To further examine the effect of VD on miR expression in vivo, a model of chronic inflammation induced by a high-fat (HF) diet was used. In this preclinical model, the induction of miR-146a, miR-150, and miR-155 levels was observed after 10 weeks of HF diet (by 2.4-, 1.4- and 1.5-fold, respectively) compared with control mice (). Interestingly, VD supplementation for 10 weeks strongly limited the expression of these three miRs in epididymal adipose tissue (1.1-, 1.1-, and 1.1-fold, respectively; ).
Figure 3. Vitamin D limits miR-146a, miR-150, and miR-155 expression in mice epididymal adipose tissue. The expression levels of miR-146a, miR-150, and miR-155 were quantified through qPCR in epididymal adipose tissue of mice fed standard, high fat (HF) diet or VD-supplemented HF diets for 10 weeks (n = 10) and expressed relative to SNORD68 in the white adipose tissue. Data are expressed as relative expression ratios. Values are presented as means ± SEM. Bars not sharing the same letters are significantly different (P < 0.05).
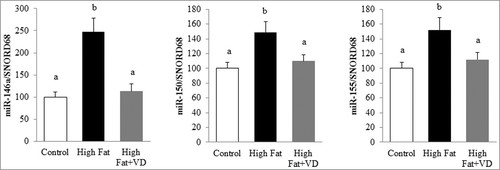
1,25(OH)2D limits microRNA expression through NF-κB signaling in 3T3-L1 adipocytes
To identify signaling pathways involved in miR-146a and miR-155 regulation by TNFα, 3T3-L1 cells were incubated with specific inhibitors of MAP kinases (JNK, p38) and NF-κB signaling, and then stimulated or not with TNFα. NF-κB signaling inhibition resulted in a significant decrease in miR-146a and miR-155 expression (by 2.7- and 1.6-fold compared with TNFα condition; A), whereas no effects of JNK and p38 inhibitors were observed. To confirm the role of NF-κB in miR-146a and miR-155 regulation in vivo, we used a transgenic model overexpressing NF-κB p65 subunit in adipose tissue (aP2-p65 mice [Citation24]). Results showed that miR-146a and miR-155 were significantly increased in epididymal adipose tissue of aP2-p65 mice compared with controls (by 2.2- and 10-fold, respectively; B). All together, these data strongly support the specific role of NF-κB on the regulation of these two miRs.
Figure 4. 1,25(OH)2D limits NF-κB activation. A. 3T3-L1 cells were treated with specific inhibitors of MAP kinases (JNK, p38) or NF-κB signaling [(JNK inhibitor II (10 µM), SB 202190 (20 µM) and BAY 117082 (10 µM), respectively] for 1 h and then stimulated with TNFα (15 ng/mL) for 24 h, and miR-146a and miR-155 expression were measured by qPCR. SNORD68 was used as the endogenous control. The data are expressed as relative expression ratios. Values are presented as means ± SEM. Bars not sharing the same letters are significantly different (P < 0.05). Experiments were performed in triplicate, at least 3 independent times. B. Expression of miR-146a and miR-155 were quantified by qPCR in epididymal adipose tissue of aP2-p65 transgenic mice. SNORD68 was used as the endogenous control. Data are expressed as relative expression ratios. The values are presented as means ± SEM. Bars not sharing the same letters are significantly different (P < 0.05). C. 3T3-L1 cells were preincubated with 1,25(OH)2D (10 and 100 nM) for 24 h in dose-dependent manner and incubated with TNFα (15 ng/mL) for 5 min. Phosphorylation levels of the NF-κB subunits (p65 and IκB) were evaluated using ELISA. Data are expressed as relative expression ratios. Values are presented as means ± SEM. Values not sharing the same letters are significantly different (P < 0.05). Experiments were performed in triplicate, at least 3 independent times.
