ABSTRACT
Obsessive-compulsive disorder (OCD) has recently been linked to increased methylation levels in the oxytocin receptor (OXTR) gene, and OXTR hypermethylation has predicted a worse treatment response to cognitive-behavioural therapy (CBT). Furthermore, OCD is associated with childhood trauma and stressful life events, which have both been shown to affect OXTR methylation. Here, we aimed to replicate findings of increased OXTR methylation as a predictor of disease and worse treatment response in an independent sample that received treatment within the public health care system. In addition, we aimed to extend previous findings by examining associations between OXTR hypermethylation, environmental stressors, OCD diagnosis, and treatment response. Methylation levels at two CpGs within OXTR exon III were compared between n = 181 OCD patients and n = 199 healthy controls using linear regression analysis. In a subsample of OCD patients (n = 98) with documented treatment data, we examined associations between methylation and treatment response to CBT. Childhood adversity and stressful life events were assessed using Childhood Trauma Questionnaire and Life Experience Survey, respectively. OCD patients exhibited significant hypermethylation at CpG site cg04523291 compared to controls, and increased methylation was associated with impaired treatment response. Moreover, hypermethylation at cg04523291 was associated with stressful life events in OCD patients, and with childhood adversity in controls. Yet, there were no significant mediation effects. In conclusion, we replicated the association between OXTR hypermethylation and OCD in the largest sample, so far. Furthermore, our findings support the role of OXTR methylation as a promising biomarker for treatment response in OCD.
Introduction
Obsessive-compulsive disorder (OCD) is a debilitating and often chronic psychiatric disorder characterized by obsessions (intrusive unwanted thoughts or images) and/or compulsions (ritualized repetitive behaviours or mental acts), which affects 1–3% of the population worldwide [Citation1]. Multiple causes contribute to the aetiology of OCD, including both genetic and environmental risk factors, such as childhood adversity and stressful life events [Citation2]. First-line treatment for OCD comprises cognitive-behavioural therapy (CBT) with exposure and response prevention (ERP) as well as medication with selective serotonin reuptake inhibitors (SSRI). Despite general effectiveness of this treatment, as much as 40–60% of patients report residual symptoms [Citation3,Citation4], stressing the necessity to identify predictive treatment response markers that could potentially guide clinical decision-making towards more personalized and thus more effective therapeutic interventions [Citation5].
Recent evidence supports the role of DNA methylation as a candidate biomarker for treatment response in OCD. DNA methylation is an epigenetic mechanism that affects gene expression and can thus act as a mediator between environmental factors and functional changes at biochemical levels, which may eventually contribute to the manifestation of clinical phenotypes. Specifically, Schiele et al. found that OCD patients exhibited increased methylation in exon III of the oxytocin receptor gene (OXTR) compared to healthy controls, and that hypermethylation of this gene region was associated with impaired CBT treatment response [Citation5]. Further supporting the role of epigenetic changes in OXTR, increased OCD symptom severity has been linked to OXTR exon III hypermethylation [Citation6], and reduced methylation in the untranslated region (UTR) of OXTR exon II has been found in OCD patients compared to controls [Citation7].
Oxytocin is a neuropeptide that regulates social behaviour by increasing overt and covert attention for social signals [Citation8]. It acts via oxytocin receptors, which are highly expressed in brain regions implicated in the processing of social and affective stimuli, such as the amygdala, insula, and striatum [Citation9]. Notably, neurobiological alterations in these brain regions, most prominently the striatum, have also been observed in patients with OCD [Citation2]. In rats, oxytocin injection into the central nucleus of the amygdala has been shown to induce hypergrooming, which is assumed to reflect OCD-related behaviour [Citation10]. In the same line, OCD patients exhibit increased levels of oxytocin in cerebrospinal fluid and blood plasma compared to healthy controls [Citation11,Citation12].
Mounting evidence suggests that childhood trauma and other stressful life events have a significant effect on the onset, development, progression, and severity of obsessive-compulsive symptoms [Citation13–18]. Furthermore, a higher number of negative life events in the past year and a higher number of different categories of adverse childhood experiences have been linked to an increased risk of entering a chronic course of illness in OCD [Citation18]. A history of trauma has also been associated with greater treatment resistance in OCD [Citation19].
So far, it has not been examined systematically whether environmental risk factors impact OXTR methylation in OCD and thus drive associations with symptom severity and treatment response. However, recent evidence highlights the association between OXTR methylation and childhood trauma. Participants who received low maternal care exhibited hypermethylation at eight CpGs in OXTR exon III [Citation20], and increased levels of early life adversity have been linked to hypermethylation at one CpG site in the first intron of OXTR in females [Citation21]. Moreover, maltreated children had increased methylation levels at two OXTR CpG sites as well as lower grey matter volume in the left orbitofrontal cortex (OFC) [Citation22], which has robustly been implicated in the pathophysiology of OCD [Citation23]. Further supporting the notion that epigenetic modulation of the oxytocin system represents a potential pathomechanism of psychiatric disease, alterations in OXTR methylation have been observed in patients with post-traumatic stress disorder (PTSD), depression, autism, social anxiety disorder, and anorexia [Citation24,Citation25].
In the present study, we investigate the role of OXTR exon III methylation in OCD via four different approaches. First, we examine methylation differences in the so far largest sample of cases and controls; second, we aim to replicate previous findings of OXTR hypermethylation as a predictor of CBT treatment response in a naturalistic outpatient setting within the public health care system; third, we explore associations between OXTR methylation, childhood trauma, and recent stressful life events; and fourth, we test whether alterations in OXTR methylation mediate the association between environmental risk factors and OCD caseness.
Materials and methods
Sample and clinical assessment
The full sample consisted of 181 patients with OCD and 199 healthy comparison subjects, who participated in the EPOC (Endophenotypes of OCD) study [Citation26–28]. Sample characteristics are presented in . OCD patients were recruited via the outpatient clinics at the Department of Psychology of Humboldt-Universität zu Berlin and at the Department of Psychiatry and Psychotherapy of the University Hospital Bonn, Germany. Healthy volunteers were recruited from the general population via public advertisements. All the participants were examined by trained clinical psychologists using the Structured Clinical Interview for DSM-IV (SCID-I) [Citation29,Citation30]. To establish cross-site reliability of clinical ratings, all instructions were standardized and raters completed assessments of four training videos. Patients were only included if they: (a) were free of past or present psychotic, bipolar, or substance-related disorders; (b) did not take neuroleptic medication for the previous 4 weeks; and (c) did not use benzodiazepines in the prior 2 weeks. Additionally, healthy controls were excluded if they: (a) took any psychoactive medication in the previous 3 months; (b) had a current Axis I disorder; (c) had a lifetime diagnosis of OCD or tic disorder; or (d) had a family history of OCD. All subjects were from European ancestry, and cases and controls did not differ with respect to population structure.
Table 1. Demographic and clinical characteristics of OCD patients and healthy controls
Written informed consent was obtained, and participants were monetarily compensated for their time. The study was in accordance with the revised Declaration of Helsinki and approved by the local ethics committees of the Department of Psychology, Humboldt-Universität zu Berlin, and the University Hospital Bonn.
Treatment subsample
A subsample of OCD patients who participated in the EPOC study completed individual CBT at a university outpatient unit at the Berlin study site (Hochschulambulanz für Psychotherapie und Psychodiagnostik der Humboldt-Universität zu Berlin). CBT was administered by licenced psychotherapists, who had completed at least 3 years of training in CBT. Treatments were bound to the general conditions for psychotherapy in the public German health care system. Therapists were instructed to apply CBT including ERP according to the national guideline for evidence-based treatment [Citation31]. Therapy sessions usually lasted 50 minutes and took place once or twice weekly, yet therapists were free to adjust session length when implementing exposure and to reduce session frequency at the end of treatment. Treatment was terminated by consensus between patient and therapist. In accordance with the naturalistic design of the present study, the number of completed treatment sessions varied between patients (M = 46.91, SD = 20.03, [8–98]).
For n = 98 patients, Y-BOCS data were available at pre- and post-treatment. Based on a criterion of 35% reduction in Y-BOCS scores from pre- to post-treatment [Citation4], n = 58 OCD patients were classified as responders, while n = 40 patients were classified as non-responders. One-year post-treatment follow-up status was assessed by telephone-based interviews for n = 40 patients.
In addition to CBT, roughly half of the patients received pharmacotherapy, predominantly with SSRI. At baseline assessment, i.e., pre-treatment, n = 40 OCD patients reported having taken psychotropic medication in the past 4 weeks, n = 17 patients reported having been medicated before, but not in the past 4 weeks, and n = 41 reported being medication-naïve. Furthermore, the majority of patients had one or more comorbid Axis I disorder, with major depressive disorder being the most common comorbidity (n = 39). One OCD patient met the diagnostic criteria for PTSD.
Measures
The severity of OCD symptoms was evaluated using the German version of the Yale-Brown Obsessive-Compulsive Scale (Y-BOCS) [Citation32,Citation33]. Childhood adversity was assessed with the German version of Childhood Trauma Questionnaire (CTQ) [Citation34,Citation35]. For each subject, a sum score was computed based on the 34 Likert items of the scale. Cronbach’s α of the global scale was α = 91, indicating high internal consistency. Stressful life events in the past 12 months were assessed via the Life Experience Survey (LES) [Citation36]. The scale consists of a list of 27 life events and three additional open items, which can be used to name other recent experiences that had a significant impact on the participant’s life. Each event is evaluated regarding its presence (yes/no) and impact (−2 = ‘very negative’ to 2 = ‘very positive’). For each subject, we computed a total score that represents the sum of individual impact ratings over all stressful life events that were reported.
Considering the broad impact of smoking on methylation levels [Citation37], smoking status was assessed as a potential confound. The severity of smoking behaviour was measured via the Fagerström Test for Nicotine Dependence (FTND) [Citation38].
Methylation assessment
DNA was obtained from blood samples using standard procedures. Methylation levels were quantified with the Infinium MethylationEPIC 850k Array (Illumina). Epigenome-wide analyses of the full dataset will be reported elsewhere. Here, we focused on OXTR exon III region Chr3:8,809,281–8,809,534 (UCSC Human Genome Browser; GRCh37/hg19), in order to replicate and extend previous findings. Two CpG sites in this region were captured by the EPIC Array, i.e., cg04523291 (chr3:8,809,501; CpG1) and cg15317815 (chr3:8,809,307; CpG2). Beta values of methylation levels were converted to M values by calculating the log2 ratio of the intensities of methylated probe versus unmethylated probe (log2(Beta/(1-Beta)) [Citation39]. The correlation between methylation levels at CpG1 and CpG2 was high (r = .65, p < 0.001), but not perfect, so that CpGs were analysed separately.
To account for the potential impact of cell-type composition in blood samples, we employed the minfi function ‘estimateCellCounts’ from the Bioconductor tool [Citation40,Citation41], which yields individual estimates for CD8+ T cells (CD8T), CD4+ T cells (CD4T), natural killer cells (NK), CD19+ B cells (Bcell), CD14+ monocytes (mono) and granulocytes (gran). Cases and controls did not significantly differ with respect to CD4T, mono, and gran. As there were significant differences for CD8T (t(377) = 2.43, p = 0.016, d = 0.25), NK (t(375.19) = −1.98, p = 0.048, d = 0.20) and Bcell (t(377) = 3.48, p = 0.001, d = 0.36), these variables were included as covariates in the ANCOVA models testing for case–control differences as well as in mediation analyses (see below). In patients, cell-type composition was neither related to changes in Y-BOCS scores from baseline to post-treatment nor from baseline to follow-up.
Statistical analyses
Group differences in DNA methylation were assessed using ANCOVA with sex, age, and divergent cell composition variables (i.e., CD8T, NK, and Bcell) as covariates. Linear regression analyses were performed to test whether methylation levels predict pre-to-post and pre-to-follow-up changes in Y-BOCS scores, respectively. Logistic regressions were computed to analyse the effects of methylation on treatment response status. Similar to Schiele et al. [Citation5], we included age, Y-BOCS baseline score, and illness duration as covariates in all regression analyses concerning treatment response. To account for multiple testing, a p-value of <0.025 (Bonferroni correction for two CpGs) was considered significant in these main analyses.
To examine the impact of childhood trauma and stressful life events on OXTR methylation, we performed linear regression analysis with CTQ score, LES score, case–control status, and age as independent variables, and methylation as dependent variable. Finally, mediation analyses were conducted using PROCESS [Citation42], to test whether the effects of CTQ and LES on case–control status were mediated by OXTR methylation. To assess mediation, we verified the direct effect of CTQ scores and LES scores, respectively, on OCD caseness using logistic regression, and employed bootstrapping with 10,000 samples to compute confidence intervals (CI) and inferential statistics of the indirect effect for both CTQ and LES on OCD caseness via methylation levels at CpG1. Again, age, sex, CD8T, NK, and Bcell were included as covariates. Given the explorative nature of these additional analyses, a p-value of <0.05 was considered to be significant.
Results
Case–control differences in OXTR methylation
OCD patients exhibited significantly increased DNA methylation at CpG1 compared to controls (F(1, 366) = 8.37, p = 0.004, ηp2 = 0.022). Methylation at CpG2, however, did not differ between cases and controls (F(1, 366) = 0.96, p = 0.33, ηp2 = 0.003). In both ANCOVAs, there were significant effects of age (CpG1: F(1, 366) = 34.84, p < 0.001, ηp2 = 0.087; CpG2: F(1, 366) = 35.17, p < 0.001, ηp2 = 0.088) and sex (CpG1: F(1, 366) = 14.17, p < 0.001, ηp2 = 0.037; CpG2: F(1, 366) = 9.94, p = 0.002, ηp2 = 0.026) on methylation levels, but no significant interactions between sex and caseness.
Methylation and treatment response prediction
In the treatment subsample, CpG1 hypermethylation at baseline was significantly associated with reduced pre-post changes in Y-BOCS scores (β = −0.25, t(85) = −2.56, p = 0.012; ), whereas methylation at CpG2 did not predict pre-post changes in Y-BOCS scores (β = −0.09, t(85) = −0.87, p = 0.39). Likewise, hypermethylation at CpG1 was significantly related to response status as defined by a 35% reduction in Y-BOCS scores from pre- to post-treatment (Wald(1) = 5.41, p = 0.020, OR = 0.19). There was no significant effect of CpG2 (Wald(1) = 1.35, p = 0.25, OR = 0.50) on response status.
Figure 1. Prediction of dimensional treatment response by methylation at CpG site cg04523291 within OXTR exon III. Y-BOCS, Yale-Brown Obsessive Compulsive Scale.
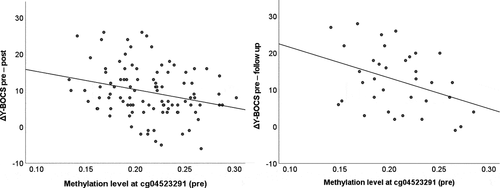
Furthermore, CpG1 methylation at baseline showed a trend-level association with changes in Y-BOCS scores from baseline to follow-up 1 year after the end of treatment (β = −0.34, t(28) = −1.97, p = 0.059). When non-significant covariates (age and illness duration) were excluded to increase statistical power, the effect reached significance (β = −0.36, t(32) = −2.36, p = 0.024; ). For CpG2, there was no significant association (β = −0.26, t(28) = −1.45, p = 0.16).
Associations between CpG1 methylation, childhood trauma, and stressful life events
Using a linear regression analysis, we observed significant effects of age (β = 0.25, t(360) = 4.86, p < 0.001) and case–control status (β = 0.11, t(360) = −2.04, p = 0.042) on methylation at CpG1, whereas the effects of CTQ and LES did not reach significance in the full sample. To account for group differences in methylation, we conducted follow-up analyses stratified by case–control status. In OCD patients, there was a significant effect of LES (β = −0.16, t(163) = −2.00, p = 0.047), but not of CTQ (β = −0.06, t(163) = −0.69, p = 0.49). Conversely, CTQ scores were significantly associated with CpG1 methylation in controls (β = 0.17, t(194) = 2.36, p = 0.019), while LES scores were not (β = 0.01, t(194) = −0.21, p = 0.84).
In the treatment subsample, there was also a significant association between LES scores and methylation at CpG1 (β = −0.22, t(90) = −2.15, p = 0.034). However, LES scores did not predict treatment response (β = 0.17, t(80) = 1.64, p = 0.11).
Mediation analysis
Mediation analysis with LES score as independent variable, case–control status as dependent variable, methylation at CpG1 as mediator and age, sex, CD8T, NK, and Bcell as covariates yielded a significant direct effect of LES score on caseness (B = −0.050, SE = 0.017, Z = −3.06, p = 0.002) as well as a significant association between CpG1 methylation and caseness (B = 0.70, SE = 0.31, Z = 2.27, p = 0.024). There was also a significant effect of LES score on CpG1 methylation (B = −0.0055, SE = 0.0026, t(359) = −2.16, p = 0.032), but the indirect effect in the overall model failed to reach significance (B = −0.0039, SE = 0.0029, [−0.011, 0.0002]).
Similar results were observed for CTQ scores. There was a significant direct effect of CTQ on caseness (B = 0.021, SE = 0.0085, Z = 2.47, p = 0.013), as well as a significant association between CpG1 methylation and caseness (B = 0.73, SE = 0.31, Z = 2.33, p = 0.020). However, the effect of CTQ score on CpG1 methylation did not reach significance (B = 0.0023, SE = 0.0014, t(357) = 1.62, p = 0.11), resulting in an insignificant indirect effect in the overall model (B = 0.0017, SE = 0.0016, [−0.0009, 0.0054]).
Sex effects
Across all subjects, females exhibited significantly higher methylation levels at both CpG1 (t(373) = −2.99, p = 0.003, d = 0.32) and CpG2 (t(373) = −2.58, p = 0.010, d = 0.27) compared to males. Among OCD patients, however, there was no significant difference between females and males (CpG1: t(174) = −1.55, p = 0.12, d = 0.24; CpG2: t(174) = −1.03, p = 0.30, d = 0.16).
Potential impact of other factors
Cases and controls did not differ with respect to smoking status (χ2(1) = 0.81, p = 0.37), and smoking status was not significantly related to DNA methylation at CpG1 (t(365) = −0.25, p = 0.80, d = 0.03) and CpG2 (t(365) = 0.77, p = 0.44, d = 0.09). Furthermore, FTND scores were not associated with methylation at CpG1 (r = 0.02, p = 0.81) and CpG2 (r = 0.13, p = 0.18).
The number of therapy sessions was neither associated with pre-post treatment response (r = 0.07, p = 0.48) nor with DNA methylation (CpG1: r = 0.16, p = 0.11; CpG2: r = 0.07, p = 0.48). Similarly, medication status at baseline was not significantly associated with pre-post treatment response (F(2, 95) = 0.31, p = 0.73, ηp2 = 0.007), methylation at CpG1 (F(2, 95) = 0.16, p = 0.85, ηp2 = 0.003) and CpG2 (F(2, 95) = 0.64, p = 0.53, ηp2 = 0.013).
Discussion
The present study investigated the role of OXTR exon III methylation as a potential biomarker of disease and treatment response in OCD. Replicating our previous findings [Citation5,Citation6], we found that methylation at CpG1 (cg04523291; chr3:8,809,501) was significantly increased in OCD patients compared to controls. Furthermore, CpG1 methylation levels at baseline predicted pre- to post-treatment response and even one-year follow-up treatment response in a naturalistic outpatient setting. Interestingly, methylation at CpG1 was associated with stressful life events in OCD patients, and with childhood adversity in controls. Yet, we did not find compelling evidence that OXTR methylation mediated the associations between childhood adversity or stressful life events and OCD diagnosis.
Our finding of OXTR exon III hypermethylation in OCD is consistent with previous reports from smaller samples [Citation5,Citation6]. Another recent study, however, did not observe a significant difference between OCD patients and healthy controls with regard to methylation of OXTR exon III, but instead found reduced methylation levels at two CpG sites in exon II [Citation7]. This inconsistency may be due to ethnical differences in DNA methylation [Citation43], as Park et al. examined a Chinese sample. Furthermore, the authors conducted sex-stratified analyses with relatively small group sizes, which might have been underpowered to detect effects of caseness on OXTR exon III methylation.
Methylation in the OXTR CpG island spanning across exon I to III has been shown to negatively impact OXTR transcription across tissues [Citation21,Citation44,Citation45]. Yet, evidence for the relation between OXTR methylation and peripheral oxytocin levels is inconclusive. One study reported that increased OXTR methylation at chr3:8,810,833 was negatively associated with circulating oxytocin levels [Citation46], while another study found no significant association between methylation at site −934 upstream of the OXTR start codon (located in exon II) and plasma oxytocin [Citation47]. In rhesus monkeys, OXTR methylation in the blood was not significantly associated with oxytocin concentrations in cerebrospinal fluid [Citation48]. The functional consequences of OXTR hypermethylation in OCD are hence unclear. Still, findings of elevated oxytocin levels in cerebrospinal fluid and blood plasma of OCD patients [Citation11,Citation12] suggest that reductions in OXTR expression might act as a compensatory down-regulation for increased peripheral oxytocin – or vice versa [Citation7,Citation25]. The role of DNA methylation for OCD is further supported by the observation that one subcutaneous dose of the drug RG108, which inhibits DNA methyltransferase (DNMT) and thus leads to reductions in methylation, effectively reduced OCD-like behaviours in three different mouse models of OCD and completely reversed anomalous changes in brain circuitry [Citation49].
