ABSTRACT
Rice growth and productivity is adversely affected by low-temperature stress. From a previous screen of diverse rice genotypes for cold tolerance parameters at the vegetative stage, we selected the tolerant Nipponbare and M202 genotypes and sensitive Cypress and Secano do Brazil genotypes for further analysis at the reproductive stage for physiological and yield parameters. Cold stress severely affected grain yield as estimated by the number of grain per panicle, panicle length, and 100 seed weight. Analysis of gene expression of 21 genes involved in physiological responses to low temperature tested, in the flag leaf and inflorescence tissue of these genotypes, showed an increased expression of the Lipid Transfer Protein genes LTP7 and LTP10 in flag leaf tissue of the tolerant Nipponbare and M202, along with a significant increase in the relative expression of stress-responsive transcription factors (TFs) and cold-inducible genes. In flag leaf tissue OsNAC9, OsNAC10 and OsNAP genes showed high correlation with photosynthesis, stomatal conductance, transpiration and Quantum Efficiency of PSII. In consequence of the foregoing results, we conclude that Nipponbare and M202 are cold tolerant genotypes and that LTP7, LTP10, OsNAC9, OsNAC10 and OsNAP genes can be used as markers in screening for cold tolerance at the reproductive stage. Furthermore based on the results we propose a model of low-temperature tolerance mechanism of how stress is perceived, and how the signal cascade acts to promote tolerance at below-ideal temperatures.
Introduction
Plant growth and productivity are adversely affected by extreme temperatures, including low-temperature stress. Environmental stress caused by sub-optimal temperatures categorized as cooling or freezing, plays an essential role in determining the geographical distribution for cultivation and growth response of plants.
Reduction in photosynthesis and stomatal conductivity, accumulation of metabolites such as proline or glycine betaine, and alterations in carbohydrate metabolism, as well as the accumulation of reactive oxygen species (ROS), are typical symptoms of physiological responses of plants to stress caused by low temperatures.Citation1,Citation2 Previous studies have shown the existence of ABA-dependent and independent response pathways in response to sub-optimal temperatures, which coordinate the expression of inducible genes such as COR, CBF, Zip, WRKY, LEA, NAC, NAP, iSAP and others.Citation3-Citation12
The survival of plants at low temperature involves two distinct mechanisms, tolerance and cold acclimatization. The tolerance to cooling or low temperatures (0° to 15°C), can be defined as the intrinsic ability of plants to survive under these conditions without injury or damage.Citation13 Acclimatization at suboptimal temperatures is characterized by the increased potential of plants to tolerate changes generated in the physicochemical composition of the cells, producing osmolytes such as soluble sugars and alcohols, as well as the nitrogenous compounds proline and glycine betaine.Citation14,Citation15 Moreover, the accumulation of osmolytes and ROS induced by stress caused by this environmental condition leads to the induction of Cold Regulated (CORs) proteins, dehydrins, as well as TFs such as AREBs or ABFs that contribute to stress tolerance through the ABA-dependent pathway.Citation16-Citation18 These stress-induced proteins lead to the removal of reactive oxygen species, the stabilization of membrane phospholipids and eventually maintenance of ionic homeostasis.Citation16 Innate tolerance at low temperatures is recognized as a complex response that involves the regulation of metabolic and morphological adaptive pathways, including proteins involved in signaling, gene expression and control of repair to stress damage.
Rice is considered as the most important crop, for feeding more than half of the world population. However, despite its economic importance, rice production is restricted by its sensitivity to abiotic stresses such as low temperatures at all stages of development, due to structural features such as a small root system and lower wax deposition.Citation19 During the course of its agricultural expansion, continuous selection and breeding efforts have led to the selection of abiotic stress tolerant rice genotypes. Although some rice genotypes have evolved to be tolerant to drought, salinity and other stresses, and though they have a low yield, can serve as excellent genetic resources for the improvement of stress tolerance in rice.
The symptoms of low temperature-induced damage are expressed at different times of development, such as at germination, seedling, vegetative, reproductive and grain maturation stages.Citation20 Stress caused by sub-optimal temperatures affects seed germination, increases the seedling period, impairing development and photosynthetic capacity, inducing leaf chlorosis and reduction of plant height.Citation19 The sensitive reproductive stage is an important and limiting factor for rice cultivars that eventually results in a significant reduction of production potential.Citation21
Treatment of rice plants to a low temperature at the reproductive stage results in spikelet sterility and abortion, or the incomplete exertion of the panicle. Spikelet sterility as a consequence of low-temperature stress is a result of the abortion of pollen after microsporogenesis. Other symptoms of lesions induced by suboptimal temperatures include abnormalities in anther development, as well as grain spotting, and late or incomplete ripening.
In this study, four rice genotypes with contrasting response to cold identified from a previous study on cold stress at the vegetative growth stage,Citation22 were evaluated for the level of tolerance and sensitivity to cold stress caused by low temperatures at the reproductive stage and analyzed using physiological and productivity parameters. In addition, changes in gene expression of the flag leaf and inflorescence were characterized to study the association with the phenotypic response parameters in response to the stress treatments.
Materials and methods
Reproductive stage cold stress treatment
Rice genotypes Nipponbare (tolerant), M202 (tolerant), Cypress (sensitive) and Secano do Brazil (sensitive) were selected based on data collected from a previous screening experiment.Citation22 Seed were germinated and individual seedlings transplanted into 500 ml plastic pots, containing commercial potting soil substrate (Redi Earth). All genotypes were grown in the greenhouse (26/22°C ± 1°C with a photon flux density of 600 μmol−2s−1, light/dark cycle of 14/10 h), and the pots placed in trays full of water simulating flooded conditions. Fertilization was done using the commercial product Miracle-Gro (Scotts Miracle-Gro Products) for the entire experiment.Citation23 When plants reached the reproductive stage (flowering -R2 stage of pre-anthesis) at around 80 days, with flowering synchronized by staggered planting of the four genotypes, they were divided into two batches of 20 pots each, five per genotype. A batch was kept under optimal conditions with well-irrigated water as a control, while another set of trays was used for the low-temperature treatment, at sub-optimal cold temperature stress, at 10°C for 96 h.
Photosynthesis and photochemical efficiency of PSII were measured on the second fully expanded leaf using a portable photosynthesis meter (LI-6400XT; LI-COR) at a CO2 concentration of 370 μmolmol -1, the photon flux density of 1,000 μ mol m-2 s-1 and 55% to 60% relative humidity. For molecular analyses of the flag leaf and panicle, tissue of flag leaf and panicle were collected and stored at -80oC and analysis of grain yield done using the methodology of Ambavaram et al.Citation24 The experimental design used was a completely randomized design, and the data were used for analysis of variance (ANOVA) and Student’s t-test using a 1% level of significance, using the SAS 9.3 statistical program (SAS Institute, Cary, NC).
Analysis of gene expression
Total RNA was extracted from the rice genotypes using Trizol reagent (Invitrogen), and cDNA was synthesized from 2 μg of total DNAseI-treated RNA using the GoScript™ reverse transcription system (Promega). The RT-qPCR reactions were performed using a GoTaq® qPCR Master Mix (Promega) kit with Ubiquitin as the internal reference geneCitation22 in the CFX-96 thermal cycler (Bio-Rad). A temperature gradient (0.5° C 10 s -1) from 55°C to 95°C was used for the analysis of the melting curve, and untranscribed RNA was used as a negative control. The relative expression differences for each of the experimental samples were determined from the normalization of Ct values for each gene relative to the Ct value of Ubiquitin and the fold change experimental expression variation is calculated using the equation (2)−ΔΔCt.Citation25 Expression analysis was performed with three biological replicates and three technical replicates, with the genes used described in Table 1 (Supplementary data). Relative quantification of the expression of each gene was obtained as described by Liviak and Schmittgen,Citation25 the data used for analysis of variance (ANOVA) and Student’s t-test (at 1%) using the statistical software SAS 9.3 (SAS Institute, Cary, NC), and the results were imported into TM4 microarray software to generate a heat-map.Citation26 To analyze the correlation between the expression of each gene with phenotypic photosynthetic and yield response parameters, the Pearson correlation coefficient was calculated for each tissue and represented in a table (Supplementary Table 2). The analyses were performed using the SAS v.9.3 software (SAS Institute Inc., Cary, NC.)
Results
Effects of cold stress on physiological parameters
Rice genotypes exposed to suboptimal cold-stress temperatures at the reproductive stage showed a significant reduction in photosynthesis ()) in Secano do Brazil (12.37–3.69) and Cypress (16.91–4.99) which showed a reduction of >70%, while Nipponbare (19.98–12.97) and M202 (18.69–11.73) showed a reduction of ~35%. A similar pattern of reduction among genotypes was also observed for stomatal conductance ()). The analysis of water use efficiency, a measure that estimates the ratio of photosynthesis to transpiration, showed a significant reduction in Cypress and Secano do Brazil while M202 and Nipponbare showed an increase of 58% and 13.34%, respectively ()). To study the effect of low temperatures on chlorophyll fluorescence, Fv’/Fm’ was measured, and verified that Cypress and Secano do Brazil showed a more significant reduction ()), while Nipponbare and M202 were also reduced, but with lower photo-damage.
Figure 1. Physiological parameters of rice genotypes in response to low temperature.
Response of plant genotypes treated at control (28°C) and stress (10°C) conditions, show response for: (a) photosynthesis, (b) stomatal conductance, (c) transpiration and (d) PSII quantum efficiency. The data represent the mean of five biological replicates. The asterisks indicate significance at P ≤ 0.01 compared to the control as analyzed by Student’s t-test.
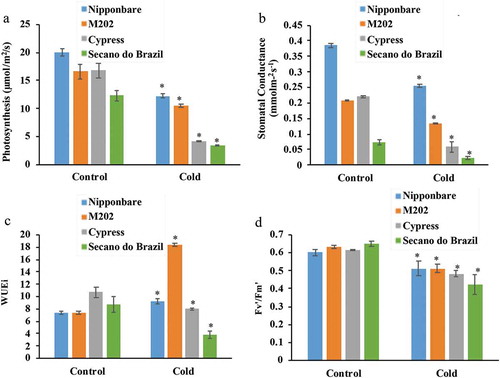
Effects of cold stress on yield parameters
At reproductive stage under cold stress, spikelet sterility was high showing 80% and 90%, for the cold-sensitive Secano do Brazil and Cypress, respectively, with Nipponbare and M202 showing more tolerance with a lower level of around 38–42% (). Subsequently, cold stress severely affected the grain yield as estimated by a number of grains per panicle and 100 seed weight for Cypress and Secano do Brazil, which were less reduced by cold for Nipponbare and M202 (–)).
Figure 2. Yield parameters evaluated for different rice genotypes treated to low temperature.
Plants treated to control (28°C) and cold stress (10°C) showed response in: (a) Panicle length, (b) Spikelet sterility, (c) Number of grains/panicle, and (d) Grain weight. Data are expressed as the mean of five replications. The asterisks indicate significance at P ≤ 0.01 compared to the control as analyzed by Student’s t-test.
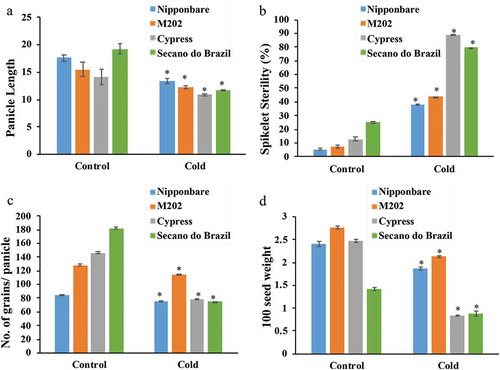
Gene expression of lipid transfer proteins (LTPs) in response to cold stress
Lipid Transfer Proteins have been shown to be differentially expressed under cold stress,Citation27 and their expression was characterized here (). The flag leaf of cold tolerant Nipponbare and M202 (compared to Cypress and Secano do Brazil) showed induced expression of OsLTP7 and OsLTP10, and Nipponbare additionally for OsLTP-12, -14 and -25 genes, and M202 inflorescence additionally for OsLTP-8, -12, and -14 genes with constitutive expression of OsLTP8 in flag leaf of all genotypes. The sensitive genotype Cypress showed induction in inflorescence tissue for OsLTP-7, -8, -10, -12, and -14, and sensitive Secano do Brazil induction in infloresence tissue for OsLTP-8, -10, -12, -14 thus partly overlapping with M202. For the low temperature induced LTP (OsDIL), no expression was observed in the Cypress and Secano do Brazil flag leaf or inflorescence tissue, whereas in Nipponbare and M202 the expression was higher in both tissues ().
Cold stress-responsive expression of other abiotic stress-inducible genes
The TFs bZIP23 and bZIP46 show cold induction/expression in the inflorescence of Nipponbare and M202, but not induced in Cypress, and bZIP46 induced in sensitive Secano Do Brazil. For OsLea3 inflorescence induction was observed for all except Cypress. The Os DIL and OsGRAS23 genes showed higher expression in Nipponbare and M202 and less expression in the sensitive genotypes for both tissues. Members of the NAC family such as OsNAC5, OsNAC9 and OsNAC10 showed different expression patterns in both tissues. The OsNAC9 gene showed higher expression in Nipponbare, M202 and Cypress, while OsNAC10 had higher expression in Nipponbare and M202 for both tissues, and Secano do Brazil showed moderate expression in the inflorescence. OsNAC5 showed higher expression in the inflorescence of Nipponbare, followed by Secano do Brazil, while no detectable expression change was observed for M202 and Cypress, with a slight induction on cold stress for Nipponbare and M202 inflorescence. OsNAP, showed good expression in inflorescence tissue of all the genotypes, in contrast to flag leaf where only Nipponbare showed increased expression.
A cold-inducible expression pattern was observed for OsABAOX3, OsBURP16 and OsWRKY47 in Nipponbare and M202, for both the tissues with little or no detectable fold-change for Cypress and Secano do Brazil. However, OsWRKY47 showed an increased expression in the inflorescence tissue of Cypress following cold stress treatment ().
Correlation of gene expression to physiological responses
The correlations between physiological parameters and gene expression in flag leaf and inflorescence under cold stress were calculated (Supplementary Table 2 and 3), providing an insight into the mechanisms for the stress responses. The NAC gene family expression showed a high correlation with photosynthetic parameters and 100 seed weight, and with genes OsGRAS23 and OsCIPK23, suggesting a regulatory role of the NAC transcription factor family. In panicle tissue, the OsNAC5 gene showed a good correlation with OsCIPK23, OsGRAS23 and OsbZIP46. OsNAC9 showed a high correlation with photosynthetic parameters and with the genes OsGRAS23 and OsNAC5, but not for the inflorescence. OsNAC10 showed a correlation with physiological parameters and with the genes OsGRAS23 and OsNAC5 showing the same pattern. For the panicle, a high correlation was found with genes OsCIPK23, OsDIL, OsGRAS23, OsbZIP23, OsLea3, OsNAC5, OsiSAP8, and OsbZIP46. OsNAP, another TF belonging to the NAC family, showed correlation with physiological parameters and gene expression of OsNAC10. However, for OsNAP, other correlations were also observed in panicle tissue for physiological parameters, and with genes OsGRAS23, OsbZIP23, OsCIPK23, OsNAC5 and OsNAC10.
The correlation analysis of gene expression in flag leaf with physiological parameters and grain yield-related components is shown in Supplementary data (Table 2). For OsLTP7, in flag leaf, we observed a high correlation with some physiological parameters and with the genes OsNAC10, OsNAC5, OsNAP, OsNAC9, OsCIPK23 and OsGRAS23. In the inflorescence, we found correlations with yield parameters and with OsWRKY47. For the gene OsLTP8, we did not find good correlations in flag leaf. However, for inflorescence tissue (Supplementary data Table 3) the best correlation was observed with OsBURP16. In the flag leaf, OsLTP10showed positive correlations with physiological parameters, followed by the genes OsSKIPa, OsGRAS23, OsBURP16, OsABAOX3, OsNAC5 and OsDIL. However, in inflorescence, OsLTP10 just showed good correlation with OsLTP7. In flag leaf, the OsLTP12 also did not show high correlations. OsLTP25 had correlations with OsLea3, and in inflorescence tissue, we got good values only in OsLTP14. The LTP gene OsDIL showed moderate correlation to a few yield parameters in flag leaf, but in inflorescence tissue, the values are higher for all photosynthetic parameters. In addition, we also observed correlations with OsLea3, OsbZIP23, OsCIPKY23 and OsbZIP46.
Discussion
Photosynthesis is one of the early physiological processes to be affected when plants are subjected to environmental factors unfavorable to growth and development such as drought, high salinity and low atmospheric temperatures.Citation28 Plants exposed to low temperatures show increased membrane permeability, inhibition of chlorophyll biosynthesis and chloroplast damage. Thus, the difference in the ability of plants to cope with photosynthetic damage is attributed to genetic variation and to the stage of development.Citation29 In this study, photosynthesis was quantified in response to stress caused by suboptimal temperatures in four rice genotypes with previously identified contrasting response patterns. In addition, physiological responses as well as changes in yield parameters were found correlated with changes in gene expression.
It was observed that the accumulation of transcripts for lipid transfer proteins, TFs and other low-temperature inducible genes are directly related to the ability of rice genotypes to deal with the adverse effects of stress caused by suboptimal temperatures. Rice genotypes subjected to low temperatures at the reproductive stage showed a significant reduction in photosynthesis, especially in genotypes Cypress and Secano do Brazil. In addition, low temperature had a major impact on stomatal conductance ()), which corroborated with the yield losses observed in all genotypes treated with this stress condition (), with the tolerant having a better efficient use of water compared to the sensitive ones ()). Previously, it was demonstrated that Fv’/Fm’ reflected a significant impact on PSII, suggesting a photoinhibitory effect.Citation30 Here we observed that stress caused by suboptimal temperatures significantly affected chlorophyll fluorescence ()), which is consistent with previous results.
Plant lipid transfer proteins (LTP) are a group of highly conserved proteins of around 9 kDa found in plant tissues. LTPs are responsible for the changes in phospholipids and other groups of fatty acids present in cell membranes. LTPs are also involved in cutin biosynthesis, pathogen defense reactions, and adaptation to environmental changes.Citation31 Previously, it was shown that LTPs are differentially expressed in response to cold stress in different rice genotypes.Citation22,Citation27 Therefore, the role of LTPs was further investigated in response to low-temperature stress, for four rice genotypes in different tissues (flag leaf and inflorescence). The OsLTP7, OsLTP8, OsLTP10, OsLTP12, OsLTP14 and OsLTP25 genes are differentially expressed in different tissues in response to cold stress (). Nipponbare and M202 showed higher expression of OsLTP7 and OsLTP10 in flag leaf tissue, while Cypress and Secano do Brazil showed very low transcript accumulation. All the OsLTP genes (7, 8, 10, 12, 14 and 25) in Nipponbare showed low expression in inflorescence tissue. For M202, Cypress and Secano do Brazil different levels of expression were observed in inflorescence tissue.
When plants are subjected to low temperatures, a signaling cascade comprising TFs and cold-inducible genes are induced, initiating the survival mechanism under stress. A significant increase in relative expression for all TFs and cold-inducible genes was observed, and this increase occurred in the tissues of the tolerant Nipponbare and M202, but very low in Cypress and Secano do Brazil. Previously it has been shown that the NAC family (SNAC) genes are expressed in different tissues and participate in various developmental programs, including floral development,Citation32 cell division,Citation33 lateral root development,Citation4 senescence,Citation9,Citation34,Citation35 secondary cell wall synthesis,Citation36-Citation39 abioticCitation40,Citation41 and biotic stress responses.Citation40-Citation42
OsNAP is a NAC family member that confers stress tolerance in rice by functioning as a transcriptional activator. OsNAP, on overexpression in rice, confers tolerance to drought, cold and low temperature as shown by reduced loss of water, as well as improved yield under drought.Citation3 Transgenic rice and Arabidopsis plants over-expressing OsNAC5 show tolerance to abiotic stresses, supporting that tolerance is achieved by regulating the expression of downstream targets of the gene and resulting in solute accumulation and elimination of peroxide radicals.Citation6 Similar results have been obtained for transgenic rice lines overexpressing OsNAC9 and OsNAC10 that showed enhanced drought resistance with an increase in root diameter.Citation12,Citation43 We observed that the expression of OsNAC9, OsNAC10 and OsNAP in flag leaf showed a high correlation with photosynthesis, stomatal conductance, transpiration and quantum efficiency of PSII, which is consistent with previous observations.
The TF superfamily WRKY, another broad class of TFs, play key roles in the complex signaling that measures plant responses to biotic and abiotic stresses.Citation44 Previously, OsWRKY47 has been proposed to confer tolerance to water-deficit stress response by regulating the expression of genes associated with the inhibition of stress-induced senescence.Citation5 Though, OsWRKY47 did not show high correlation of gene expression in leaf with PS (0.562) and PL (0.718), but showed high correlation with OsbZIP46 (0.824). The bZIP TF family consists of a large number of TFs, with diverse roles and ability to bind to abscisic acid- (ABA) responsive elements (ABREs), and also be associated with increased tolerance of plants to other abiotic stresses. OsbZIP46 showed good expression in inflorescence tissue for Nipponbare and Secano do Brazil, and also a high correlation with PL (0.757). On analyzing OsbZIP46, Tang et al.Citation8, found that this is a positive regulator of ABA signaling and drought stress tolerance in rice. These results are in accordance with the observation by Xiang et al.Citation7, where they analyzed OsbZIP23 and identified it as the “major player” of the bZIP family that confers drought and salinity stress tolerance by the ABA-dependent pathway. Further, Dey et al.Citation45, identified OsbZIP23 as a transcriptional activator of the genes OsRab16B, OsRab21, and OsLEA3-1 in rice. Previously, it has also been found that OsRab16A and members of the dehydrin family are inducible by low-temperature stress in rice.Citation26 OsLEA3-2, when overexpressed in rice results in drought tolerance and high salinity. Though LEA proteins have been postulated to be associated with a mechanism of desiccation tolerance,Citation11 the data from this work did not show expression of OsLEA3-2 in the flag leaf for any of the genotypes. In the inflorescence, expression was observed in Nipponbare, M202 and Secano do Brazil. Moreover, it showed a positive correlation with OsbZIP23 (0.740) and OsbZIP46 (0.943), which is consistent with previous observations where it has been shown that the tolerance mediated by these TFs is mediated by the ABA-dependent pathway that in turn regulates the LEA genes.
The SAP family has Zinc Finger A20/ANI domains that confer response to abiotic stresses, and overexpression in rice and tobacco showed increased tolerance to salt, drought and cold.Citation46 In this work, OsiSAP8 showed high correlation with photosynthetic parameters and with the genes OsbZIP23, OsbZIP46 and OsLEA3-2. The expression of OsGRAS23 showed high correlation with photosynthetic parameters and with the genes OsDIL and OsCIPK23, which is consistent with previous observations by Tian et al.Citation47 This demonstrates further that members of the GRAS gene family encode transcriptional regulators that have diverse functions in plant growth and development such as gibberellin signal transduction, root radial patterning, axillary meristem formation, phytochrome A signaling and gametogenesis. The OsSKIPa protein is a positive regulator for drought and salt stress in rice, which promotes tolerance to environmental stress and facilitates cellular adaptations, increasing the elimination of reactive oxygen species. In our data, we find OsSKIPa is expressed in inflorescence tissue with higher expression in the cold tolerant Nipponbare.
ABA is a phytohormone that plays a key role in various steps of plant growth and development and it is the largest inducer of stress-related signaling. OsABAOX3 is a key enzyme for ABA catabolism and also plays a key role in the germination of rice seeds.Citation48 In the present work, a higher expression of the gene was observed in the inflorescence of tolerant Nipponbare, with a correlation above 0.9. It was also observed that for the same tissue the genes OsCIPK23, OsNAC10, OsiSAP8, OsNAC5, OsbZIP46, OsGRAS23 and OsDIL had a high correlation, indicating that the product of OsABAOX3 can perturb various cell signaling pathways. In the leaf, the transcription of OsBURP16 is induced by cold, salinity and drought stresses, as well as by ABA treatment.Citation49 In this work, OsBURP16 showed correlations with OsNAC5, OsNAC9, OsNAC10, and OsNAP in flag leaf expression. This observation was made on the flag leaf of Nipponbare and M202, while the inflorescence of all genotypes showed increased expression. These data are opposite to those reported by Liu et al.Citation50, where they show that the OsBURP16 gene is an indicator of sensitivity to abiotic stress at the vegetative stage of the rice cultivar Zhonghua 10 (japonica).
A model for low-temperature tolerance mechanisms () was created and proposed as a step towards an improvement in the understanding of how stress is perceived by plant cells, and how the regulatory cascade of signals act to promote tolerance to suboptimal low temperatures. Stress is perceived by cells through molecular signals, which in turn, induce concomitant expression of multiple genes (cited in the text). They act in the perception and protection against damage caused by suboptimal temperatures generating responses that may, for example, increase the activity of antioxidant and osmoprotector enzymes, which results in higher tolerance to stress.
Figure 4. Model for mechanism of tolerance to low temperatures at the reproductive stage in rice. Regulatory cascade of perception and induction of damage in response to cold stress, shown by genes responsive to this condition (colored green) and regulation of downstream genes, as well as the induction of biochemical responses leading to tolerance to low temperatures.
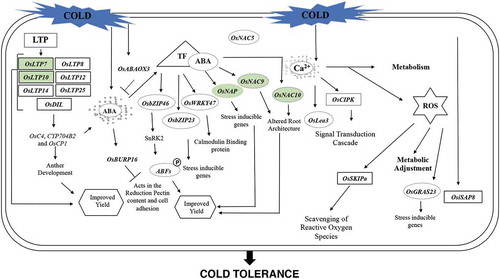
The results of this work support the identification of Nipponbare and M202 as genotypes with relative tolerance to low temperatures, showing less reduction in yield under stress caused by suboptimal temperatures. Further, gene expression analyses also showed that some genes are specifically expressed in the inflorescence tissue. In addition, the significant correlation in expression between genes and with physiological and performance parameters, enabled the prediction of the roles of these genes in coordinated control of downstream gene expression, and therefore aided in the prediction of stress response phenotypes. Finally, some genes (OsLTP7, OsLTP10, OsNAC9, OsNAC10 and OsNAP) have been identified that can be used as markers in screening for low-temperature tolerance at the reproductive stage.
Author contribution statement
GPMF, SB, and AP designed and carried out the research at the University of Arkansas. GPMF, JT, VR and SB performed the analyses of the physiological gas-exchange parameters, yield and gene expression. LCB and EJBB guided research on cold colerance in rice. GPMF analyzed data and together with AP wrote the manuscript. All authors read and approved the manuscript.
Disclosure of Potential Conflicts of Interest
No potential conflicts of interest were disclosed.
Compliance with ethical standards
Conflict of interest: The authors declare that they have no conflict of interest.
Supplemental Material
Download PDF (201.3 KB)Acknowledgments
This work was conducted during a scholarship supported by the International Cooperation Program CAPES/COFECUB at the Federal University of Pelotas Financed by CAPES – Brazilian Federal Agency for Support and Evaluation of Graduate Education within the Ministry of Education of Brazil. Research at the University of Arkansas laboratory was supported by a grant of the National Science Foundation (NSF-MCB 1716844).
Supplementary material
Supplemental data for this article can be accessed here
Additional information
Funding
References
- Farooq M, Wahid A, Kobayashi ND, Fujita SMA. Review article plant drought stress : effects, mechanisms and management. