ABSTRACT
Soil salinization is a major cause of plant stress, partly due to the physicochemical similarities between Na+ and K+. Na+ ions compete with K+ ions for their transport into root cells. However, the point of Na+ entry remains unidentified. Here, I have applied the Electrical Penetration Graph as a method for whole plant electrophysiology in order to test if (a) root exposure to NaCl induces depolarization waves that propagate from root to shoot via the phloem, and if (b) the electrophysiological effects of root exposure to NaCl require expression of the potassium channels AKT1 and/or AKT2. The data suggest that AKT2 subunit containing K+ channels mediate NaCl-induced depolarization of root cells, and that this depolarization does not propagate to leaves via the phloem.
Abbreviations
EPG | = | Electrical Penetration Graph |
SE | = | Sieve element |
CC | = | Companion cell |
Land plants are chronically exposed to many environmental variables. Some, like temperature, vary gradually in intensity over circadian and seasonal time scales. Others, like herbivorous insects, do not follow predictable temporal patterns. Salinity is a complex environmental factor to which land plants have been exposed for millions of years, and it currently represents a major threat to agriculture.Citation1 NaCl is a particularly dangerous salt; Na+ competes with K+ for its transport into root cells, but the ion channel(s) through which it enters remain(s) unidentified". Ion channels that catalyze the influx of K+ ions into the cytoplasm, known as inward rectifiers, are good candidates as points of entry for Na+ ions. Two important plant inward rectifiers are AKT1 and AKT2. AKT1 is involved in the uptake of K+ into the root.Citation2,3 The weak inward rectifier AKT2Citation4 is expressed in the phloem thorough the entire plant,Citation4-6 and additionally in mesophyll cells, where it accounts for half of the K+ permeability.Citation3 In addition to its effect on root cell physiology, soil salinity may be communicated to the shoot via electrical waves. In fact, application of salt shocks (100 mM NaCl) to the root of Arabidopsis seedlings was found to induce Ca2+ waves that spread to the shoot and involved the participation of endodermal and cortex cells.Citation7 These results beg the question of whether salt-induced, root-to-shoot electrical signals are propagated via the phloem. Here, I asked whether root exposure to NaCl induces electrical waves that are transmitted to the leaves via the phloem, and whether AKT1 and/or AKT2 are involved in the electrophysiological effects of root exposure to NaCl. For comparative purposes, I also examined electrophysiological responses of root exposure to salt in the aquatic fern Azolla filiculoides. In this study, I have implemented the Electrical Penetration Graph, or EPG,Citation8 which measures potential differences between a root area containing thousands of cells, and a single sieve element/companion cell (SE/CC) complex, usually in a leaf (). Salt was applied during the E2 feeding phase of EPG-recorded aphids, in which the aphid stylet is stably inserted into a SE/CC complex to be used as an intracellular electrode.Citation8 shows representative depolarization responses of an Arabidopsis and an Azolla plant to root application of increasing concentrations of NaCl. While 1 mM NaCl induced small depolarizations that spontaneously returned to baseline within 1 or 2 minutes, concentrations of 10 mM or greater induced sustained depolarizations that lasted for several minutes after reaching a steady-state, which were reversible by washout (). The onset of these depolarizations was immediate after salt application - within 1s - and they rose slowly, taking c. 20s to peak. In most cases, a brief hyperpolarization preceded the depolarization onset. shows average maximum levels of NaCl induced depolarization for A. thaliana and A. filiculoides as a function of NaCl concentration. Application of 100 mM KCl induced depolarizations similar to 100 mM NaCl, and were also reversible by washout (). The root, rather than the SE/CC complex sampled by the aphid stylet, is the most likely origin of these depolarizations, because they started immediately after root salt application. The sustained depolarizations recorded with external coarse electrodes would correspond to the summed electrical activity of thousands of cells within a volume of excitable tissue,Citation9 in this case root tissue. Perhaps that the molecular determinant(s) for the salt-induced depolarizations are shared between ancient and modern plants, given the similarities of the responses root NaCl application between Azolla and Arabidopsis.
Figure 1. The Electrical Penetration Graph (EPG) rig for recording salt-induced electrical signals in plants. (A) General configuration of the EPG, as set up for this study. The EPG measures the potential difference between a fine intracellular electrode specific for the SE/CC complex (represented by the aphid stylet), and a coarse external copper electrode inserted into the root medium, in this case an aqueous medium. (B) Diagram showing the recording horizons (not to scale) of the intracellular and external EPG electrodes. Since the electrode's recording horizon is positively correlated with the electrode diameter,Citation16 it is reasonable to assume that the large EPG soil electrode (Ø ∼1.5 mm) (electrode B) must be able to detect the electrical activity arising from potentially several cell layers of the area(s) of the root in close proximity, whereas the aphid stylet (electrode A), senses the electrical potential within a small area within the SE/CC complex into which it is inserted – through its watery food and salivary canals with diameters of c. 1 and 0.5 µm, respectively. Electrode A feeds into the non-inverting input of the EPG operational amplifier, whereas electrode B feeds into the inverting input. In this manner, the output of the EPG is the difference between the potentials sensed by electrodes A and B, after reversing the sign of the potential recorded by electrode B. A signal can originate from a potential generated by ionic currents within the recording horizon of electrode A, electrode B, or both.
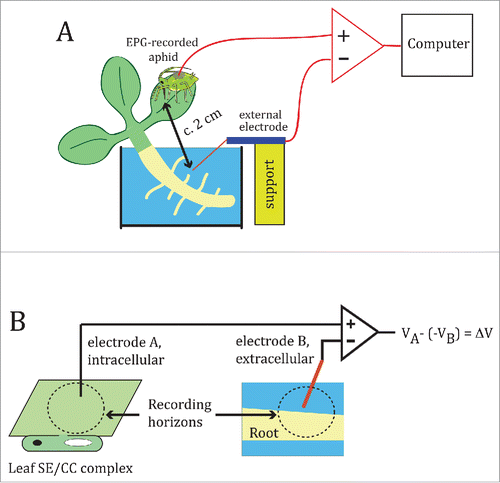
Figure 2. Electrophysiological responses of wild type Arabidopsis thaliana and Azolla filiculoides to root salt application. (A, B) Electrical potentials generated between the SE/CC-intracellular electrode and the root-external electrode of the EPG, in response to application of increasingly higher concentrations of NaCl (1, 10, 50 and 100 mM) in an A. thaliana (A) and in an A. filiculoides plant (B). Before applying 10, 50 or 100 mM NaCl, the effects of the previous salt concentration were washed out by replacing the NaCl solution with distilled water, as shown in (C). (D), Comparison between the averaged maximum depolarizations of A. thaliana and A. filiculoides as a function of NaCl concentration. Data points represent mean ± s.e.m. Numbers in parentheses indicate the number of plants per NaCl concentration. (E), Electrical potential triggered by application of 100 mM KCl to the root of A. thaliana, and its reversibility by washout.
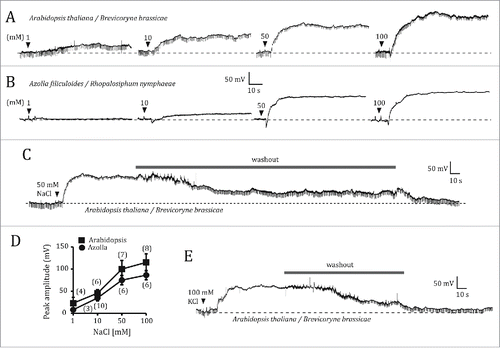
Next, I tested the potential role of two inward K+ rectifiers, AKT1 and AKT2, as entry points for Na+ into the root cells. The electrophysiological responses of akt1 plants to NaCl root application were similar to those of wild type plants. Immediately upon NaCl application, a brief hyperpolarization was followed by a slowly rising depolarization whose maximum values were not statistically different from those of wild type plants (). In contrast, akt2 mutant plants did not depolarize in response to NaCl concentrations up to 50 mM, and only minimally in response to 100 mM NaCl. Small and transient hyperpolarizations were, however, present in all of the akt2 recordings (). Although the origin of this hyperpolarization is not clear, the fact that it is transient, i.e. returning to the baseline level spontaneously during the presence of NaCl, suggests a biological origin, rather than a polarizing effect of the copper electrode caused by the salt. Since the NaCl solutions were applied manually, the effect of small mechanical disturbances must be taken into consideration. It is thus conceivable that the transient hyperpolarization was caused by mechanically activated ion channels, as is the case with other excitable tissues.Citation10 shows averaged maximum depolarization levels for wild type, akt1 and akt2 plants, as a function of NaCl concentration. The average maximal depolarizations induced by 50 or 100 mM NaCl in akt1 and akt2 plants were statistically significantly different (P = 0.0029 for 50 mM; P = 0.0001 for 100 mM).
Figure 3. Electrophysiological responses of akt1 and akt2 mutants of Arabidopsis thaliana to NaCl root application. Electrical potentials induced by 50 mM NaCl to the root of akt1 (A) and akt2 (B). (C), Plot of the averaged maximum depolarization levels as a function of NaCl concentration for the wild type, and the akt1 and akt2 mutants. Data points represent mean ± s.e.m. Numbers in parentheses indicate the number of plants per NaCl concentration. Only one NaCl concentration per plant was tested. Whenever plants were exposed to several NaCl concentrations, a given NaCl solution was only applied after the effects of the previous NaCl solution had been completely washed out by manual solution exchange.
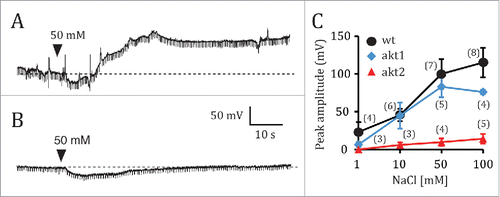
On the basis of the results presented here, I propose that Na+ is taken up in root cells via K+ channels containing AKT2 subunits, and that AKT2 is required for Na+ uptake. Interestingly Ca2+ has a protective effect against salinization,Citation11 and is known to inhibit inward rectifying K+ channels.Citation12,13 It is possible that AKT2 mediates the protective effect of Ca2+ against salinity.
Materials and methods
Plants and growth conditions
Wild type Arabidopsis thaliana L. Col-0 and homozygous akt1 and akt2 Ws-0 plants were used. Arabidopsis plants were grown under long day conditions (16 hrs light, 8 hrs dark; 21°C) on sand and watered with Hyponex plant food solution twice per week, after attaining 2 weeks of age. Before conducting the subsequent the experiments, they were carefully extracted and immersed in distilled water in order to remove all sand. Then they were placed in dishes, with the roots submerged in tap water. The Azolla filiculoides Lam. plants were collected from outdoor pools at Plant Research International (Wageningen). They were maintained in a glasshouse at 21° C without supplemental lighting in floating culture in a beaker with tap water.
Aphids
Clones of the cabbage aphid Brevicoryne brassicae L. were reared on Brassica oleracea L. in a glasshouse at Wageningen University (Netherlands) without supplemental lighting, under long day conditions (16 hrs light, 8 hrs dark; 21°C). Rhopalosiphum nymphaeae L. aphids were collected in a pool in the Wageningen area, and maintained on Lemna minor L. in a glasshouse at 21°C without supplemental lighting in floating culture in a beaker with tap water. For EPG recordings, R. nymphaeae aphids were transferred from Lemna to Azolla plants on the day of the experiment as I found that no acclimation period was necessary.
Electrophysiology
The Electrical Penetration Graph (EPG) technique was re-purposed as a method for recording systemic electrical signals in plants, as described previously.Citation8 Aphid probing was recorded with a Giga-8 amplifier (8-channel DC system; EPG Systems, Netherlands). The aphid, the plant, and the EPG pre-amplifier were set inside a Faraday cage. Aphids for EPG recording were attached to a gold wire (φ18 μm) by means of a water-based glue droplet containing silver particles, as originally described.Citation14,15 Signals were acquired at a sampling rate of 100 Hz, digitized with a built-in converter, and analyzed using Stylet+ software (EPG Systems, Netherlands) on a PC. Since the input resistance (Ri) of the DC-EPG amplifier was 1GΩ, the measured voltage differences were calibrated with a pulse of known voltage (in this case, 50 mV), in order to compensate for the attenuation of the membrane potential changes due to the relatively low Ri. The EPG-recorded aphids were positioned on the midvein at the leaf/petiole junction of leaf 3 or 4 in the case of Arabidopsis, or on a peripheral scale-like leaf in the case of Azolla. The extracellular copper electrode was placed within the aqueous medium surrounding the root.
Data analyses
Statistical analyses were performed with GraphPad software. Data are given as mean ± s.e.m. Statistical differences between means were determined with the Student t test for independent samples. The level of statistical significance was set at P < 0.05.
Disclosure of potential conflicts of interest
No potential conflicts of interest were disclosed.
Acknowledgments
I would like to thank Adrie van der Werf for supplying R. nymphaeae aphids and A. filiculoides plants, Ingo Dreyer for the donation of akt1 and akt2 mutant seeds, Joop van Loon for providing laboratory facilities at the University of Wageningen, Henriette Schluepmann for providing office space at Utrecht University, Michael Laing for editorial help, and Michael Thorpe for helpful discussions. This research was supported by crowd funding through www.experiment.com.
References
- Dasgupta S, Hossain MM, Huq M, Wheeler D. Climate change and soil salinity: The case of coastal Bangladesh. Ambio 2015; 44:815-26; PMID:26152508; http://dx.doi.org/10.1007/s13280-015-0681-5
- Hirsch RE, Lewis BD, Spalding EP, Sussman MR. A role for the AKT1 potassium channel in plant nutrition. Science 1998; 280:918-21; PMID:9572739; http://dx.doi.org/10.1126/science.280.5365.918
- Dennison KL, Robertson WR, Lewis BD, Hirsch RE, Sussman MR, Spalding EP. Functions of AKT1 and AKT2 potassium channels determined by studies of single and double mutants of Arabidopsis. Plant Physiol 2001; 127:1012-9; PMID:11706182; http://dx.doi.org/10.1104/pp.010193
- Cao Y, Ward JM, Kelly WB, Ichida AM, Gaber RF, Anderson JA, Uozumi N, Schroeder JI, Crawford NM. Multiple genes, tissue specificity, and expression-dependent modulation contribute to functional diversity of potassium channels in Arabidopsis thaliana. Plant Physiol 1995; 109:1093-106; PMID:8552711; http://dx.doi.org/10.1104/pp.109.3.1093
- Lacombe B, Pilot G, Michard E, Gaymard F, Sentenac H, Thibaud JB. A shaker-like channel with weak rectification is expressed in both source and sink phloem tissues of Arabidopsis. Plant Cell 2000; 12:837-52; PMID:10852932
- Marten I, Hoth S, Deeken R, Ache P, Ketchum KA, Hoshi T, Hedrich R. AKT3, a phloem-localized K+ channel, is blocked by protons. Proc Nat Acad Sci USA 1999; 96:7581-6; PMID:10377458; http://dx.doi.org/10.1073/pnas.96.13.7581
- Choi WG, Toyota M, Kim SH, Hilleary R, Gilroy S. Salt stress-induced Ca2+ waves are associated with rapid, long-distance root-to-shoot long signalling in plants. Proc Nat Acad Sci USA 2014; 111:6497-502; PMID:24706854; http://dx.doi.org/10.1073/pnas.1319955111
- Salvador-Recatalà V, Tjallingii WF, Farmer EE. Real-time, in vivo intracellular recordings of caterpillar–induced depolarization waves in sieve elements using aphid electrodes. New Phytol 2014; 203:674-84; http://dx.doi.org/10.1111/nph.12807
- Carter M, Shie JC. Guide to research techniques in neuroscience. Academic Press, Elsevier Inc., London 2010
- Terakawa S, Watanabe A. Electrical responses to mechanical stimulation of the membrane of squid giant axons. Pflugers Arch 1982; 395:59-64; PMID:7177772; http://dx.doi.org/10.1007/BF00584969
- Zhong H, Läuchli A. Spatial distribution of solutes, K, Na, Ca and their deposition rates in the growth zone of primary cotton roots: effects of NaCl and CaCl2. Planta 1994; 194:34-41; http://dx.doi.org/10.1007/BF00201032
- Schroeder JI, Ward JM, Gassmann W. Perspective on the physiology and structure of inward-rectifying K+ channels in higher plants: Biophysical implications for K+ uptake. Ann Rev Biophys Biomol Struct 1994; 23:441-71; http://dx.doi.org/10.1146/annurev.bb.23.060194.002301
- Latz A, Ivashikina N, Fischer S, Ache P, Sano T, Becker D, Deeken R, Hedrich R. In planta AKT2 subunits constitute a pH- and Ca2+ -sensitive inward rectifying K+ channel. Planta 2007; 225:1179-91; PMID:17146665; http://dx.doi.org/10.1007/s00425-006-0428-4
- McLean DL, Kinsey MG. A technique for electronically recording aphid feeding and salivation. Nature 1964; 202:1358-9; http://dx.doi.org/10.1038/2021358a0
- Tjallingii WF. Electronic recording of penetration behavior by aphids. Entomol Exp Appl 1978; 24:721-30; http://dx.doi.org/10.1111/j.1570-7458.1978.tb02836.x
- Lin Y, Chen C, Chen L, Zeng S, Luo Q. The analysis of electrode-recording horizon. Conf Proc IEEE Eng Med Biol Soc 2005; 7:7345-8; PMID:17281977