Replicating and extending findings from Schiele et al. [Citation5], we observed that OXTR methylation predicted pre-post reductions in Y-BOCS scores as well as treatment response status. While Schiele et al. employed an 8- to 10-week CBT programme for inpatients [Citation5], the present study used a naturalistic outpatient design with a mean number of 46.91 therapy sessions at a frequency of approximately one session per week. Remarkably, methylation at CpG1 predicted a response to such an extensive treatment and was even linked to Y-BOCS changes at one-year follow-up, lending further support to the hypothesis that OXTR methylation represents a promising biomarker of treatment response.
Dysfunctions in the oxytocinergic system may impair treatment response via different mechanisms. First, oxytocin plays an integral part in social processing. Therapy sessions are inherently social situations, and the relationship between patient and therapist is the single most important predictor of treatment response across patient groups and therapy methods [Citation50]. Oxytocinergic dysfunctions may contribute to a deficient therapeutic alliance via reduced social synchrony and thus result in impaired treatment response. Of note, this may represent a transdiagnostic mechanism, since other psychiatric disorders associated with biases in social perception, such as social phobia and PTSB, have also been linked to alterations in OXTR methylation [Citation24,Citation25]. Furthermore, compulsions often fulfil an interpersonal function, in addition to neutralizing negative feelings such as anxiety. For example, many OCD patients seek reassurance from their relatives or partners as a strategy to establish attachment (see ‘Two Stages Model of OCD’) [Citation51]. Impaired social processing due to oxytocinergic imbalances could make OCD patients overly reliant on such interpersonal functions of the disorder and thus hamper their response to CBT. Accordingly, smaller reductions in reassurance seeking have been linked to poorer treatment outcomes [Citation52]. OXTR hypermethylation has also been observed in autism spectrum disorder [Citation45,Citation53], and poor communication skills as measured by an Autism Quotient subscale predicted worse treatment response to ERP-based CBT in OCD [Citation54]. Another explanation, put forward by Schiele et al., concerns the ‘amnestic’ properties of oxytocin [Citation5]. Specifically, oxytocin appears to attenuate memory consolidation and retrieval [Citation55–57]. Considering the importance of inhibition learning for ERP [Citation58], oxytocin may impair treatment response via impeding this learning process. Further research is clearly needed to determine the contribution of these potential change mechanisms.
As possible causes of OXTR hypermethylation, we examined the effects of childhood trauma and stressful life events. Increased OXTR methylation has been linked to childhood trauma in participants from non-psychiatric populations [Citation20–22]. In line with these reports, we also found a significant association between CpG1 methylation and CTQ scores among healthy controls. In OCD patients, however, there was no effect of CTQ scores on methylation levels, but instead, LES scores were significantly associated with methylation at CpG1. Hence, OXTR methylation in OCD appears to be more strongly related to recent adverse events than to more distant ones. Though previous research emphasized the role of early childhood as a particularly vulnerable phase for OXTR methylation [Citation24,Citation59], Simons et al. found that adult adversity was related to OXTR methylation and depression, while childhood adversity was not [Citation60]. Accordingly, epigenetic calibration of various biological systems appears to continue throughout the life course, and may be particularly sensitive in psychiatric patients. In the present study, OCD patients reported lower LES scores compared to controls, indicating more negative and less positive recent life events, which supposedly ‘overwrote’ epigenetic changes linked to childhood adversity. Conversely, effects of childhood adversity on OXTR methylation may have been ‘conserved’ in controls, as they experienced fewer and less negative life events in the past year. These differential effects in cases and controls may also explain why we did not find compelling evidence for a mediation effect of OXTR methylation on the association between environmental risk factors and OCD diagnosis. Still, these findings should be considered preliminary given their marginal significance, and longitudinal data are warranted to corroborate this hypothesis.
Changes in OXTR methylation patterns may contribute to alterations in brain functioning. Specifically, higher OXTR methylation at site −934 is associated with increased activity in brain regions implicated in social processing, and with decreased functional coupling of the amygdala within regions supporting social perception and emotion processing [Citation61,Citation62]. On a molecular level, Quintana et al. observed that the expression of three selected oxytocin pathway genes including OXTR was enriched in subcortical and olfactory regions [Citation9]. Furthermore, there was high co-expression of several dopaminergic and muscarinic acetylcholine genes, and a meta-analysis of fMRI data revealed that the oxytocin pathway gene maps correspond to the processing of anticipatory, appetitive, and aversive cognitive states.
Given that the functions of the oxytocin system are sexually dimorphic [Citation63,Citation64], we considered sex as an important covariate. Among healthy controls, females exhibited significantly increased methylation levels at both OXTR CpGs compared to males, which is in line with previous reports [Citation47,Citation61]. Notably, there were no sex differences among OCD patients, and the group difference between cases and controls was not modulated by sex, suggesting that sexually dimorphic functions of the oxytocin system are potentially attenuated in OCD. Future research may further investigate the role of sex on oxytocinergic functioning in OCD.
The present study is not without limitations. Due to technical feasibility (quantification of methylation using the Infinium MethylationEPIC 850k Array), only two CpGs in OXTR exon III could be examined. As these two CpGs were located towards the front and rear ends of the gene region defined by Schiele et al. [Citation5] and their methylation levels did not inter-correlate perfectly, divergent findings for each CpG are plausible. Specifically, CpG1 was significantly linked to outcome measures of the present study, whereas CpG2 was not. While we concede that the assessment of multiple CpGs may be insightful in exploratory studies, studies with a stronger focus on replication, like ours, may restrict analyses to fewer CpGs, thus mitigating the issue of multiple testing. In the present study, we analysed methylation from blood plasma, but we did not have the opportunity to examine brain samples, precluding definite conclusions regarding central processes. Notably, however, some studies report parallel oxytocin levels in the blood and brain [Citation65,Citation66], and evidence from prairie voles indicates that OXTR methylation state of the blood predicts expression in the brain, supporting the utility of blood as a biomarker for the transcription state of the brain [Citation67]. Furthermore, we did not examine the functional consequences of OXTR hypermethylation on oxytocin levels in blood or cerebrospinal fluid. Future studies may focus on the specific pathways between methylation, gene expression, brain, and clinical phenotypes, preferably using longitudinal designs. Finally, genetic variation in OXTR was not considered as an additional factor in the present study, as Schiele et al. did not observe a significant effect of OXTR rs53576 [Citation5].
In conclusion, we replicated findings of increased OXTR methylation in OCD compared to healthy controls in the largest sample, so far. Furthermore, methylation levels at CpG1 predicted response to ERP-based CBT in a naturalistic outpatient setting with a relatively extensive number of therapy sessions, supporting the role of OXTR methylation as a potential biomarker of treatment response in OCD. In addition, we demonstrated that methylation at CpG1 is sensitive to recent stressful life events in OCD patients, and to childhood adversity in controls. Therapeutic strategies may enhance the focus on social functions established by the oxytocinergic system to improve treatment response.
Acknowledgments
We sincerely thank Björn Elsner, Stephan Heinzel, Leonhard Lennertz and Christian Kaufmann for their great support, as well as all research assistants and subjects who participated in the study.
Disclosure statement
The authors report no conflict of interest.
Additional information
Funding
References
- Ruscio AM, Stein DJ, Chiu WT, et al. The epidemiology of obsessive-compulsive disorder in the national comorbidity survey replication. Mol Psychiatry. 2010;15(1):53–63.
- Stein DJ, Costa DLC, Locher C, et al. Obsessive compulsive disorder. Nat Rev Dis Primers. 2019;5(1):52.
- Hirschtritt ME, Bloch MH, Mathews CA. Obsessive-compulsive disorder advances in diagnosis and treatment. J Am Med Assoc. 2017;317(13):1358–1367.
- Elsner B, Wolfsberger F, Srp J, et al. Long-term stability of benefits of cognitive behavioral therapy for obsessive compulsive disorder depends on symptom remission during treatment. Clin Psychol Eur. 2020;2(1). DOI:https://doi.org/10.32872/cpe.v2i1.2785
- Schiele MA, Thiel C, Kollert L, et al. Oxytocin receptor gene DNA methylation: a biomarker of treatment response in obsessive-compulsive disorder? Psychother Psychosom. Published online 2020. DOI:https://doi.org/10.1159/000509910
- Cappi C, Diniz JB, Requena GL, et al. Epigenetic evidence for involvement of the oxytocin receptor gene in obsessive-compulsive disorder. BMC Neurosc. 2016;17(1):79.
- Park CI, Kim HW, Jeon S, et al. Reduced DNA methylation of the oxytocin receptor gene is associated with obsessive-compulsive disorder. Clin Epigenetics. 2020;12(1):1–8.
- Quintana DS, Lischke A, Grace S, et al. Advances in the field of intranasal oxytocin research: lessons learned and future directions for clinical research. Mol Psychiatry. Published online 2020. DOI:https://doi.org/10.1038/s41380-020-00864-7
- Quintana DS, Rokicki J, van der Meer D, et al. Oxytocin pathway gene networks in the human brain. Nat Commun. 2019;10(1):1–12.
- Marroni SS, Nakano FN, Gati CDC, et al. Neuroanatomical and cellular substrates of hypergrooming induced by microinjection of oxytocin in central nucleus of amygdala, an experimental model of compulsive behavior. Mol Psychiatry. 2007;12(12):1103–1117.
- Leckman JF, Goodman WK, North WG, et al. Elevated cerebrospinal fluid levels of oxytocin in obsessive-compulsive disorder: comparison with tourette’s syndrome and healthy controls. Arch Gen Psychiatry. 1994;51(10):782–792.
- Marazziti D, Baroni S, Giannaccini G, et al. Plasma oxytocin levels in untreated adult obsessive-compulsive disorder patients. Neuropsychobiology. 2015;72(2):74–80.
- Real E, Labad J, Alonso P, et al. Stressful life events at onset of obsessive-compulsive disorder are associated with a distinct clinical pattern. Depress Anxiety. 2011;28(5):367–376.
- Cromer KR, Schmidt NB, Murphy DL. An investigation of traumatic life events and obsessive-compulsive disorder. Behav Res Ther. 2007;45(7):1683–1691.
- Barzilay R, Patrick A, Calkins ME, et al. Association between early-life trauma and obsessive compulsive symptoms in community youth. Depress Anxiety. 2019;36(7):586–595.
- Carpenter L, Chung MC. Childhood trauma in obsessive compulsive disorder: the roles of alexithymia and attachment. Psychol Psychother Theory, Res Pract. 2011;84(4):367–388.
- Kıvılcım S, Erensoy H, Tonguç DB, et al. The comorbidity of traumatic life events in childhood age with adult obsessive-compulsive disorder. Europ Psychiatry. 2016;33(suppl):S497.
- Van Oudheusden LJB, Eikelenboom M, Van Megen HJGM, et al. Chronic obsessive – compulsive disorder : prognostic factors. Psychological Medicine. 2018;48(c):2213–2222.
- Gershuny BS, Baer L, Parker H, et al. Trauma and posttraumatic stress disorder in treatment-resistant obsessive-compulsive disorder. Depress Anxiety. 2008;25(1):69–71.
- Unternaehrer E, Meyer AH, Burkhardt SCA, et al. Childhood maternal care is associated with DNA methylation of the genes for brain-derived neurotrophic factor (BDNF) and oxytocin receptor (OXTR) in peripheral blood cells in adult men and women. Stress. 2015;18(4):451–461.
- Gouin JP, Zhou QQ, Booij L, et al. Associations among oxytocin receptor gene (OXTR) DNA methylation in adulthood, exposure to early life adversity, and childhood trajectories of anxiousness. Sci Rep. 2017;7(1). DOI:https://doi.org/10.1038/s41598-017-07950-x
- Fujisawa TX, Nishitani S, Takiguchi S, et al. Oxytocin receptor DNA methylation and alterations of brain volumes in maltreated children. Neuropsychopharmacology. 2019;44(12):2045–2053.
- Pauls DL, Abramovitch A, Rauch SL, et al. Obsessive-compulsive disorder: an integrative genetic and neurobiological perspective. Nat Rev Neurosci. 2014;15(6):410–424.
- Kraaijenvanger EJ, He Y, Spencer H, et al. Epigenetic variability in the human oxytocin receptor (OXTR) gene: a possible pathway from early life experiences to psychopathologies. Neurosci Biobehav Rev. 2019;96(November2017):127–142.
- Ziegler C, Dannlowski U, Bräuer D, et al. Oxytocin receptor gene methylation: converging multilevel evidence for a role in social anxiety. Neuropsychopharmacology. 2015;40(6):1528–1538.
- Bey K, Lennertz L, Riesel A, et al. Harm avoidance and childhood adversities in patients with obsessive–compulsive disorder and their unaffected first-degree relatives. Acta Psychiatr Scand. 2017;135(4):328–338.
- Bey K, Weinhold L, Grützmann R, et al. The polygenic risk for obsessive-compulsive disorder is associated with the personality trait harm avoidance. Acta Psychiatr Scand. 2020;142(4):326–336.
- Riesel A, Klawohn J, Grützmann R, et al. Error-related brain activity as a transdiagnostic endophenotype for obsessive-compulsive disorder, anxiety and substance use disorder. Psychol Med. 2019;49(7):1207–1217.
- First MB, Spitzer RL, Gibbon M, et al. User’s guide for the structured clinical interview for DSM-IV axis I disorders SCID-I: clinician version. Arlington: American Psychiatric Association Publishing; 1997.
- Wittchen H-U, Zaudig M, Fydrich T. SKID. strukturiertes klinisches interview für DSM-IV. Achse I und II. Handanweisung [Structured Clinical Interview for DSM-IV. Axis I and II. Manual]. 1997.
- Hohagen F, Wahl-Kordon A, Lotz-Rambaldi W, et al. S3-Leitlinie Zwangsstörungen. Springer Berlin Heidelberg; 2015. p. 1–11.
- Goodman WK, Price LH, Rasmussen SA, et al. The yale-brown obsessive compulsive scale: II. validity. Arch Gen Psychiatry. 1989;46(11):1012–1016.
- Hand I, Büttner-Westphal H. Die Yale-Brown Obsessive Compulsive Scale (Y-BOCS): ein halbstrukturiertes Interview zur Beurteilung des Schweregrades von Denk- und Handlungszwängen. Verhaltenstherapie. 1991;1(3):223–225.
- Bernstein DP, Fink L, Handelsman L, et al. Initial reliability and validity of a new retrospective measure of child abuse and neglect. Am J Psychiatry. 1994;151(8):1132–1136.
- Wingenfeld K, Spitzer C, Mensebach C, et al. The German version of the childhood trauma questionnaire (CTQ): preliminary psychometric properties. PPmP Psychother Psychosom Medizinische Psychol. 2010;60(11):442–450.
- Sarason IG, Johnson JH, Siegel JM. Assessing the impact of life changes: development of the life experiences survey. J Consult Clin Psychol. 1978;46(5):932–946.
- Joehanes R, Just AC, Marioni RE, et al. Epigenetic signatures of cigarette smoking. Circ Cardiovasc Genet. 2016;9(5):436–447.
- Heatherton TF, Kozlowsi LT, Frecker RC, et al. The fagerström test for nicotine dependence: a revision of the fagerström tolerance questionnaire. Br J Addict. 1991;86(9):1119–1127.
- Du P, Zhang X, Huang CC, et al. Comparison of Beta-value and M-value methods for quantifying methylation levels by microarray analysis. BMC Bioinformatics. 2010;11(1):587.
- Jaffe AE, Irizarry RA. Accounting for cellular heterogeneity is critical in epigenome-wide association studies. Genome Biol. 2014;15(2):1–9.
- Maksimovic J, Phipson B, Oshlack A. A cross-package bioconductor workflow for analysing methylation array data. F1000Res. 2016;5(3):1281.
- Hayes A. Introduction to mediation, moderation, and conditional process analysis: a regression-based approach. 2017.
- Zhang FF, Cardarelli R, Carroll J, et al. Significant differences in global genomic DNA methylation by gender and race/ethnicity in peripheral blood. Epigenetics. 2011;6(5):623–629.
- Kusui C, Kimura T, Ogita K, et al. DNA methylation of the human oxytocin receptor gene promoter regulates tissue-specific gene suppression. Biochem Biophys Res Commun. 2001;289(3):681–686.
- Gregory SG, Connelly JJ, Towers AJ, et al. Genomic and epigenetic evidence for oxytocin receptor deficiency in autism. BMC Med. 2009;7. DOI:https://doi.org/10.1186/1741-7015-7-62.
- Dadds MR, Moul C, Cauchi A, et al. Methylation of the oxytocin receptor gene and oxytocin blood levels in the development of psychopathy. Dev Psychopathol. 2014;26(1):33–40.
- Rubin LH, Connelly JJ, Reilly JL, et al. Sex and diagnosis specific associations between DNA methylation of the oxytocin receptor gene with emotion processing and temporal-limbic and prefrontal brain volumes in psychotic disorders HHS Public Access. Biol Psychiatry Cogn Neurosci Neuroimaging. 2016;1(2):141–151.
- De Leon D, Nishitani S, Walum H, et al. Methylation of OXT and OXTR genes, central oxytocin, and social behavior in female macaques. Horm Behav. 2020;126. DOI:https://doi.org/10.1016/j.yhbeh.2020.104856.
- Todorov G, Mayilvahanan K, Ashurov D, et al. Amelioration of obsessive-compulsive disorder in three mouse models treated with one epigenetic drug: unraveling the underlying mechanism. Sci Rep. 2019;9(1):1–13.
- Orlinsky DE, Ronnestad ML, Willutzki U. Fifty years of psychotherapy process-outcome research: continuity and change. In: Lambert M, editor. Bergin and Garfield’s handbook of psychotherapy and behavior change. 5th ed. New York (NY): Wiley; 2004. p. 307–389.
- Hoffmann N, Hofmann B. Expositionen bei Ängsten und Zwängen: Praxishandbuch. 2004.
- Rector NA, Katz DE, Quilty LC, et al. Reassurance seeking in the anxiety disorders and OCD: construct validation, clinical correlates and CBT treatment response. J Anxiety Disord. 2019;67. DOI:https://doi.org/10.1016/j.janxdis.2019.102109.
- Rijlaarsdam J, van IJzendoorn MH, Verhulst FC, et al. Prenatal stress exposure, oxytocin receptor gene (OXTR) methylation, and child autistic traits: the moderating role of OXTR rs53576 genotype. Autism Res. 2017;10(3):430–438.
- Hamatani S, Tsuchiyagaito A, Nihei M, et al. Predictors of response to exposure and response prevention-based cognitive behavioral therapy for obsessive-compulsive disorder. BMC Psychiatry. 2020;20(1):1–8.
- Bohus B. Vasopressin oxytocin and memory: effects on consolidation and retrieval processes. Acta Psychiatr Belg. 1980 [cited 2020 Nov 26];80(5):714–720. Available from: https://pubmed.ncbi.nlm.nih.gov/7234456/
- Ferrier BM, Kennet DJ, Devlin MC. Influence of oxytocin on human memory processes. Life Sci. 1980;27(24):2311–2317.
- Heinrichs M, Meinlschmidt G, Wippich W, et al. Selective amnesic effects of oxytocin on human memory. Physiol Behav. 2004;83(1SPEC. ISS.):31–38.
- Craske MG, Treanor M, Conway CC, et al. Maximizing exposure therapy: an inhibitory learning approach. Behav Res Ther. 2014;58:10–23.
- Nagy C, Turecki G. Sensitive periods in epigenetics: bringing us closer to complex behavioral phenotypes. Epigenomics. 2012;4(4):445–457.
- Simons RL, Lei MK, Beach SRH, et al. Methylation of the oxytocin receptor gene mediates the effect of adversity on negative schemas and depression. Dev Psychopathol. 2017;29(3):725–736.
- Puglia MH, Lillard TS, Morris JP, et al. Epigenetic modification of the oxytocin receptor gene influences the perception of anger and fear in the human brain. Proc Natl Acad Sci U S A. 2015;112(11):3308–3313.
- Jack A, Connelly JJ, Morris JP. DNA methylation of the oxytocin receptor gene predicts neural response to ambiguous social stimuli. Front Hum Neurosci. 2012;6:280.
- Bredewold R, Veenema AH. Sex differences in the regulation of social and anxiety-related behaviors: insights from vasopressin and oxytocin brain systems. Curr Opin Neurobiol. 2018;49:132–140.
- Dumais KM, Veenema AH. Vasopressin and oxytocin receptor systems in the brain: sex differences and sex-specific regulation of social behavior. Front Neuroendocrinol. 2016;40:1–23.
- Carson DS, Berquist SW, Trujillo TH, et al. Cerebrospinal fluid and plasma oxytocin concentrations are positively correlated and negatively predict anxiety in children. Mol Psychiatry. 2015;20(9):1085–1090.
- Dal Monte O, Noble PL, Turchi J, et al. CSF and blood oxytocin concentration changes following intranasal delivery in macaque. PLoS One. 2014;9(8). DOI:https://doi.org/10.1371/journal.pone.0103677
- Perkeybile AM, Carter CS, Wroblewski KL, et al. Early nurture epigenetically tunes the oxytocin receptor. Psychoneuroendocrinology. 2019;99:128–136.