ABSTRACT
Pregnancy can exacerbate or prompt the onset of stress-related disorders, such as post-traumatic stress disorder (PTSD). PTSD is associated with heightened stress responsivity and emotional dysregulation, as well as increased risk of chronic disorders and mortality. Further, maternal PTSD is associated with gestational epigenetic age acceleration in newborns, implicating the prenatal period as a developmental time period for the transmission of effects across generations. Here, we evaluated the associations between PTSD symptoms, maternal epigenetic age acceleration, and infant gestational epigenetic age acceleration in 89 maternal-neonatal dyads. Trauma-related experiences and PTSD symptoms in mothers were assessed during the third trimester of pregnancy. The MethylationEPIC array was used to generate DNA methylation data from maternal and neonatal saliva samples collected within 24 h of infant birth. Maternal epigenetic age acceleration was calculated using Horvath’s multi-tissue clock, PhenoAge and GrimAge. Gestational epigenetic age was estimated using the Haftorn clock. Maternal cumulative past-year stress (GrimAge: p = 3.23e-04, PhenoAge: p = 9.92e-03), PTSD symptoms (GrimAge: p = 0.019), and difficulties in emotion regulation (GrimAge: p = 0.028) were associated with accelerated epigenetic age in mothers. Maternal PTSD symptoms were associated with lower gestational epigenetic age acceleration in neonates (p = 0.032). Overall, our results suggest that maternal cumulative past-year stress exposure and trauma-related symptoms may increase the risk for age-related problems in mothers and developmental problems in their newborns.
Introduction
Pregnancy can be a stressful period for women due to potential changes in relationships, health-related concerns, preparation for parenthood, and increased financial strain [Citation1,Citation2]. These pregnancy-related stressors coupled with the effects of fluctuating hormones may exacerbate or prompt the onset of psychological impairment, particularly stress-related disorders, such as post-traumatic stress disorder (PTSD) [Citation3–5]. PTSD also may occur as a sequela of pregnancy-related stressors that are traumatic in nature (e.g., intimate partner violence), and it may be precipitated or exacerbated by an accumulation of exposures to stressors that occur during pregnancy but are neither traumatic nor directly related to pregnancy [Citation4]. PTSD is associated with heightened emotional and physiological reactivity and altered regulation of both physiological and behavioural responses to stressors, in addition to increased risk of chronic age-related disorders and earlier mortality [Citation6–8]. Prenatal PTSD prevalence may reach to 40% in low-income, minority populations with known health disparities [Citation8,Citation9]. McEwen’s stress model describes the perinatal period as a ‘window of opportunity’ [Citation10], where maternal stress exposure and PTSD may influence foetal health trajectories via placental-foetal stress physiology [Citation11,Citation12], epigenetic processes [Citation13], and problems with caregiving [Citation14]. Indeed, studies have linked maternal PTSD to negative perinatal outcomes, including pre-term birth and low birth weight [Citation9], as well as developmental problems in young children [Citation15–17].
In addition, lifetime stress exposure [Citation18] and PTSD [Citation19] have been shown to be associated with problems in maternal emotion dysregulation. When mothers of infants or young children experience difficulties in emotion regulation, this has been found to be associated with problems in parenting [Citation20], maternal-child attachment bonding [Citation21], and their child’s development and psychological functioning, such as child social-emotional/behavioural problems [Citation22]. Difficulties in maternal emotion regulation during pregnancy or soon after childbirth may impair maternal-infant emotional co-regulation and precipitate a cascade of problems with parenting, attachment, and infant development and functioning [Citation23,Citation24].
Intergenerational effects of maternal stress exposure and PTSD are also associated with DNA methylation (DNAm) age acceleration in the offspring [Citation25]. DNAm age is a reliable measure of biological age [Citation26–29], which refers to the biological wellbeing of the body based on an individual’s physiological state and cellular health. A significant body of literature supports an association between PTSD and DNAm age acceleration (i.e., higher DNAm age values compared to chronological age) in adults [Citation30–32], measured by Horvath’s multi-tissue clock [Citation26] and Hannum’s blood-specific clock [Citation27]. These earlier epigenetic clocks, Horvath’s multi-tissue clock [Citation26] and Hannum’s blood-specific clock [Citation27], were trained on chronological age and proposed as a measure of biological ageing. However, the second-generation epigenetic clocks, PhenoAge that includes clinical biomarkers as predictors [Citation28], and GrimAge that was developed by regressing time-to-death on DNAm based age-related biomarkers and smoking packs per year [Citation29], were proposed as an epigenetic predictors of healthspan and long-term mortality risk. Recent evidence reports accelerated DNAm GrimAge in those with PTSD [Citation33–37]. Of those, the study of Katrinli et al. conducted in a predominantly African American cohort showed that individuals with current and lifetime PTSD have accelerated GrimAge, which also associated with cortical atrophy in brain areas related to emotion processing and threat response [Citation33]. Yang et al. reported accelerated GrimAge in male veterans with PTSD, as well as a significant association between longitudinal changes in GrimAge acceleration and PTSD symptoms at 3-years follow-up [Citation34].
However, the measures of DNAm age acceleration do not accurately translate to earlier phases of life in which deceleration rather than acceleration of ageing represents a potential adverse outcome. Hence, recent efforts have focused on developing gestational epigenetic clocks predictive of gestational age (i.e., gestational epigenetic age) [Citation38–42]. The prediction accuracy of gestational epigenetic age clocks is more concordant with gestational age predictions assessed with the gold standard of ultrasound than with predictions based on the last menstrual period (LMP) [Citation39]. The difference between gestational epigenetic age and clinically estimated gestational age (i.e., gestational epigenetic age acceleration) may serve as a surrogate indicator of the physiological development of the neonate, with higher gestational epigenetic age acceleration values (i.e., accelerated gestational epigenetic age) potentially indicating more developmental maturity in neonates [Citation39]. Recently, Suarez et al. reported a significant association between maternal depression and lower gestational epigenetic age acceleration, which in turn is associated with developmental problems in boys [Citation43]. A recent study by Koen and colleagues, conducted on 271 Southern African mothers and infant dyads, showed that maternal PTSD was associated with lower gestational epigenetic age acceleration in offspring [Citation44]. Cumulative exposure to recent stressors is associated with both depression [Citation45] and PTSD [Citation46]; but, the impact of maternal cumulative stress exposure, PTSD, and premature epigenetic ageing on the gestational epigenetic age acceleration of their newborn child has not been investigated.
Therefore, we first aimed to evaluate the association between past-year cumulative stress exposure, prenatal PTSD symptoms, and premature accelerated ageing in women, using three different epigenetic clocks, Horvath’s multi-tissue clock, PhenoAge, and GrimAge. Then, we sought to investigate the relationship of maternal stress exposure and PTSD symptoms with gestational epigenetic age acceleration in their newborn child.
Materials and methods
Sample
Salivary DNA samples from 96 mother-neonatal dyads were selected for a genome-wide methylation analysis from a cohort of 203 Hispanic/Latina participants enrolled in a larger study focused on maternal trauma and prenatal stress. The selection was made to test research aims from two supplemental projects [information withheld] budgeted to assay samples from 96 dyads using the MethylationEPIC BeadChip (Illumina). Among the cohort of 203 women, 68 were excluded from selection due to exclusion criteria of the larger study (i.e., infant placed in the NICU, twins, delivered elsewhere precluding collection of samples at birth), exclusion criteria of the current study (i.e., no trauma endorsed on the trauma history questionnaire), study withdrawal prior to sample collection, or missing key data (i.e., missing maternal and/or neonatal DNA sample, full trauma history and symptom data). Among the 135 remaining dyads, 96 were selected such that 48 were drawn sequentially starting from the highest maternal PTSD symptom score and moving downwards and 48 were drawn sequentially starting from the lowest PTSD symptom score and moving upwards. This approach was to ensure sufficient variability in PTSD symptoms. Among the 96 dyads selected for the current study, 7 dyads were removed following DNA methylation quality control (described below), resulting in an analytic sample of 89 dyads. See for a flowchart of the analytic sample.
Procedure
Third-trimester patients seen at an urban prenatal care clinic were invited to participate. Eligibility criteria included English-speaking, singleton pregnancy, and plan to deliver at [information withheld] Hospital. Participants provided written, informed consent in private locations and were asked to summarize their understanding of material before enrolment. Enrolled participants completed self-report measures of past-year stressful events, lifetime trauma history, PTSD symptoms, and emotion dysregulation. Salivary DNA samples were collected from mothers and infants within 24 h of delivery. All included study mothers reported at least one type of past exposure to traumatic event(s), and all infants who had a gestational age of at least 34 weeks. All study procedures and protocols were approved by institutional review boards from the [information withheld].
Measures
Demographic information on age, race, and smoking (lifetime and during pregnancy) was collected by self-report. Newborn characteristics (i.e., sex, gestational age, head circumference, length, weight) were obtained via medical chart extraction. Past-year stressful life events (e.g., serious accident or injury, family went on welfare) were assessed using the widely used and validated Turner life events scale (LES) [Citation47]. LES score was operationalized as a continuous measure, by summing 34 dichotomous items assessing the presence of past-year events.
Lifetime trauma history and DSM-5 PTSD symptoms were assessed via self-report with the Structured Trauma-Related Experiences and Symptoms Screener for Adults (STRESS-A) [Citation8,Citation48]. Part I assessed lifetime exposure to 29 PTSD qualifying traumatic events. In Part II, respondents rated the frequency of symptoms over the past week on a 4-point rating scale (0=none, 1=1 day, 2=2–3 days, 3=most days). Cronbach’s α for total symptom severity was 0.949.
Emotion dysregulation was assessed with the Difficulties in Emotion Regulation Scale (DERS) [Citation49]. The DERS is a 36-item self-report measure of six dimensions of emotion dysregulation: Non-acceptance, Goals, Impulse, Strategy, Clarity, and Awareness. Respondents indicate how often each statement applies to them on a 5-point rating scale (1=almost never, 2=sometimes, 3=about half the time, 4=most of the time, 5=almost always). The DERS exhibits good reliability and validity among racially diverse demographic groups. The DERS total score (sum of subscales) was used (α= .927).
DNA methylation and epigenetic clocks
Saliva samples were collected from mothers using Oragene DNA collection kits. Saliva samples from neonates were collected using five micro-sponges. Both samples were collected within 24 hours post-delivery. DNA was extracted from saliva samples using the Oragene Prepit L2P procedure by DNA Genotek and assayed using the MethylationEPIC BeadChip (Illumina). The R package CpGassoc was used for quality control steps, including i) removal of samples with probe detection call rates < 90% and an average intensity value of either < 50% of the experiment-wide sample mean or < 2000 arbitrary units (removed 4 mother-neonatal dyad samples and 3 neonatal samples); ii) setting probes with detection p-values >0.01 as missing; and iii) filtering out missing probes for > 10% of samples [Citation50]. Probes that were known to cross-hybridize between autosomes and sex chromosomes were filtered out [Citation51]. Methylation data was preprocessed and normalized using single-sample Noob (ssNoob) background correction implemented in R package minfi [Citation52]. Batch effects of chip and position were removed using ComBat [Citation53]. We also excluded mother pairs of 3 failed neonatal samples. The analytical sample included 89 mother-neonatal dyads.
DNAm data was used to estimate saliva cellular composition, and a smoking score for each sample. Saliva cell proportions (i.e., epithelial, fibroblast, CD8+T, CD4+T, natural killer (NK), B cells, monocytes, and neutrophils) was estimated using the hepidish function from R package Epidish [Citation54]. The smoking score was generated using the weights of 39 CpG sites associated with smoking pack years [Citation55] (Supplementary Table S1), as previously described [Citation56].
Horvath’s online calculator (https://dnamage.genetics.ucla.edu/new) was used to calculate epigenetic age and age acceleration (age-adjusted epigenetic age) measures, Horvath’s multi-tissue clock, PhenoAge, and GrimAge. Gestational epigenetic age of infants was calculated based on the recent EPIC-based gestational clock developed by Haftorn and colleagues [Citation42]. We regressed gestational epigenetic age on chronological gestational age in weeks to obtain gestational epigenetic age acceleration.
Statistical analyses
We first evaluated the correlation between epigenetic age estimates and chronological age in mothers, and the correlation between DNAm gestational age and chronological gestational age in infants to validate the accuracy of these epigenetic clocks for saliva samples.
In mothers, we tested the correlations between epigenetic age acceleration measures and potential confounders, including cell proportions, self-reported smoking and marijuana use (lifetime and during pregnancy), and DNAm-derived smoking score. We conducted separate linear regressions to test the associations between each epigenetic age acceleration measures (GrimAge acceleration, PhenoAge acceleration, and Horvath’s DNAmAge acceleration) and each stress and trauma-related measures (i.e., LES, STRESS, DERS). We then employed full models with each epigenetic age acceleration measures as the dependent variables and all three trauma-related measures as independent variables. All models were adjusted for proportions of monocyte, B cell, NK, CD4+T and epithelial cells. Since, GrimAge incorporates DNAm surrogate of smoking assessed by smoking packs per year, a DNAm-based smoking score was not included in the regression model for GrimAge. For PhenoAge and Horvath’s DNAmAge models, post-hoc sensitivity analyses explored the possible confounding effect of smoking by including DNAm-based smoking score as a covariate.
In infants, we tested the correlations of gestational epigenetic age acceleration with cell proportions, anthropomorphic variables of infants (e.g., sex, length, weight, and head circumference), and smoking status of mothers. We used a linear regression model to test the association between gestational epigenetic age acceleration and maternal stress and trauma-related measures (i.e., LES, STRESS, DERS), including infant’s sex and estimated cell proportions (i.e., monocyte, B cell, NK, CD4+T and epithelial cells) as covariates. Since, fibroblast and CD8+T proportions were too low, they were not included as covariates. A post-hoc sensitivity analysis also examined the possible confounding effect of maternal smoking by including maternal DNAm-based smoking score as a covariate.
Results
Demographic and clinical characteristics
Demographic and clinical characteristics of mothers and their newborn infants are presented in . All mothers in this study self-identified as Hispanic. None of the subjects reported alcohol use during pregnancy. Correlations between sociodemographic characteristics and study variables are presented in Supplementary Figure S1.
Table 1. Demographic and clinical characteristics of the study.
Life stress, PTSD symptoms, and emotion regulation problems associate with GrimAge
All three epigenetic age measures (GrimAge, PhenoAge, and Horvath’s DNAmAge) strongly correlated with maternal chronological age (r > 0.85, p < 2.2e-16, Supplementary Figure S2). Of the three epigenetic clocks, GrimAge showed the strongest correlation with chronological age (r = 0.89, p < 2.2e-16). Pairwise correlations between epigenetic age acceleration measures and potential confounders, including cell proportions, self-reported smoking and marijuana use (lifetime and during pregnancy), and DNAm-derived smoking score were shown in Supplementary Figure S3.
Exposure to past-year stressful life events (), PTSD symptoms (), and difficulties in emotion regulation () were each significantly associated with accelerated GrimAge in mothers (), suggestive of premature accelerated ageing. Exposure to past-year stressful life events was also associated with accelerated PhenoAge (). However, the association did not remain significant in the post-hoc sensitivity analysis that adjusts for DNAm-based smoking score (beta estimate = 0.23, p = 0.08). Horvath’s DNAmAge acceleration was not associated with any stress and trauma-related measures (). In the full model that includes all three trauma-related measures, only past-year stressful life events remained associated with GrimAge acceleration and PhenoAge acceleration ().
Figure 2. Associations between GrimAge acceleration and (a) number of past-year stressful life events (LES), (b) PTSD symptoms (STRESS), and (c) difficulties in emotion regulation in mothers (DERS). Results are from multiple linear regression models, adjusted for proportions of monocyte, B cell, NK, CD4+T and epithelial cells. Gray shaded area represents 95% confidence region.
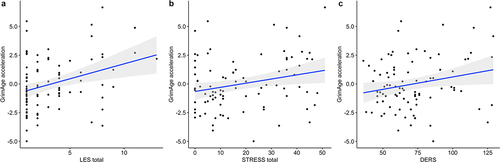
Table 2. Associations of GrimAge acceleration and gestational epigenetic age acceleration with maternal stress, PTSD symptoms, and difficulties in emotion regulation.
Gestational epigenetic age acceleration of infants associates with maternal PTSD symptoms
Newborn infants’ gestational epigenetic age was correlated with their chronological gestational age (r = 0.38, p = 2.9e-04, Supplementary Figure S4). Gestational epigenetic age acceleration was not correlated with infant’s length at birth, weight, or head circumference, but correlated with infant sex, with females having higher gestational epigenetic age acceleration (Supplementary Figure S5). Gestational epigenetic age acceleration was positively correlated with the proportions of epithelial cells, and negatively correlated with proportions of B cells and monocytes. There was no significant correlation between gestational epigenetic age acceleration and smoking status of mothers for both self-reported smoking and DNAm derived smoking score. Gestational epigenetic age acceleration of neonates was correlated with maternal PhenoAge acceleration (r = 0.22, p = 0.038), but not with maternal GrimAge (r = −0.16, p = 0.14) and Horvath’s DNAmAge acceleration (r = 0.09, p = 0.40).
Maternal PTSD symptoms were negatively associated with their newborn’s gestational epigenetic age acceleration (, ), suggestive of a plausible delayed foetal development. The association remained significant with the same direction of effect in the sensitivity analysis adjusted for maternal smoking score (beta estimate = −3.58e-04, p = 0.49). In the stratified analysis for neonatal sex, maternal PTSD symptoms were negatively associated with gestational epigenetic acceleration in female neonates (beta estimate = −0.005, p = 0.019), but not in males (beta estimate = −0.001, p = 0.68). However, neonatal sex did not moderate the association between maternal PTSD symptoms and their newborn’s gestational epigenetic age acceleration (beta estimate = −0.002, p = 0.57). Infant gestational epigenetic age acceleration did not associate with maternal past-year stressful life events or emotion dysregulation ().
Discussion
In this study, we evaluated the associations between stress and trauma-related metrics and epigenetic indicators of ageing and mortality risk in mother-neonatal dyads. Our major findings were as follows: 1) Maternal exposure to past-year stressful life events were associated with accelerated GrimAge and PhenoAge, whereas PTSD symptoms and emotion dysregulation were only associated with accelerated GrimAge; 2) In addition, maternal PTSD symptoms, although not maternal report of stress exposure or difficulty with emotion regulation, were associated with lower gestational epigenetic age acceleration in neonates.
Our findings from saliva were consistent with previous reports from blood linking accelerated GrimAge to trauma burden and PTSD [Citation33–36]. Saliva is composed of a mix of epithelial cells and leukocytes (the main component of blood tissue). Although GrimAge was developed in blood samples, GrimAge estimates calculated from blood and buccal (predominantly consisting of epithelial cells) samples from the same N = 21 subjects were shown to be correlated (r = 0.48, p < 0.001) [Citation57]. In addition, multiple studies report that saliva derived GrimAge were strongly correlated with chronological age (r > 0.6, p < 0.001) [Citation57–59]. Notably, a recent longitudinal study reported that PTSD symptom severity upon trauma exposure associated with GrimAge acceleration calculated from saliva samples [Citation37]. Since GrimAge was trained on time-to-death and incorporates DNAm-based surrogates of age-related biomarkers, it can accurately predict mortality and morbidity [Citation60]. Hence, accelerated GrimAge in mothers who experienced a greater number of past-year stressful life events and have higher PTSD symptoms is suggestive of a greater risk of earlier mortality. On the other hand, exposure to past-year stressful life events, but not PTSD symptoms or difficulty with emotion regulation, were associated with higher PhenoAge acceleration. These findings were consistent with previous studies that examined all three epigenetic clocks and showed only GrimAge acceleration, but not PhenoAge and Horvath’s DNAm acceleration, was associated with PTSD [Citation35,Citation36].
In our neonate samples, females showed higher gestational epigenetic age acceleration compared to males. Although adult males exhibit higher biological age (i.e., epigenetic age acceleration) compared to females [Citation26], the same phenomenon doesn’t apply to gestational epigenetic age acceleration. Adult epigenetic age acceleration is a predictor of biological ageing [Citation26], whereas gestational age acceleration reflects offspring development and maturity [Citation39]. Hence, there is no well-established sex bias for gestational epigenetic age acceleration, with some studies reporting higher gestational epigenetic age acceleration in female offspring [Citation61–63], and others in male offspring [Citation64–66]. To further evaluate sex differences in gestational epigenetic age acceleration, we conducted a sex-stratified analysis and evaluated neonate sex as a moderator of the association between gestational age acceleration and maternal PTSD symptoms. Our findings did not show a sex-specific association between gestational age acceleration and maternal PTSD symptoms. In addition, gestational epigenetic age acceleration was correlated with maternal PhenoAge acceleration, but not with maternal GrimAge or Horvath’s DNAmAge acceleration. To the best of our knowledge, no study to date reported a correlation between gestational epigenetic age acceleration and maternal epigenetic age acceleration.
Although maternal PTSD symptoms was correlated with both emotional dysregulation (r = 0.53, p < 0.01), and exposure to past-year stressful life events (r = 0.59, p < 0.01, Supplementary Figure S1), only maternal PTSD symptoms were associated with gestational epigenetic age deceleration in neonates. Since LES only measures recent stressful and traumatic events, whereas STRESS assesses lifetime events and associated PTSD symptoms, we can speculate that cumulative exposure and trauma-specific symptoms might be necessary for reliable epigenetic changes to occur. In addition, PTSD usually co-occurs with immune dysregulation [Citation67], which might influence gestational epigenetic age acceleration [Citation68].
At first glance, the findings in neonates may seem contradictory to our findings in mothers and previous adult studies that reported associations between accelerated epigenetic age and trauma-related experiences and symptoms [Citation30–34,Citation37]. Moreover, children at ages 6 to 13 years exposed to neighbourhood violence also exhibited higher epigenetic age acceleration [Citation25]. However, epigenetic age acceleration measures trained on adult and paediatric samples are not suitable for use in neonates, nor for estimating gestational age [Citation39]. Since these epigenetic age clocks are not trained on gestational age data, they do not correlate with gestational age [Citation39,Citation69]. Studies investigating the associations of gestational epigenetic age acceleration with prenatal adversities and foetal growth yield mixed results. Lower gestational epigenetic age acceleration has been associated with i) prenatal maternal health adversities, including assisted delivery, prior insulin-treated gestational diabetes mellitus, maternal Sjögren’s syndrome, maternal history of depression, and antenatal depressive symptom severity [Citation43,Citation63,Citation70–72]; ii) prenatal stress assessed using the cerebroplacental ratio [Citation70]; iii) male neonate sex [Citation61–63]; iv) neonatal development delay, including lower birth weight, length, and head circumference, development of bronchopulmonary dysplasia and higher need for respiratory intervention [Citation62,Citation63]; and v) prospective child psychiatric problems [Citation43]. Contradicting reports have shown associations between accelerated gestational epigenetic age and lower birth length, lower 1-min Apgar scores, male neonate sex, maternal preeclampsia, and maternal age greater than 40 years at delivery [Citation41,Citation63–66]. Nevertheless, our results align with a recent study reporting an association between lower gestational epigenetic age and maternal trauma-related experiences and symptoms [Citation44]. The findings of our study and others linking maternal prenatal mental health problems (e.g., maternal antenatal depressive symptoms or PTSD) with lower gestational epigenetic age acceleration in the neonates are aligned with the Developmental Origins of Health and Diseases (DOHaD) hypothesis, which suggests that experiences during the prenatal period can influence the developmental trajectory of the foetus [Citation73].
This study is not without limitations. Firstly, the relatively small sample size restricted the statistical power of our analyses. Even so, we have reported significant associations of GrimAge acceleration with stress and trauma-related metrics and PTSD symptoms, as well as an association between gestational epigenetic age acceleration and maternal PTSD symptoms, which is consistent with previous reports [Citation33–35,Citation37,Citation44].
Second, our cohort is racially homogenous and only includes mother-neonatal dyads of Hispanic ethnicity. Hence, it should be acknowledged that Hispanics exhibit lower GrimAge acceleration compared to white populations, and our results may not generalize to other ethnic or racial groups [Citation74]. However, there is an empiric need to increase research in Hispanic and other minoritized groups, as such our focus on data within Hispanic families has specific relevance. Future studies that examine effects between different minoritized populations, rather than the typical comparison of minority groups to whites, are needed to better understand how structural inequity may differentially effect minoritized groups, a key knowledge gap when considering culturally responsive intervention and prevention efforts.
Third, all participants are from a low socioeconomic group, with 75.8% of participants reporting an annual income < $20,000 and 95.2% relying on Medicare/Medicaid. Since there was no substantive variability in socioeconomic status, the models were not adjusted for socioeconomic status, which has been associated with epigenetic age acceleration [Citation57].
Fourth, we used GrimAge and PhenoAge clocks that were originally developed in blood samples to estimate epigenetic age in saliva samples. However, the chronological age is strongly correlated with both GrimAge (r = 0.89, p < 2.2e-16) and PhenoAge estimates (r = 0.85, p < 2.2e-16), indicating that PhenoAge and GrimAge are applicable to saliva samples in our study. Levine and colleagues also demonstrated that PhenoAge shows strong blood-saliva correlation (r = 0.81, p = 7e-60) [Citation28]. In addition, multiple studies report that saliva-derived GrimAge were strongly correlated with chronological age (r > 0.6, p < 0.001) [Citation57–59]. Notably, a recent longitudinal study reported that PTSD symptom severity upon trauma exposure is associated with GrimAge acceleration calculated from saliva samples [Citation37].
Fifth, we used the recent EPIC-based gestational epigenetic clock trained on cord blood samples to predict gestational epigenetic age in our neonate saliva samples, as no gestational epigenetic clock specific to saliva tissue yet exists. However, the significant -albeit moderate- correlation between gestational epigenetic age and chronological gestational age (r = 0.38, p = 2.9e-04), despite being weaker compared to studies that used the Haftorn clock in cord blood (r = 0.43–0.65, p < 0.001) [Citation61,Citation64,Citation72,Citation75,Citation76], suggests that the recent EPIC-based gestational epigenetic clock is applicable to saliva samples in our study.
Finally, we did not examine or control for the effects of neither maternal and pregnancy health (e.g., gestational diabetes, maternal obesity) nor cumulative stress prior to pregnancy. Hence, we were not able to segregate the impacts of stress during pregnancy on GrimAge acceleration and gestational epigenetic age acceleration from earlier life stress and maternal physical health.
In conclusion, our study shows that pregnant women reporting exposure to more past-year stressors and exhibiting more PTSD symptoms and difficulties with emotion regulation demonstrated accelerated GrimAge soon after childbirth, suggestive of a higher mortality risk. Further, infants born to mothers with higher PTSD symptoms during pregnancy exhibit lower gestational epigenetic age acceleration, which may indicate elevated risk of later developmental problems [Citation43]. Future prospective studies with larger sample sizes are required to investigate possible interactions between PTSD symptoms and GrimAge acceleration in relation to gestational epigenetic age acceleration and prospective child outcomes.
Author Contributions
1. Substantial contributions to the conception or design of the work; or the acquisition, analysis, or interpretation of data for the work
a. Seyma Katrinli
b. Damion Grasso
c. Jonathan Covault
d. Vijender Singh
e. Bo Reese
f. Victoria Scranton
g. Pamela Fall
h. Amy Johnson
2. Drafting the work or revising it critically for important intellectual content
a. Seyma Katrinli
b. Damion Grasso
c. Stacy Drury
d. Margaret Briggs-Gowan
e. Julian Ford
f. Alicia Smith
3. Final approval of the version to be published
a. Seyma Katrinli
b. Damion Grasso
4. Agreement to be accountable for all aspects of the work in ensuring that questions related to the accuracy or integrity of any part of the work are appropriately investigated and resolved.
a. Seyma Katrinli
b. Damion Grasso
c. Bo Reese
d. Vijender Singh
e. Pamela Fall
f. Jonathan Covault
Supplemental Material
Download MS Word (1.3 MB)Acknowledgments
We thank Ms. Meghan Fish for her role in participant recruitment and data collection. We thank Drs. Joseph Newell and Margaret McLaren, as well as to Drs. Sandra Motta and Sonia Chaudhry and the medical staff at Hartford Hospital on the Labor and Delivery and Postpartum units for their participation in sample collection, as well as staff at the Hartford Hospital Women’s Ambulatory Health Services Clinic for their critical role in supporting recruitment and enrollment.
Disclosure statement
No potential conflict of interest was reported by the authors.
Supplementary material
Supplemental data for this article can be accessed online at https://doi.org/10.1080/15592294.2023.2231722
Data availability statement
The data that support the findings of this study are available from the corresponding author, DJG, upon reasonable request.
Additional information
Funding
References
- Yali AM, Lobel M. Coping and distress in pregnancy: an investigation of medically high risk women. J Psychosom Obstet Gynaecol. 1999;20(1):39–14. doi: 10.3109/01674829909075575
- Ibrahim SM, Lobel M. Conceptualization, measurement, and effects of pregnancy-specific stress: review of research using the original and revised prenatal distress questionnaire. J Behav Med. 2020;43(1):16–33. doi: 10.1007/s10865-019-00068-7
- Yali AM, Lobel M. Stress-resistance resources and coping in pregnancy. Anxiety, Stress & Coping: An International Journal. 2002;15(3):289–309. doi: 10.1080/1061580021000020743
- Lobel M, Hamilton JG, Cannella DT. Psychosocial perspectives on pregnancy: prenatal maternal stress and coping. Soc Personal Psychol Compass. 2008;2(4):1600–1623. doi: 10.1111/j.1751-9004.2008.00119.x
- Seng JS, Rauch SA, Resnick H, et al. Exploring posttraumatic stress disorder symptom profile among pregnant women. J Psychosom Obstet Gynaecol. 2010;31(3):176–187. doi: 10.3109/0167482X.2010.486453
- Geller PA, Stasko EC. Effect of previous posttraumatic stress in the perinatal period. J Obstet Gynecol Neonatal Nurs. 2017;46(6):912–922. doi: 10.1016/j.jogn.2017.04.136
- Goldstein BL, Briggs-Gowan M, Grasso DJ. The effects of intimate partner violence and a history of childhood abuse on mental health and stress during pregnancy. J Fam Violence. 2021;36(3):337–346. doi: 10.1007/s10896-020-00149-1
- Grasso DJ, Ford JD, Greene CA. Preliminary psychometrics of the structured trauma-related experiences and symptoms screener for adults (STRESS-A) in an urban prenatal healthcare clinic. Psychol Trauma. 2019;11(8):927–935. doi: 10.1037/tra0000476
- Sanjuan PM, Fokas K, Tonigan JS, et al. Prenatal maternal posttraumatic stress disorder as a risk factor for adverse birth weight and gestational age outcomes: a systematic review and meta-analysis. J Affect Disord. 2021;295:530–540. doi: 10.1016/j.jad.2021.08.079
- McEwen BS, Akil H. Revisiting The stress concept: implications for affective disorders. J Neurosci. 2020;40(1):12–21. doi: 10.1523/JNEUROSCI.0733-19.2019
- Glover V. Prenatal stress and its effects on the fetus and the child: possible underlying biological mechanisms. Adv Neurobiol. 2015;10:269–283.
- Graignic-Philippe R, Dayan J, Chokron S, et al. Effects of prenatal stress on fetal and child development: a critical literature review. Neurosci Biobehav Rev. 2014;43:137–162. doi: 10.1016/j.neubiorev.2014.03.022
- Grasso DJ, Drury S, Briggs-Gowan M, et al. Adverse childhood experiences, posttraumatic stress, and FKBP5 methylation patterns in postpartum women and their newborn infants. Psychoneuroendocrinology. 2020;114:104604. doi: 10.1016/j.psyneuen.2020.104604
- Scorza P, Merz EC, Spann M, et al. Pregnancy-specific stress and sensitive caregiving during the transition to motherhood in adolescents. Bmc Pregnancy Childbirth. 2021;21(1):458. doi: 10.1186/s12884-021-03903-5
- Cook N, Ayers S, Horsch A. Maternal posttraumatic stress disorder during the perinatal period and child outcomes: a systematic review. J Affect Disord. 2018;225:18–31. doi: 10.1016/j.jad.2017.07.045
- Yehuda R, Engel SM, Brand SR, et al. Transgenerational effects of posttraumatic stress disorder in babies of mothers exposed to the world trade center attacks during pregnancy. J Clin Endocrinol Metab. 2005;90(7):4115–4118. doi: 10.1210/jc.2005-0550
- Pierrehumbert B, Nicole A, Muller-Nix C, et al. Parental post-traumatic reactions after premature birth: implications for sleeping and eating problems in the infant. Arch Dis Child Fetal Neonatal Ed. 2003;88(5):F400–4. doi: 10.1136/fn.88.5.F400
- Riva Crugnola C, Ierardi E, Ferro V, et al. Mother-infant emotion regulation at three months: the role of maternal anxiety, depression and parenting stress. Psychopathology. 2016;49(4):285–294. doi: 10.1159/000446811
- Katz LF, Gurtovenko K. Posttraumatic stress and emotion regulation in survivors of intimate partner violence. J Family Psychol. 2015;29(4):528–536. doi: 10.1037/fam0000128
- Crandall A, Deater-Deckard K, Riley AW. Maternal emotion and cognitive control capacities and parenting: a conceptual framework. Develop Rev. 2015;36:105–126. doi: 10.1016/j.dr.2015.01.004
- Coppola G, Ponzetti S, Aureli T, et al. Patterns of emotion regulation at two years of age: associations with mothers’ attachment in a fear eliciting situation. Attachment And Human Development. 2016;18(1):16–32. doi: 10.1080/14616734.2015.1109676
- Lewis J, Binion G, Rogers M, et al. The associations of maternal emotion dysregulation and early child dissociative behaviors. J Trauma Dissoc. 2020;21(2):203–216. doi: 10.1080/15299732.2019.1678211
- Lavelli M, Carra C, Rossi G, et al. Culture-specific development of early mother-infant emotional co-regulation: Italian, Cameroonian, and West African immigrant dyads. Dev Psychol. 2019;55(9):1850–1867. doi: 10.1037/dev0000696
- Leerkes EM, Su J, Sommers SA. Mothers’ self-reported emotion dysregulation: a potentially valid method in the field of infant mental health. Infant Ment Health J. 2020;41(5):642–650. doi: 10.1002/imhj.21873
- Jovanovic T, Vance LA, Cross D, et al. Exposure to Violence Accelerates Epigenetic Aging in Children. Sci Rep. 2017;7(1):8962. doi: 10.1038/s41598-017-09235-9
- Horvath S. DNA methylation age of human tissues and cell types. Genome Biol. 2013;14(10):R115. doi: 10.1186/gb-2013-14-10-r115
- Hannum G, Guinney J, Zhao L, et al. Genome-wide methylation profiles reveal quantitative views of human aging rates. Mol Cell. 2013;49(2):359–367. doi: 10.1016/j.molcel.2012.10.016
- Levine ME, Lu AT, Quach A, et al. An epigenetic biomarker of aging for lifespan and healthspan. Aging. 2018;10(4):573–591. doi: 10.18632/aging.101414
- Lu AT, Quach A, Wilson JG, et al. DNA methylation GrimAge strongly predicts lifespan and healthspan. Aging. 2019;11(2):303–327. doi: 10.18632/aging.101684
- Wolf EJ, Logue MW, Hayes JP, et al. Accelerated DNA methylation age: associations with PTSD and neural integrity. Psychoneuroendocrinology. 2016;63:155–162. doi: 10.1016/j.psyneuen.2015.09.020
- Wolf EJ, Logue MW, Stoop TB, et al. Accelerated DNA methylation age: associations with posttraumatic stress disorder and mortality. Psychosom Med. 2018;80(1):42–48. doi: 10.1097/PSY.0000000000000506
- Wolf EJ, Logue MW, Morrison FG, et al. Posttraumatic psychopathology and the pace of the epigenetic clock: a longitudinal investigation. Psychol Med. 2019;49(5):791–800. doi: 10.1017/S0033291718001411
- Katrinli S, Stevens J, Wani AH, et al. Evaluating the impact of trauma and PTSD on epigenetic prediction of lifespan and neural integrity. Neuropsychopharmacology. 2020;45(10):1609–1616. doi: 10.1038/s41386-020-0700-5
- Yang R, GWY W, Verhoeven JE, et al. A DNA methylation clock associated with age-related illnesses and mortality is accelerated in men with combat PTSD. Mol Psychiatry. 2021;26(9):4999–5009. doi: 10.1038/s41380-020-0755-z
- Kuan PF, Ren X, Clouston S, et al. PTSD is associated with accelerated transcriptional aging in World Trade Center responders. Transl Psychiatry. 2021;11(1):311. doi: 10.1038/s41398-021-01437-0
- Zannas AS, Linnstaedt SD, An X, et al. Epigenetic aging and PTSD outcomes in the immediate aftermath of trauma. Psychol Med. 2023;1–10.
- Mehta D, Bruenig D, Pierce J, et al. Recalibrating the epigenetic clock after exposure to trauma: the role of risk and protective psychosocial factors. J Psychiatr Res. 2021;149:374–381. doi: 10.1016/j.jpsychires.2021.11.026
- Bohlin J, Haberg SE, Magnus P, et al. Prediction of gestational age based on genome-wide differentially methylated regions. Genome Biol. 2016;17(1):207. doi: 10.1186/s13059-016-1063-4
- Knight AK, Craig JM, Theda C, et al. An epigenetic clock for gestational age at birth based on blood methylation data. Genome Biol. 2016;17(1):206. doi: 10.1186/s13059-016-1068-z
- Lee Y, Choufani S, Weksberg R, et al. Placental epigenetic clocks: estimating gestational age using placental DNA methylation levels. Aging. 2019;11(12):4238–4253. doi: 10.18632/aging.102049
- Mayne BT, Leemaqz SY, Smith AK, et al. Accelerated placental aging in early onset preeclampsia pregnancies identified by DNA methylation. Epigenomics. 2017;9(3):279–289. doi: 10.2217/epi-2016-0103
- Haftorn KL, Lee Y, Denault WRP, et al. An EPIC predictor of gestational age and its application to newborns conceived by assisted reproductive technologies. Clin Epigenetics. 2021;13(1):82. doi: 10.1186/s13148-021-01055-z
- Suarez A, Lahti J, Czamara D, et al. The epigenetic clock at birth: associations with maternal antenatal depression and child psychiatric problems. J Am Acad Child Adolesc Psychiatry. 2018;57(5):321–28 e2. doi: 10.1016/j.jaac.2018.02.011
- Koen N, Jones MJ, Nhapi RT, et al. Maternal psychosocial risk factors and child gestational epigenetic age in a South African birth cohort study. Transl Psychiatry. 2021;11(1):358. doi: 10.1038/s41398-021-01434-3
- Hammen C, Kim EY, Eberhart NK, et al. Chronic and acute stress and the prediction of major depression in women. Depress Anxiety. 2009;26(8):718–723. doi: 10.1002/da.20571
- McLaughlin KA, Conron KJ, Koenen KC, et al. Childhood adversity, adult stressful life events, and risk of past-year psychiatric disorder: a test of the stress sensitization hypothesis in a population-based sample of adults. Psychol Med. 2010;40(10):1647–1658. doi: 10.1017/S0033291709992121
- Turner RJ, Wheaton B, Lloyd DA. The epidemiology of social stress. Am Sociological Rev. 1995;60(1):104–125. doi: 10.2307/2096348
- McGuire A, Beebe R, Stover C, et al. Structured Trauma-Related Experiences and Symptoms Screener for Adults (STRESS-A): validation in a child welfare sample of mothers and fathers with active domestic violence. Psychol Trauma. 2021; 15(1): 163–172. doi: 10.1037/tra0001029
- Gratz KL, Roemer L. Multidimensional assessment of emotion regulation and dysregulation: development, factor structure, and initial validation of the difficulties in emotion regulation scale. J Psychopathology Behav Assess. 2004;26(1):41–54. doi: 10.1023/B:JOBA.0000007455.08539.94
- Barfield RT, Kilaru V, Smith AK, et al. CpGassoc: an R function for analysis of DNA methylation microarray data. Bioinformatics. 2012;28(9):1280–1281. doi: 10.1093/bioinformatics/bts124
- McCartney DL, Walker RM, Morris SW, et al. Identification of polymorphic and off-target probe binding sites on the Illumina Infinium MethylationEPIC BeadChip. Genom Data. 2016;9:22–24. doi: 10.1016/j.gdata.2016.05.012
- Triche TJ Jr., Weisenberger DJ, Van Den Berg D, et al. Low-level processing of Illumina Infinium DNA Methylation BeadArrays. Nucleic Acids Res. 2013;41(7):e90. doi: 10.1093/nar/gkt090
- Leek JT, Johnson WE, Parker HS, et al. The sva package for removing batch effects and other unwanted variation in high-throughput experiments. Bioinformatics. 2012;28(6):882–883. doi: 10.1093/bioinformatics/bts034
- Zheng SC, Webster AP, Dong D, et al. A novel cell-type deconvolution algorithm reveals substantial contamination by immune cells in saliva, buccal and cervix. Epigenomics. 2018;10(7):925–940. doi: 10.2217/epi-2018-0037
- Li S, Wong EM, Bui M, et al. Causal effect of smoking on DNA methylation in peripheral blood: a twin and family study. Clin Epigenetics. 2018;10:18. doi: 10.1186/s13148-018-0452-9
- Logue MW, Miller MW, Wolf EJ, et al. An epigenome-wide association study of posttraumatic stress disorder in US veterans implicates several new DNA methylation loci. Clinical Epigenetics. 2020;12(1):46. doi: 10.1186/s13148-020-0820-0
- Raffington L, Schwaba T, Aikins M, et al. Associations of socioeconomic disparities with buccal DNA-methylation measures of biological aging. Clin Epigenetics. 2023;15(1):70. doi: 10.1186/s13148-023-01489-7
- Santos Oliveira NC D, Katrinli S, de Assis SG, et al. Community and domestic violence are associated with DNA methylation GrimAge acceleration and heart rate variability in adolescents. Eur J Psychotraumatol. 2023;14(2):2202054. doi: 10.1080/20008066.2023.2202054
- Richmond RC, Sillero-Rejon C, Khouja JN, et al. Investigating the DNA methylation profile of e-cigarette use. Clin Epigenetics. 2021;13(1):183. doi: 10.1186/s13148-021-01174-7
- Zhang Q, Vallerga CL, Walker RM, et al. Improved precision of epigenetic clock estimates across tissues and its implication for biological ageing. Genome Med. 2019;11(1):54. doi: 10.1186/s13073-019-0667-1
- Daredia S, Huen K, Van Der Laan L, et al. Prenatal and birth associations of epigenetic gestational age acceleration in the center for the health assessment of mothers and children of salinas (CHAMACOS) cohort. Epigenetics. 2022;17(13):2006–2021. doi: 10.1080/15592294.2022.2102846
- Knight AK, Smith AK, Conneely KN, et al. Relationship between epigenetic maturity and respiratory morbidity in preterm infants. J Pediatr. 2018;198:168–73 e2. doi: 10.1016/j.jpeds.2018.02.074
- Girchenko P, Lahti J, Czamara D, et al. Associations between maternal risk factors of adverse pregnancy and birth outcomes and the offspring epigenetic clock of gestational age at birth. Clin Epigenetics. 2017;9(1):49. doi: 10.1186/s13148-017-0349-z
- Polinski KJ, Robinson SL, Putnick DL, et al. Epigenetic gestational age and the relationship with developmental milestones in early childhood. Hum Mol Genet. 2023;32(9):1565–1574. doi: 10.1093/hmg/ddac302
- Bozack AK, Rifas-Shiman SL, Gold DR, et al. DNA methylation age at birth and childhood: performance of epigenetic clocks and characteristics associated with epigenetic age acceleration in the project viva cohort. Clin Epigenetics. 2023;15(1):62. doi: 10.1186/s13148-023-01480-2
- Dieckmann L, Lahti-Pulkkinen M, Kvist T, et al. Characteristics of epigenetic aging across gestational and perinatal tissues. Clin Epigenetics. 2021;13(1):97. doi: 10.1186/s13148-021-01080-y
- Katrinli S, Oliveira NCS, Felger JC, et al. The role of the immune system in posttraumatic stress disorder. Transl Psychiatry. 2022;12(1):313. doi: 10.1038/s41398-022-02094-7
- Euclydes V, Gomes C, Gouveia G, et al. Gestational age acceleration is associated with epigenetic biomarkers of prenatal physiologic stress exposure. Clin Epigenetics. 2022;14(1):152. doi: 10.1186/s13148-022-01374-9
- Simpkin AJ, Hemani G, Suderman M, et al. Prenatal and early life influences on epigenetic age in children: a study of mother-offspring pairs from two cohort studies. Hum Mol Genet. 2016;25(1):191–201. doi: 10.1093/hmg/ddv456
- Palma-Gudiel H, Eixarch E, Crispi F, et al. Prenatal adverse environment is associated with epigenetic age deceleration at birth and hypomethylation at the hypoxia-responsive EP300 gene. Clin Epigenetics. 2019;11(1):73. doi: 10.1186/s13148-019-0674-5
- Khouja JN, Simpkin AJ, O’Keeffe LM, et al. Epigenetic gestational age acceleration: a prospective cohort study investigating associations with familial, sociodemographic and birth characteristics. Clin Epigenetics. 2018;10(1):86. doi: 10.1186/s13148-018-0520-1
- Appleton AA, Lin B, Kennedy EM, et al. Maternal depression and adverse neighbourhood conditions during pregnancy are associated with gestational epigenetic age deceleration. Epigenetics. 2022;17(13):1905–1919. doi: 10.1080/15592294.2022.2090657
- Barker DJ. The fetal and infant origins of adult disease. BMJ. 1990;301(6761):1111. doi: 10.1136/bmj.301.6761.1111
- Crimmins EM, Thyagarajan B, Levine ME, et al. Associations of Age, Sex, Race/Ethnicity, and Education with 13 Epigenetic Clocks in a Nationally Representative U.S. Sample: the Health and Retirement Study. J Gerontol A Biol Sci Med Sci. 2021;76(6):1117–1123. doi: 10.1093/gerona/glab016
- Xu K, Li S, Muskens IS, et al. Accelerated epigenetic aging in newborns with Down syndrome. Aging Cell. 2022;21(7):e13652. doi: 10.1111/acel.13652
- Ladd-Acosta C, Vang E, Barrett ES, et al. Analysis of pregnancy complications and epigenetic gestational age of newborns. JAMA Netw Open. 2023;6(2):e230672. doi: 10.1001/jamanetworkopen.2023.0672