abstract
Recently we investigated the function of the 9-lipoxygenase (LOX) derived cyclopentenones 10-oxo-11-phytoenoic acid (10-OPEA) and 10-oxo-11,15-phytodienoic acid (10-OPDA) and identified their C-14 and C-12 derivatives. 10-OPEA accumulation is elicited by fungal and insect attack and acts as a strong inhibitor of microbial and herbivore growth. Although structurally similar, comparative analyses between 10-OPEA and its 13-LOX analog 12-oxo-phytodienoic acid (12-OPDA) demonstrate specificity in transcript accumulation linked to detoxification, secondary metabolism, jasmonate regulation, and protease inhibition. As a potent cell death signal, 10-OPEA activates cysteine protease activity leading to ion leakage and apoptotic-like DNA fragmentation. In this study we further elucidate the distribution, abundance, and functional roles of 10-OPEA, 10-OPDA, and 12-OPDA, in diverse organs under pathogen- and insect-related stress.
Abbreviations
(LOX) | = | lipoxygenase |
(10-OPEA) | = | 10-oxo-11-phytoenoic acid |
(10-OPDA) | = | 10-oxo-11-phytodienoic acid |
(12-OPDA) | = | 12-oxophytodienoic acid |
(JA) | = | jasmonic acid |
(fall armyworm – FAW) | = | Spodoptera frugiperda |
(R) | = | regurgitant |
Cyclopente(a)none oxylipins regulate diverse biological processes. In animals, prostaglandins formed by cylcooxygenases have hormone-like effects that sustain homeostatic functions and mediate pathogen interactions, including inflammation responses.Citation1 Plant cyclopente(a)nones also have important roles in signaling and defense. While distinct functions for various cyclopente(a)nones have been elucidated, the vast majority of plant studies have focused on the 13-lipoxygenase (LOX) product 12-oxo-phytodienoic acid (12-OPDA) and its derivatives, collectively termed jasmonates. A vast number of physiological and genetic analyses have demonstrated diverse roles for jasmonates including developmental processes and inducible defenses against biotic threats.Citation2,3 In maize (Zea mays), genetic evidence highlights roles for jasmonic acid (JA) in defense against biotic threats, senescence, and developmental processes that control male sex determination.Citation4,5 As an essential jasmonate precursor, 12-OPDA can trigger development and defense signaling distinct from JA itself or its bioactive amino acid conjugate (+)-7-iso-jasmonoyl-L-isoleucine.Citation6,7,8,9 During physiological stress in Arabidopsis, free 12-OPDA binds cyclophilin 20–3 to promote the formation of a cysteine synthase complex leading to elevated glutathione levels and cellular redox homeostasis.Citation9 In general, 12-OPDA signaling is consistent with a role in cell protection and inhibition of cell death.Citation10
In addition to the 13-LOX jasmonate pathway, 9-LOX genes also function in plant development and plant-biotic interactions. Specific 9-LOXs have been shown to influence cell death,Citation11,12,13 root growth,Citation14 senescence,Citation15 and to promote either susceptibilityCitation16 or resistanceCitation17,15,18 to pathogens and pests in host-species interactions. Despite evidence linking 9-LOX genes to important biological roles, many of the specific metabolites that promote these functions have remained elusive. 10-oxo-11-phytoenoic acid (10-OPEA) and 10-oxo-11,15-phytodienoic acid (10-OPDA) have been long considered candidates for biologically active compounds given their similarity to jasmonates ().Citation19,13 First isolated from potato (Solanum tuberosum), 10-OPEA and 10-OPDA were identified as products of 9-allene oxide synthase enzymes and subsequent non-enzymatic cyclization, resulting in racemic mixtures of low yield stereoisomers.Citation19,20 In contrast, our empirical findings in maize silksCitation13 and a concurrent study done in maize rootsCitation21 showed that cis-10-OPEA synthesis in maize is consistent with full enzymatic biosynthesis, yielding predominantly (9S,13S)-10-OPEA as a result of an as-yet unidentified enzyme with novel 9-AOC activity. Together, these results suggest pathway diversity in 9-cyclopentenone formation between dicot and monocot species.
Figure 1. Proposed model for the biosynthesis of the 9- and 13-lipoxygenase derived cyclopentenones 10-oxo-11-phytoenoic acid (10-OPEA), 10-oxo-11,15-phytodienoic acid (10-OPDA), and 12-oxo-10,15-phytodienoic acid (12-OPDA) and their respective derivatives (modified from Christensen et al.Citation13). Black dashed arrows indicate the presence of predicted enzyme activities requiring future confirmation. Abbreviations are as follows: lipoxygenase (LOX), allene oxide synthase (AOS), linolenic acid (18:3), linoleic acid (18:2), 9-hydroperoxy-10,12-octadecadienoic acid (9-HPOD), 9-hydroperoxy-10,12,15- octadecatrienoic acid (9-HPOT), 13-hydroperoxy-10,12,15-octadecatrienoic acid (13-HPOT); 9,10-epoxyoctadecatrienoic acid (9,10-EOT); 9,10-epoxyoctadecadienoic acid (9,10-EOD); 12,13-epoxyoctadecatrienoic acid (12,13-EOT); 3-oxo-2-(2′-pentenyl)-cyclopentane-1-octanoic acid (OPC-8:0), and hexanoic acid (OPC-6:0), DA0-4:1, (4-[(1,5)-2-oxo-5-pentylcyclopent-3-ene-1-yl] hexanoic acid; DA0-4:0, 4-[(1,5)-2-oxo-5-pentylcyclopent-3-ene-1-yl] butanoic acid; DA0-2:0, (2-[(1, 5)-2-oxo-5-pentylcyclopent-3-ene-1-yl] acetic acid; DA1-4:0, 4-[(1,5)-2-oxo-5-[(2)-pent-2-en-1-yl]clyclopentyl] butanoic acid; DA1-2:0, 2-[(1,5)-2-oxo-5-[(2)-pent-2-en-1-yl]clyclopentyl] acetic acid.
![Figure 1. Proposed model for the biosynthesis of the 9- and 13-lipoxygenase derived cyclopentenones 10-oxo-11-phytoenoic acid (10-OPEA), 10-oxo-11,15-phytodienoic acid (10-OPDA), and 12-oxo-10,15-phytodienoic acid (12-OPDA) and their respective derivatives (modified from Christensen et al.Citation13). Black dashed arrows indicate the presence of predicted enzyme activities requiring future confirmation. Abbreviations are as follows: lipoxygenase (LOX), allene oxide synthase (AOS), linolenic acid (18:3), linoleic acid (18:2), 9-hydroperoxy-10,12-octadecadienoic acid (9-HPOD), 9-hydroperoxy-10,12,15- octadecatrienoic acid (9-HPOT), 13-hydroperoxy-10,12,15-octadecatrienoic acid (13-HPOT); 9,10-epoxyoctadecatrienoic acid (9,10-EOT); 9,10-epoxyoctadecadienoic acid (9,10-EOD); 12,13-epoxyoctadecatrienoic acid (12,13-EOT); 3-oxo-2-(2′-pentenyl)-cyclopentane-1-octanoic acid (OPC-8:0), and hexanoic acid (OPC-6:0), DA0-4:1, (4-[(1,5)-2-oxo-5-pentylcyclopent-3-ene-1-yl] hexanoic acid; DA0-4:0, 4-[(1,5)-2-oxo-5-pentylcyclopent-3-ene-1-yl] butanoic acid; DA0-2:0, (2-[(1, 5)-2-oxo-5-pentylcyclopent-3-ene-1-yl] acetic acid; DA1-4:0, 4-[(1,5)-2-oxo-5-[(2)-pent-2-en-1-yl]clyclopentyl] butanoic acid; DA1-2:0, 2-[(1,5)-2-oxo-5-[(2)-pent-2-en-1-yl]clyclopentyl] acetic acid.](/cms/asset/9d5df682-10eb-4f68-a253-8d937ba48a95/kpsb_a_1120395_f0001_b.gif)
In a recent study, we initiated the functional characterization of 10-OPEA, 10-OPDA and derived C14 and C12 metabolites, collectively termed death acids.Citation13 10-OPEA is inducible at the local site of pathogen and insect attack and has antimicrobial and herbivore growth inhibitor activity. The capacity of 10-OPEA as a plant defense signal/metabolite is similar to 12-OPDA, yet it demonstrates specificity, with only 60% overlap in the co-expression of over 5000 genes differentially expressed in response to these 2 conceptually related oxylipins. One distinction from 12-OPDA activity is the weak ability of 10-OPEA to promote accumulation of transcripts coding for protease inhibitors. Furthermore, exogenous 10-OPEA application strongly induces cysteine protease activation and programmed cell death, as evidenced by immunoblot protease labeling, lesion development, ion leakage, and DNA fragmentation.Citation13 Collectively these results suggest that 9- and 13-LOX-derived cyclopentenones can have distinct and separate functions, despite their structural similarities. To further elucidate and contrast the established roles of 12-OPDA in insect related defense responses, and the more specific role of 10-OPEA in fungal-infected tissues, we examined the induction of 9-LOX and 13-LOX derived cyclopentenones in additional maize tissues under varied stress conditions.
To explore 9- and 13-LOX derived cyclopentenone production in maize in response to pathogen and insect attack, we first infected the inner-whorl leaf tissue and developing upper node and internode stem tissue with Cochliobolus heterostrophus (Southern Leaf Blight – SLB) and measured 10-OPEA, 10-OPDA, and 12-OPDA over a 3 day time course. At 24 h post-inoculation, 10-OPEA concentrations in inner-whorl leaf tissues were significantly higher than 10-OPDA or 12-OPDA and remained higher over the duration of the time course (). Similar results were observed in node/internode stem tissues, collectively indicating a bias toward 10-OPEA production in both leaf and developing node/internode tissues. Previous work has routinely demonstrated that all of these metabolites are near or at the limits of detection in healthy untreated control tissues.Citation13 To mimic insect feeding, regurgitant (R) from Spodoptera frugiperda (fall armyworm – FAW) was applied to fully expanded maize leaves. Basal levels of 12-OPDA were significantly higher than 10-OPEA and 10-OPDA with 3- and 10-fold differences, respectively (). By 1 h post treatment, FAW-R had elicited a strong 13-LOX response with 12-OPDA levels exceeding 10-OPEA and 10-OPDA production by >65 fold, demonstrating a strong preference for the jasmonate pathway in response to herbivory on mature leaves.
Figure 2. Treatment dependent elicitation of 9- and 13- cylcopentenones in leaf and node/internode tissue. (A) Average (n = 4, ± SEM) concentrations (µg g−1 FW) of 10-OPEA (black), 10-OPDA (light gray) and 12-OPDA (dark gray) in maize interior whorl and node/internode tissue 1, 2, and 3 d following SLB inoculation. (Insert) Average (n = 3, ± SEM) concentrations (µg g−1 FW) of 10-OPEA, 10-OPDA and 12-OPDA in fully expanded maize leaves in response to no treatment (NT), damage (DAM), and fall armyworm regurgitant (FAW-R) 1 hour post-application. The limits of detection for 10-OPEA, 10-OPDA, and 12-OPDA are at 10pg/µl (All ANOVA P< 0.05, Tukey test corrections for multiple comparisons).
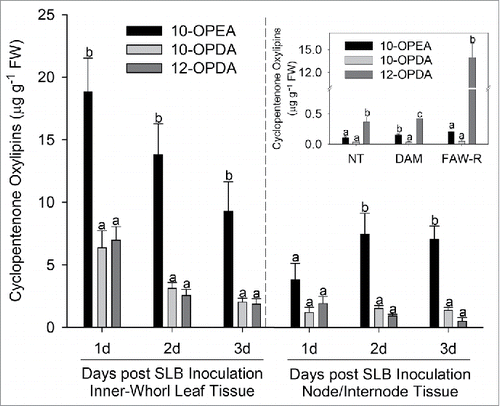
Both insect feeding and pathogen attack can occur on diverse maize organs and cause the damage-induced collapse of cells. To build upon our previous results showing abundant levels of 9-LOX cyclopentenones in the silksCitation13 and to better understand the capacity of different organs to produce 10-OPE(D)A and 12-OPDA, hydroponic root and silk tissues were crush-damaged and metabolites were measured over a time course. Both hydroponic roots and silks displayed a rapid induction of 10-OPEA upon damage, producing approximately 3 and 40ug/g FW within 20 min, respectively, and sustaining high levels for at least 120 min (). Both 10-OPDA and 12-OPDA were at least 2-fold less than 10-OPEA in response to crush-damage and remained significantly lower throughout both time courses. Together, these results demonstrate that 10-OPEA is the predominant LOX-derived cyclopentenone in silks and roots in response to cell damage.
Figure 3. 10-OPEA is the predominant LOX-derived cyclopentenone in wounded silks and roots. (A) Average (n = 4, ± SEM) 10-OPEA, 10-OPDA, and 12-OPDA (µg g−1 FW) levels from crush damaged silks (Zea mays hybrid ‘Golden Queen’) harvested 0, 5, 15, 45, 60, 90 and 120 min post damage. (B) Crush damage-induced levels of 10-OPEA, 10-OPDA, and 12-OPDA in hydroponic roots of 2 wk old plants (n = 4, ± SEM) at 0, 20, 60, 90, 180, and 360 min post treatment. In silk and root treatment plots, different letters (a-b) represent significant differences at 120 min (All ANOVA P < 0.05, Tukey test corrections for multiple comparisons).
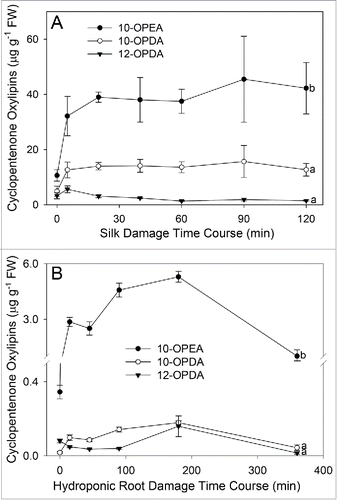
The maize scutellum is an important developmental organ in early maize ontogeny, acting to absorb, store, and transfer nutrients from the endosperm to the growing seedling while preventing microbial infections.Citation22 To determine the presence of 10-OPE(D)A and 12-OPDA during seedling growth, we harvested scutella in developing seedlings over a 14 d time course. Ten d post-planting, 10-OPEA accumulation was >30- and 190-fold above that of 10-OPDA and 12-OPDA, respectively, and 40- and 300-fold higher by 14 d (). Cell death, as measured by ion leakage, increased throughout seedling growth and corresponded to the progressive accumulation of 10-OPEA(), indicating a strong and positive monotonic correlation between 10-OPEA production and programmed cell death (rs = 0.77; p < 0.001). These data suggest that 10-OPEA is developmentally regulated and that it may play a role in scutella senescence. It is also possible that 10-OPEA levels increase in the scutella in response to soil-borne microbes that attack the dying tissue.
Figure 4. 10-OPEA is strongly induced and shows a positive correlation with ion leakage in maize scutella. Average (n = 4, ± SEM) 10-OPEA, 10-OPDA, and 12-OPDA (µg g−1 FW) levels in scutella during the first 14 d of seedling development. (Insert) Scatter plot with bidirectional error bars showing percent ion leakage and 10-OPEA increases over the 14 d time course. Different letters (a-b) represent significant differences (All ANOVA P < 0.05, Tukey test corrections for multiple comparisons).
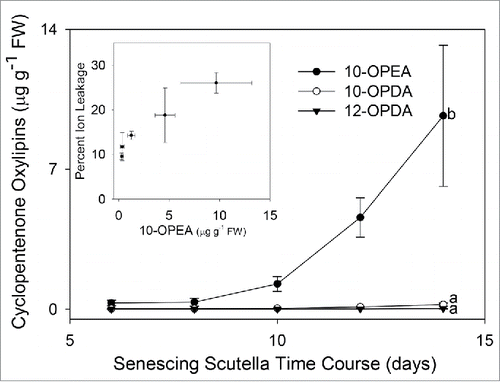
While many studies have investigated the biosynthesis and function of 13-LOX derived cyclopentenones such as 12-OPDA, surprisingly little is known about the 9-LOX derived jasmonate-like analogs 10-OPEA and 10-OPDA. We recently demonstrated that 10-OPEA is abundantly and predominantly produced during SLB infection and can function both as a direct plant defense and transcriptionally active signal. In comparative analyses between 9- and 13-LOX derived cyclopentenones, we observed a measurable divergence in function with 10-OPE(D)A displaying significantly heightened cell death promoting activity. To better understand aspects of distribution and abundance, and to gain insight into additional functions, we investigated the production of 10-OPEA, 10-OPDA, and 12-OPDA in several maize organs under diverse treatments. In response to SLB-infection, we observed that 10-OPEA is significantly more abundant than 12-OPDA in both inner-whorl leaf and developing stem node/internode tissues. This bias toward 10-OPEA production was somewhat predictable as transcript levels for the candidate 9-LOXs responsible for 10-OPEA biosynthesis (ZmLOX3, ZmLOX4, and ZmLOX5) are induced to higher levels during SLB infection than the 13-LOX genes that regulate jasmonate production (ZmLOX8 and ZmLOX10).Citation13,23 Differences in accumulation between 10-OPEA and the two 18:3 derived cyclopentenones may also be due to substrate availability, as 18:3 levels are approximately 2-3 fold lower than 18:2 under SLB infection conditions.Citation13 Interestingly, 12-OPDA is produced at much higher levels than 10-OPE(D)A in FAW-R treated leaves. Although 10-OPEA was previously shown to be inducible in corn ear worm infested inner-whorl tissues,Citation13 its production does not significantly increase in fully expanded leaves in response to FAW-R (P = 0.352), indicating that insect-induced 10-OPEA in leaves may be specific to particular developmental states of leaf tissue.
Large differences in cyclopentenone production were observed in other maize organs including silks and roots, as 10-OPEA levels significantly exceeded those of 10- and 12-OPDA. The physiological and ecological role for above as well as below ground organs to accumulate 10-OPEA is likely due to enhanced specificity for activating pathogen defenses. For example, maize silks facilitate pathogenicity for spores of airborne ear rotting pathogens such as Aspergillus flavus and Fusarium verticillioides.Citation24,25 Consistent with previous work demonstrating the significance of maize silks in pathogen defense,Citation25 10-OPEA has strong antimicrobial activity against both A. flavus and F. verticillioides.Citation13 Current efforts to generate null mutants in the 10-OPEA biosynthesis pathway will allow the functional role of 10-OPEA in maize defense against biotic threats to be tested genetically.
This study addresses the production of the 9- and 13-LOX derived cyclopentenones 10-OPEA, 10-OPDA, and 12-OPDA, in multiple organs under different biotic and developmental conditions. While these findings broaden our understanding of 10-OPEA, 10-OPDA, and 12-OPDA abundance, distribution, and function, many questions remain. For example, what are the biological functions of 10-OPEA and 10-OPDA in direct defense and defense signaling against a broad range of pathogens and insects? And, what is the occurrence and function of 10-OPEA and 10-OPDA in other monocot and dicot species? Critical to these questions will be the development of effective genetic tools that eliminate or overproduce these metabolites in planta.
Methods
Hybrid maize (Zea mays var. Golden Queen) growth conditions for fungal infections, crush-damage treatments, and ion leakage were carried out using methods previously described.Citation13 FAW-R treatment was performed with application of 10µl FAW-RCitation26 on the 3rd fully expanded leaf of V-3 stage plants grown in a Conviron E15 (Pembina, ND, USA) growth chamber controlled at 28°C day/25°C night at a 12 h photoperiod. The collection of scutella was done as outlined in Schmelz et al.Citation27 For separation, identification, and quantification of the cyclopentenones described in this work, samples were solvent extracted, methylated, collected on a polymeric adsorbent using vapor-phase extraction (VPE), and analyzed by GC/isobutane CI-MS as previously described.Citation27 For the broad screening of known and unknown compounds, total ion collection was performed, followed by extractive ion collection for the analysis of individual metabolites. The limits of detection for the cyclopentenones measured in this study are at or slightly below 10pg/µl. Metabolite quantification was based on U-13C-18:3 (Cambridge Isotope Laboratories, Inc., Tewksbury, MA, USA), as an internal standard.
Disclosure of potential conflicts of interest
No potential conflicts of interest were disclosed.
Acknowledgments
Many thanks to Dawn Diaz-Ruiz, Erika Friman, Steve Willms, Bevin Forguson and Maritza Romero for their technical support. Research was funded by US Department of Agriculture (USDA)–Agricultural Research Service Projects 6615-21000-010-00 / 6615-22000-027-00, and by NSF Division of Integrative Organismal Systems Competitive Award 1139329.
References
- Ricciotti E, FitzGerald GA. Prostaglandins and inflammation. Arterioscler Thromb Vasc Biol 2011; 31:986-1000; PMID:21508345; http://dx.doi.org/10.1161/ATVBAHA.110.207449.
- Farmer EE, Almeras E, Krishnamurthy V. Jasmonates and related oxylipins in plant responses to pathogenesis and herbivory. Curr Opin Plant Biol 2003; 6:372-8; PMID:12873533; http://dx.doi.org/10.1016/S1369-5266(03)00045-1.
- Wasternack C, Hause B. Jasmonates: biosynthesis, perception, signal transduction and action in plant stress response, growth and development. An update to the 2007 review in Annals of Botany. Ann Bot 2013; 111:1021-58; PMID:23558912; http://dx.doi.org/10.1093/aob/mct067.
- Acosta IF, Laparra H, Romero SP, Schmelz E, Hamberg M, Mottinger JP, Moreno MA, Dellaporta SL. tasselseed1 Is a Lipoxygenase Affecting Jasmonic Acid Signaling in Sex Determination of Maize. Science 2009; 323:262-5; PMID:19131630; http://dx.doi.org/10.1126/science.1164645.
- Yan Y, Christensen S, Isakeit T, Engelberth J, Meeley R, Hayward A, Emery RJN, Kolomiets MV. Disruption of OPR7 and OPR8 Reveals the Versatile Functions of Jasmonic Acid in Maize Development and Defense. Plant Cell 2012; 24:1420-36; PMID:22523204; http://dx.doi.org/10.1105/tpc.111.094151.
- Stintzi A, Weber H, Reymond P, Browse J, Farmer EE. Plant defense in the absence of jasmonic acid: The role of cyclopentenones. Proc Natl Acad Sci U S A 2001; 98:12837-42; PMID:11592974; http://dx.doi.org/10.1073/pnas.211311098.
- Mueller S, Hilbert B, Dueckershoff K, Roitsch T, Krischke M, Mueller MJ, Berger S. General detoxification and stress responses are mediated by oxidized lipids through TGA transcription factors in Arabidopsis. Plant Cell 2008; 20:768-85; PMID:18334669; http://dx.doi.org/10.1105/tpc.107.054809.
- Fonseca S, Chini A, Hamberg M, Adie B, Porzel A, Kramell R, Miersch O, Wasternack C, Solano R. (+)-7-iso-Jasmonoyl-L-isoleucine is the endogenous bioactive jasmonate. Nat Chem Biol 2009; 5:344-50; PMID:19349968; http://dx.doi.org/10.1038/nchembio.161.
- Park S-W, Li W, Viehhauser A, He B, Kim S, Nilsson AK, Andersson MX, Kittle JD, Ambavaram MMR, Luan S, et al. Cyclophilin 20-3 relays a 12-oxo-phytodienoic acid signal during stress responsive regulation of cellular redox homeostasis. Proc Natl Acad Sci U S A 2013; 110:9559-64; PMID:23671085; http://dx.doi.org/10.1073/pnas.1218872110.
- Thoma I, Loeffler C, Sinha AK, Gupta M, Krischke M, Steffan B, Roitsch T, Mueller MJ. Cyclopentenone isoprostanes induced by reactive oxygen species trigger defense gene activation and phytoalexin accumulation in plants. Plant J 2003; 34:363-75; PMID:12713542; http://dx.doi.org/10.1046/j.1365-313X.2003.01730.x.
- Montillet JL, Chamnongpol S, Rusterucci C, Dat J, van de Cotte B, Agnel JP, Battesti C, Inze D, Van Breusegem F, Triantaphylides C. Fatty acid hydroperoxides and H2O2 in the execution of hypersensitive cell death in tobacco leaves. Plant Physiol 2005; 138:1516-26; PMID:15980200; http://dx.doi.org/10.1104/pp.105.059907.
- van der Linde K, Hemetsberger C, Kastner C, Kaschani F, van der Hoorn RA, Kumlehn J, Doehlemann G. A maize cystatin suppresses host immunity by inhibiting apoplastic cysteine proteases. Plant Cell 2012; 24:1285-300; PMID:22454455; http://dx.doi.org/10.1105/tpc.111.093732.
- Christensen SA, Huffaker A, Kaplan F, Sims J, Ziemann S, Doehlemann G, Ji L, Schmitz RJ, Kolomiets MV, Alborn HT, et al. Maize death acids, 9-lipoxygenase-derived cyclopente(a)nones, display activity as cytotoxic phytoalexins and transcriptional mediators. Proc Natl Acad Sci U S A 2015; 112:11407-12; PMID:26305953; http://dx.doi.org/10.1073/pnas.1511131112.
- Vellosillo T, Martinez M, Lopez MA, Vicente J, Cascon T, Dolan L, Hamberg M, Castresana C. Oxylipins produced by the 9-lipoxygenase pathway in Arabidopsis regulate lateral root development and defense responses through a specific signaling cascade. Plant Cell 2007; 19:831-46; PMID:17369372; http://dx.doi.org/10.1105/tpc.106.046052.
- Gao X, Starr J, Goebel C, Engelberth J, Feussner I, Tumlinson J, Kolomiets M. Maize 9-lipoxygenase ZmLOX3 controls development, root-specific expression of defense genes, and resistance to root-knot nematodes. Mol Plant-Microbe Interact 2008; 21:98-109; PMID:18052887; http://dx.doi.org/10.1094/MPMI-21-1-0098.
- Gao X, Shim WB, Gobel C, Kunze S, Feussner I, Meeley R, Balint-Kurti P, Kolomiets M. Disruption of a maize 9-lipoxygenase results in increased resistance to fungal pathogens and reduced levels of contamination with mycotoxin fumonisin. Mol Plant Microbe Interact 2007; 20:922-33; PMID:17722696; http://dx.doi.org/10.1094/MPMI-20-8-0922.
- Weber H, Chetelat A, Caldelari D, Farmer EE. Divinyl ether fatty acid synthesis in late blight-diseased potato leaves. Plant Cell 1999; 11:485-94; PMID:10072406.
- Hwang IS, Hwang BK. The Pepper 9-Lipoxygenase Gene CaLOX1 Functions in Defense and Cell Death Responses to Microbial Pathogens. Plant Physiol 2010; 152:948-67; PMID:19939946; http://dx.doi.org/10.1104/pp.109.147827.
- Hamberg M. New cyclopentenone fatty acids formed from linoleic and linolenic acids in potato. Lipids 2000; 35:353-63; PMID:10858019; http://dx.doi.org/10.1007/s11745-000-532-z.
- Itoh A, Schilmiller AL, McCaig BC, Howe GA. Identification of a jasmonate-regulated allene oxide synthase that metabolizes 9-hydroperoxides of linoleic and linolenic acids. J Biol Chem 2002; 277:46051-8; PMID:12351632; http://dx.doi.org/10.1074/jbc.M207234200.
- Ogorodnikova AV, Gorina SS, Mukhtarova LS, Mukhitova FK, Toporkova YY, Hamberg M, Grechkin AN. Stereospecific biosynthesis of (9S,13S)-10-oxo-phytoenoic acid in young maize roots. Biochim Biophys Acta 2015; 1851:1262-70; PMID:26008579; http://dx.doi.org/10.1016/j.bbalip.2015.05.004.
- Kiesselbach TA. The structure and reproduction of corn. Cold Spring Harbor laboratory press: Cold Spring Harbor, 1949.
- Christensen SA, Nemchenko A, Borrego E, Murray I, Sobhy IS, Bosak L, DeBlasio S, Erb M, Robert CA, Vaughn KA, et al. The maize lipoxygenase, ZmLOX10, mediates green leaf volatile, jasmonate and herbivore-induced plant volatile production for defense against insect attack. Plant J 2013; 74:59-73; PMID:23279660; http://dx.doi.org/10.1111/tpj.12101.
- Marsh S, Payne, G. Preharvest infection of corn silks and kernels by Aspergillus flavus. Phytopathology 1984; 74:1284-9; http://dx.doi.org/10.1094/Phyto-74-1284.
- Campos-Bermudez VA, Fauguel CM, Tronconi MA, Casati P, Presello DA, Andreo CS. Transcriptional and metabolic changes associated to the infection by Fusarium verticillioides in maize inbreds with contrasting ear rot resistance. PloS One 2013; 8:e61580; PMID:23637860; http://dx.doi.org/10.1371/journal.pone.0061580.
- Schmelz EA, Engelberth J, Alborn HT, Tumlinson JH, 3rd, Teal PE. Phytohormone-based activity mapping of insect herbivore-produced elicitors. Proc Natl Acad Sci U S A 2009; 106:653-7; PMID:19124770; http://dx.doi.org/10.1073/pnas.0811861106.
- Schmelz EA, Kaplan F, Huffaker A, Dafoe NJ, Vaughan MM, Ni X, Rocca JR, Alborn HT, Teal PE. Identity, regulation, and activity of inducible diterpenoid phytoalexins in maize. Proc Natl Acad Sci U S A 2011; 108:5455-60; PMID:21402917; http://dx.doi.org/10.1073/pnas.1014714108.