![Figure 4. 1,25(OH)2D limits NF-κB activation. A. 3T3-L1 cells were treated with specific inhibitors of MAP kinases (JNK, p38) or NF-κB signaling [(JNK inhibitor II (10 µM), SB 202190 (20 µM) and BAY 117082 (10 µM), respectively] for 1 h and then stimulated with TNFα (15 ng/mL) for 24 h, and miR-146a and miR-155 expression were measured by qPCR. SNORD68 was used as the endogenous control. The data are expressed as relative expression ratios. Values are presented as means ± SEM. Bars not sharing the same letters are significantly different (P < 0.05). Experiments were performed in triplicate, at least 3 independent times. B. Expression of miR-146a and miR-155 were quantified by qPCR in epididymal adipose tissue of aP2-p65 transgenic mice. SNORD68 was used as the endogenous control. Data are expressed as relative expression ratios. The values are presented as means ± SEM. Bars not sharing the same letters are significantly different (P < 0.05). C. 3T3-L1 cells were preincubated with 1,25(OH)2D (10 and 100 nM) for 24 h in dose-dependent manner and incubated with TNFα (15 ng/mL) for 5 min. Phosphorylation levels of the NF-κB subunits (p65 and IκB) were evaluated using ELISA. Data are expressed as relative expression ratios. Values are presented as means ± SEM. Values not sharing the same letters are significantly different (P < 0.05). Experiments were performed in triplicate, at least 3 independent times.](/cms/asset/da82042b-f78b-43c2-bae9-6a74707b1899/kepi_a_1276681_f0004_b.gif)
Thus, we examined the impact on NF-κB signaling by 1,25(OH)2D preincubation (10 nM and 100 nM) for 24 hours followed by a 24-hour incubation with TNFα. To this end, the phosphorylation levels of p65 and IκB were quantified by ELISA. Expectedly, the phosphorylation levels of p65 and IκB were significantly increased into 3T3-L1 adipocytes incubated with TNFα (by 4.1- and 3.6-fold, respectively), whereas incubation with 1,25(OH)2D strongly limited the phosphorylation of p65 and IκB, suggesting that 1,25(OH)2D reduced NF-κB activation in 3T3-L1 adipocytes (C).
Discussion
Our results indicate that TNFα-mediated inflammation increased the production of miR-146a, miR-150, and miR-155 in adipocytes. We also reported a beneficial role of VD or its active form (1,25(OH)2D) on the expression of these miRs in human and murine adipocytes, but also in inflamed adipose tissue of mice. Molecular mechanisms for these effects were investigated and the NF-κB signaling pathway is suggested to be involved in miR regulation and to be targeted by 1,25(OH)2D. If the anti-inflammatory effect of VD has largely been reported based on cytokines and chemokines expression in adipocytes, as described in the introduction; its impact regarding its ability to modulate inflammatory-linked miRs has never been reported.
In this study, we identified miR-146a, miR-150, and miR-155 as adipocyte inflammatory stimulus modulated miR. These miRs have been largely suspected to be involved in inflammatory tone regulation; indeed, miR-155, which is described as a typical multifunctional microRNA [Citation25], has been associated with the regulation of different immune-related processes, such as hematopoiesis [Citation26], innate immunity [Citation27], cancer [Citation28], and B-cell and T-cell differentiation [Citation29]. In the context of obesity onset and/or obesity comorbidities, we have recently shown that miR-155 was induced in adipocytes submitted to inflammatory stress and in subcutaneous adipose tissue biopsies of obese subjects [Citation11]. We also showed that this miR induced inflammatory response, chemokine expression, and macrophage migration in 3T3-L1 adipocytes [Citation11]. A recent study also demonstrated that the deletion of miR-155 in female mice prevented diet-induced obesity, improved insulin sensitivity and energy uncoupling machinery, and abrogated HF diet-induced adipocyte hypertrophy and white adipose tissue inflammation [Citation30]. Together, these data confirm the importance of miR-155 in adipose tissue function, notably in the control of inflammation.
To the best of our knowledge, no specific effect of miR-146a in adipocyte or adipose tissue biology has been reported. However, in inflammatory context, it has been reported that miR-146a is involved in the control of innate immunity. Indeed, miR-146a was found to be inducible upon stimulation with LPS in a NF-κB-dependent manner in human monocytes [Citation31]. Such induction of miR-146a could contribute to the establishment of endotoxin tolerance in monocytes and to the regulation of TNFα production [Citation32]. In addition, exposure of human lung alveolar epithelial cells resulted in a pronounced increase of miR-146a levels, that in turn induced a decrease of IL-8 and RANTES chemokines [Citation33]. Finally, miR-146a deficiency during diabetes led to increased expression of M1 activation markers and pro-inflammatory cytokines and suppression of M2 markers in macrophages [Citation34]. Together, these data strongly suggest that miR-146a displays an anti-inflammatory effect. However, its role in adipose tissue will require further investigation.
Regarding miR-150, inflammatory or anti-inflammatory properties are less clear. A recent study demonstrated that miR-150 could physiologically modulate metabolic activities and inflammatory response both in cells and animals by regulating lipid metabolism and inflammatory response. Indeed, overexpression of miR-150 in macrophages resulted in an increase in lipid accumulation, associated with an elevated expression of several pro-inflammatory cytokines [Citation35]. Conversely, when miR-150 knockout mice were challenged with a HF diet, these mice presented reduced whole body weight with less fat accumulation, improved systemic glucose tolerance, and insulin sensitivity [Citation35]. In contrast, another study reported that miR-150 knockout mice showed exacerbated obesity-associated tissue inflammation and systemic insulin resistance [Citation36]. Such versatility has already been suggested in human cancer, where the role of miR-150 is context-dependent, i.e., this miR can have either oncogenic or tumor suppressor activity in cells that originate from different tissues [Citation37]. Thus, its specific role in adipose tissue and, notably, in the control of inflammatory tone will require further investigation.
To identify molecular mechanisms involved in miR regulation, several approaches were combined. First, we demonstrated that miR-146a and miR-155 regulations were primarily NF-κB-dependent in 3T3-L1 adipocytes, through the use of specific NF-κB inhibitor that blunted TNFα-mediated induction of these two miRs. The involvement of this signaling pathway was also confirmed in vivo through the use of aP2-p65 transgenic mice that display specific adipose tissue overexpression of p65 [Citation24] and that presented a strong induction of these two miRs. Based on this putative role of NF-κB in the induction of miR-146a and miR-155, the effect of the VD on the phosphorylation of two intermediates of the NF-κB signaling pathway (p65 and IκB) has been investigated. As previously demonstrated [Citation19,Citation23], we reported that 1,25(OH)2D has a strong limiting effect on NF-κB signaling in 3T3-L1 adipocytes through the reduction of the phosphorylation levels of p65 and IκB. This effect could be related to the ability of 1,25(OH)2D to bind VDR and to interact with IκB kinase (IKK) [Citation38] or could result from the induction of phosphatases involved in the dephosphorylation of p65 and IκB. All together these data strongly suggested that the inhibition of miRs by VD is largely mediated by its ability to reduce NF-κB signaling, which is consistent with data generated in macrophages [Citation39].
Interestingly, we observed both in vitro (in human and murine adipocytes) and in vivo (in mice) global upregulation of the miRs in pro-inflammatory conditions, suggesting that these three miRs, independently of their inflammatory effect (pro- or anti-), actively participated in inflammatory process in adipocytes and adipose tissue. We also observed that VD, through the downregulation of these three miRs, displayed an overall blocking effect of the inflammatory pathway in adipocytes. The modulation of miRs by VD is reported here for the first time in the context of obesity-associated comorbidities; similar ability has been widely described in cancer context. Indeed, differential regulation of miR expression profiles by 1,25(OH)2D has been demonstrated in prostate cancer cells [Citation40], bladder cancer cells [Citation41], and in lung cancer [Citation42].
These original observations not only reinforce the role of VD as an anti-inflammatory agent in adipose tissue and adipocytes, but also demonstrate the relevance of miR regulation as a new anti-inflammatory mechanism in adipose tissue/adipocytes, which could represent an interesting new target to limit metabolic inflammation.
Material and methods
Reagents
Dulbecco's modified Eagle's medium (DMEM) was purchased from Life Technologies (Cergy Pontoise, France); fetal bovine serum (FBS) was obtained from PAA Laboratories (Les Mureaux, France). Isobutylmethylxanthine, dexamethasone, and insulin were purchased from Sigma-Aldrich (Saint Quentin Fallavier, France). TRIzol reagent was obtained from Life Technologies (Saint Aubin, France). QuantiTect SYBR Green PCR Master Mix, miScript Universal Primer and miScript Primer assays were purchased from Qiagen (Courtabœuf, France).
Cell culture
Adipocyte cells were grown at 37°C in a 5% CO2 humidified atmosphere. The human preadipocytes (3 independent cultures) were obtained from Promocell and cultured with preadipocyte differentiation medium, according to the manufacturer's instructions. Mature adipocytes (day 12) were incubated with 1,25(OH)2D (100 nM, 24 h) followed by a 24-hour incubation with TNFα (15 ng/mL). Experiments were performed in triplicate, on 3 independent cultures.
The 3T3-L1 cells (ATCC, Manassas, VA) were seeded in 3.5 cm-diameter dishes at a density of 15 × 10<sp>4</sp> cells/well, and grown in DMEM supplemented with 10% FBS, at 37°C, as previously reported [Citation43]. To induce differentiation, two-day postconfluent 3T3-L1 preadipocytes (day 0) were stimulated for 72 h with 0.5 mM isobutylmethylxanthine, 0.25 µmol/L dexamethasone and 1 µg/mL insulin in DMEM supplemented with 10% FBS. The cultures were then treated with DMEM supplemented with 10% FBS and 1 µg/mL insulin. The mature adipocytes (day 8) were incubated with 1,25(OH)2D (100 nM, 24 h) followed by a 24-hour incubation with TNFα (15 ng/mL). Experiments were performed in triplicate, on 3 independent cultures.
3T3-L1 adipocytes incubations
To identify signaling pathways involved in microRNA regulation, 3T3-L1 cells were treated with specific inhibitors of mitogen activated protein (MAP) kinases (JNK, p38) and NF-κB signaling [JNK inhibitor II (10 µM), SB 202190 (20 µM) and BAY 117082 (10 µM), respectively] for 1 h (all obtained from Calbiochem, Merck Millipore, Darmstadt, Germany) and then stimulated or not with TNFα (15 ng/mL) for 24 h. All the treatments were performed on day 8.
NF-κB activation
The levels of p65 (Ser536) and IκBα (Ser32/36) phosphorylation were quantified using the ELISA Instant One kit according to the manufacturer's instructions (eBiosciences SAS, Paris, France).
miRNA PCR arrays
miRNA PCR arrays (Qiagen, Courtabœuf, France) were used to quantify miRs extracted from human culture adipocytes, according to the manufacturer's instructions. Reactions were performed in a 12.5 μL volume containing 6.25 μL 2X QuantiTect SYBR Green PCR Master Mix (Qiagen, Courtabœuf, France), 1.25 μL 10X miScript Universal Primer (Qiagen, Courtabœuf, France). After an initial incubation step of 15 min at 95°C, amplification reaction was performed in 40 cycles comprising 3 steps (94°C, 15 sec, 55°C, 30 sec, and 70°C, 30 sec). For each condition, the expression was quantified in duplicate and the SNORD68 was used as the endogenous control in the comparative cycle threshold (CT) method.
RNA isolation and qPCR
Total cellular RNA was extracted using TRIzol reagent, according to the manufacturer's instructions. To quantify miR-146a, miR-150, and miR-155, cDNAs were first synthesized from 1 µg of total RNA in 20 μL using 5X miScript Hiflex Buffer, 10X nucleic mix and miScript reverse transcriptase according to the manufacturer's instructions (Qiagen). Real-time quantitative RT-PCR analyses were performed using the Mx3005P Real-Time PCR System (Stratagene, La Jolla, CA), as previously described [Citation44]. Reactions were performed in a 12.5 μL volume containing 6.25 μL of 2X QuantiTect SYBR Green PCR Master Mix (Qiagen), 1.25 μL of 10X miScript Universal Primer (Qiagen), 1.25 μL of 10X miScript Primer Assay [Mm_miR146_1 miScript Primer Assay, Mm_miR-150_1 miScript Primer Assay, Mm_miR-155_1 miScript Primer Assay and Hs_SNORD68 miScript Primer Assay (Qiagen)] and 2.5 μL of RNase-free water. For each condition, the expression was quantified in duplicate, and the SNORD68 was used as the endogenous control in the comparative cycle threshold (CT) method.
Animal experiments
The protocol was approved through the local ethics committee. Six-week-old male C57BL/6J mice were obtained from Janvier (Le Genest Saint Isle, France). The mice were fed ad libitum (chow diet A04, Safe, Augy, France), with full access to drinking water. The animals were maintained at 22°C under a 12 h light: 12 h dark cycle with a 50% humidity level.
To assess the impact of vitamin D (cholecalciferol) on miRs expression, three experimental groups of mice were fed ad libitum with a low (10% of total energy as fat; TD06416; Harlan, Indianapolis, Indiana, USA) or HF diet, providing 45% of total energy as fat (TD06415, Harlan, Indianapolis, Indiana, USA), supplemented (3000 IU/kg of body weight, i.e., around 90 UI/j/mouse, Sigma-Aldrich, Saint Quentin Fallavier, France) or not (300 IU/kg of body weight, i.e., around 9 UI/j/mouse) with cholecalciferol. Mice (10 per group) were assigned into one of the three experimental groups depending on the diet, i.e., control, HF, and HF plus vitamin D (HFVD), for 10 weeks, as previously described [Citation45]. Epidydimal adipose tissue was collected and stored at -80°C.
aP2-p65 mice were generated on the C57BL/6J background, as described elsewhere [Citation24,Citation46]. Mice were housed in the animal facility at the Pennington Biomedical Research Center with a 12:12-h light-dark cycle and constant temperature (22-24°C). Male mice were fed with a chow diet (MF 5001, 11% calorie in fat) and the epididymal fat tissue was collected at 20 weeks. The mice were housed at four per cage with free access to water and diet.
Statistical analysis
Data are expressed as mean ± SEM. Significant differences between the control and treated group were determined using ANOVA, followed by the PLSD Fischer post hoc test using Statview software, and P < 0.05 was considered statistically significant. Significant differences between two groups were determined using t test.
Disclosure of potential conflicts of interest
No potential conflicts of interest were disclosed.
Acknowledgments
This work was partially funded through grants from the Fondation Coeur et Artères (FCA).
References
- Trayhurn P, Beattie JH. Physiological role of adipose tissue: white adipose tissue as an endocrine and secretory organ. Proc Nutr Soc. 2001;60:329–339. doi:10.1079/PNS200194. PMID:11681807
- Gregor MF, Hotamisligil GS. Inflammatory mechanisms in obesity. Annu Rev Immunol. 2011;29:415–445. doi:10.1146/annurev-immunol-031210-101322. PMID:21219177
- Proenca AR, Sertie RA, Oliveira AC, et al. New concepts in white adipose tissue physiology. Braz J Med Biol Res. 2014. doi:10.1590/1414-431X20132911. PMID:24604427
- Arner P, Kulyte A. MicroRNA regulatory networks in human adipose tissue and obesity. Nat Rev Endocrinol. 2015;11:276–288. doi:10.1038/nrendo.2015.25. PMID:25732520
- Ge Q, Brichard S, Yi X, et al. microRNAs as a new mechanism regulating adipose tissue inflammation in obesity and as a novel therapeutic strategy in the metabolic syndrome. J Immunol Res. 2014;2014:987285. doi:10.1155/2014/987285. PMID:24741638
- Hulsmans M, De Keyzer D, Holvoet P. MicroRNAs regulating oxidative stress and inflammation in relation to obesity and atherosclerosis. Faseb J. 2011;25:2515–2527. doi:10.1096/fj.11-181149. PMID:21507901
- Krol J, Loedige I, Filipowicz W. The widespread regulation of microRNA biogenesis, function and decay. Nat Rev Genet. 2010;11:597–610. doi:10.1038/nrg2843. PMID:20661255
- Ortega FJ, Moreno-Navarrete JM, Pardo G, et al. MiRNA expression profile of human subcutaneous adipose and during adipocyte differentiation. PLoS One. 2010;5:e9022. doi:10.1371/journal.pone.0009022. PMID:20126310
- Oger F, Gheeraert C, Mogilenko D, et al. Cell-specific dysregulation of microRNA expression in obese white adipose tissue. J Clin Endocrinol Metab. 2014;99:2821–2833. doi:10.1210/jc.2013-4259. PMID:24758184
- Ortega FJ, Moreno M, Mercader JM, et al. Inflammation triggers specific microRNA profiles in human adipocytes and macrophages and in their supernatants. Clin Epigenetics. 2015;7:49. doi:10.1186/s13148-015-0083-3. PMID:25926893
- Karkeni E, Astier J, Tourniaire F, et al. Obesity-associated inflammation induces microRNA-155 expression in adipocytes and adipose tissue: outcome on adipocyte function. J Clin Endocrinol Metab. 2016;101:1615–1626. doi:10.1210/jc.2015-3410. PMID:26829440
- Landrier JF, Karkeni E, Marcotorchino J, et al. Vitamin D modulates adipose tissue biology: possible consequences for obesity? Proc Nutr Soc. 2016;75:38–46. doi:10.1017/S0029665115004164. PMID:26564334
- Garcia OP, Long KZ, Rosado JL. Impact of micronutrient deficiencies on obesity. Nutr Rev. 2009;67:559–572. doi:10.1111/j.1753-4887.2009.00228.x. PMID:19785688
- Kaidar-Person O, Person B, Szomstein S, et al. Nutritional deficiencies in morbidly obese patients: a new form of malnutrition? Part A: vitamins. Obes Surg. 2008;18:870–876. doi:10.1007/s11695-007-9349-y. PMID:18465178
- Marcotorchino J, Tourniaire F, Landrier JF. Vitamin D, adipose tissue, and obesity. Horm Mol Biol Clin Investig. 2013;15:123–128. doi:10.1515/hmbci-2013-0027. PMID:25436739
- Mezza T, Muscogiuri G, Sorice GP, et al. Vitamin D deficiency: a new risk factor for type 2 diabetes? Ann Nutr Metab. 2012;61:337–348. doi:10.1159/000342771. PMID:23208163
- Gao D, Trayhurn P, Bing C. 1,25-Dihydroxyvitamin D3 inhibits the cytokine-induced secretion of MCP-1 and reduces monocyte recruitment by human preadipocytes. Int J Obes (Lond). 2012;37:357–365. doi:10.1038/ijo.2012.53. PMID:22508334
- Lorente-Cebrian S, Eriksson A, Dunlop T, et al. Differential effects of 1alpha,25-dihydroxycholecalciferol on MCP-1 and adiponectin production in human white adipocytes. Eur J Nutr. 2012;51:335–342. doi:10.1007/s00394-011-0218-z. PMID:21701898
- Marcotorchino J, Gouranton E, Romier B, et al. Vitamin D reduces the inflammatory response and restores glucose uptake in adipocytes. Mol Nutr Food Res. 2012;56:1771–1782. doi:10.1002/mnfr.201200383. PMID:23065818
- Wamberg L, Cullberg KB, Rejnmark L, et al. Investigations of the anti-inflammatory effects of vitamin D in adipose tissue: results from an in vitro study and a randomized controlled trial. Horm Metab Res. 2013;45:456–462. doi:10.1055/s-0032-1331746. PMID:23322512
- Mutt SJ, Karhu T, Lehtonen S, et al. Inhibition of cytokine secretion from adipocytes by 1,25-dihydroxyvitamin D(3) via the NF-kappaB pathway. Faseb J. 2012;26:4400–4407. doi:10.1096/fj.12-210880. PMID:22798425
- Zoico E, Franceschetti G, Chirumbolo S, et al. Phenotypic shift of adipocytes by cholecalciferol and 1alpha,25 dihydroxycholecalciferol in relation to inflammatory status and calcium content. Endocrinology. 2014;155:4178–4188. doi:10.1210/en.2013-1969. PMID:25153146
- Karkeni E, Marcotorchino J, Tourniaire F, et al. Vitamin D limits chemokine expression in adipocytes and macrophage migration in vitro and in male mice. Endocrinology. 2015;156:1782–1793. doi:10.1210/en.2014-1647. PMID:25730105
- Tang T, Zhang J, Yin J, et al. Uncoupling of inflammation and insulin resistance by NF-kappaB in transgenic mice through elevated energy expenditure. J Biol Chem. 2010;285:4637–4644. doi:10.1074/jbc.M109.068007. PMID:20018865
- Faraoni I, Antonetti FR, Cardone J, et al. miR-155 gene: a typical multifunctional microRNA. Biochim Biophys Acta. 2009;1792:497–505. doi:10.1016/j.bbadis.2009.02.013. PMID:19268705
- Masaki S, Ohtsuka R, Abe Y, et al. Expression patterns of microRNAs 155 and 451 during normal human erythropoiesis. Biochem Biophys Res Commun. 2007;364:509–514. doi:10.1016/j.bbrc.2007.10.077. PMID:17964546
- O'Connell RM, Taganov KD, Boldin MP, et al. MicroRNA-155 is induced during the macrophage inflammatory response. Proc Natl Acad Sci U S A. 2007;104:1604–1609. doi:10.1073/pnas.0610731104. PMID:17242365
- Gironella M, Seux M, Xie MJ, et al. Tumor protein 53-induced nuclear protein 1 expression is repressed by miR-155, and its restoration inhibits pancreatic tumor development. Proc Natl Acad Sci U S A. 2007;104:16170–16175. doi:10.1073/pnas.0703942104. PMID:17911264
- Kluiver J, Poppema S, de Jong D, et al. BIC and miR-155 are highly expressed in Hodgkin, primary mediastinal and diffuse large B cell lymphomas. J Pathol. 2005;207:243–249. doi:10.1002/path.1825. PMID:16041695
- Gaudet AD, Fonken LK, Gushchina LV, et al. miR-155 Deletion in Female Mice Prevents Diet-Induced Obesity. Sci Rep. 2016;6:22862. doi:10.1038/srep22862. PMID:26953132
- Taganov KD, Boldin MP, Chang KJ, et al. NF-kappaB-dependent induction of microRNA miR-146, an inhibitor targeted to signaling proteins of innate immune responses. Proc Natl Acad Sci U S A. 2006;103:12481–12486. doi:10.1073/pnas.0605298103. PMID:16885212
- Nahid MA, Pauley KM, Satoh M, et al. miR-146a is critical for endotoxin-induced tolerance: IMPLICATION IN INNATE IMMUNITY. J Biol Chem. 2009;284:34590–34599. doi:10.1074/jbc.M109.056317. PMID:19840932
- Perry MM, Williams AE, Tsitsiou E, et al. Divergent intracellular pathways regulate interleukin-1beta-induced miR-146a and miR-146b expression and chemokine release in human alveolar epithelial cells. FEBS Lett. 2009;583:3349–3355. doi:10.1016/j.febslet.2009.09.038. PMID:19786024
- Bhatt K, Lanting LL, Jia Y, et al. Anti-Inflammatory role of MicroRNA-146a in the pathogenesis of diabetic nephropathy. J Am Soc Nephrol. 2016;27:2277–2288. doi:10.1681/ASN.2015010111. PMID:26647423
- Luo N, Garvey WT, Wang D-Z, et al. MicroRNA-150 regulates lipid metabolism and inflammatory response. J Metabolic Synd. 2013;2:131. doi:10.4172/2167-0943.1000131
- Ying W, Tseng A, Chang RC, et al. miR-150 regulates obesity-associated insulin resistance by controlling B cell functions. Sci Rep. 2016;6:20176. doi:10.1038/srep20176. PMID:26833392
- Moles R, Bellon M, Nicot C. STAT1: A Novel Target of miR-150 and miR-223 Is Involved in the Proliferation of HTLV-I-Transformed and ATL Cells. Neoplasia. 2015;17:449–462. doi:10.1016/j.neo.2015.04.005. PMID:26025667
- Chen Y, Zhang J, Ge X, et al. Vitamin D receptor inhibits nuclear factor kappaB activation by interacting with IkappaB kinase beta protein. J Biol Chem. 2013;288:19450–19458. doi:10.1074/jbc.M113.467670. PMID:23671281
- Chen Y, Liu W, Sun T, et al. 1,25-Dihydroxyvitamin D promotes negative feedback regulation of TLR signaling via targeting microRNA-155-SOCS1 in macrophages. J Immunol. 2013;190:3687–95. doi:10.4049/jimmunol.1203273. PMID:23436936
- Kutmon M, Coort SL, de Nooijer K, et al. Integrative network-based analysis of mRNA and microRNA expression in 1,25-dihydroxyvitamin D3-treated cancer cells. Genes Nutr. 2015;10:484. doi:10.1007/s12263-015-0484-0.
- Ma Y, Hu Q, Luo W, et al. 1alpha,25(OH)2D3 differentially regulates miRNA expression in human bladder cancer cells. J Steroid Biochem Mol Biol. 2015;148:166–171. doi:10.1016/j.jsbmb.2014.09.020. PMID:25263658
- Guan H, Liu C, Chen Z, et al. 1,25-Dihydroxyvitamin D3 up-regulates expression of hsa-let-7a-2 through the interaction of VDR/VDRE in human lung cancer A549 cells. Gene. 2013;522:142–146. doi:10.1016/j.gene.2013.03.065. PMID:23566834
- Landrier JF, Gouranton E, El Yazidi C, et al. Adiponectin expression is induced by vitamin E via a peroxisome proliferator-activated receptor gamma-dependent mechanism. Endocrinology. 2009;150:5318–5325. doi:10.1210/en.2009-0506. PMID:19833717
- Landrier JF, Malezet-Desmoulins C, Reboul E, et al. Comparison of different vehicles to study the effect of tocopherols on gene expression in intestinal cells. Free Radic Res. 2008;42:523–530. doi:10.1080/10715760802098859. PMID:18484416
- Marcotorchino J, Tourniaire F, Astier J, et al. Vitamin D protects against diet-induced obesity by enhancing fatty acid oxidation. J Nutr Biochem. 2014;25:1077–1083. doi:10.1016/j.jnutbio.2014.05.010. PMID:25052163
- Tourniaire F, Romier-Crouzet B, Lee JH, et al. Chemokine expression in inflamed adipose tissue is mainly mediated by NF-kappaB. PLoS One. 2013;8:e66515. doi:10.1371/journal.pone.0066515. PMID:23824685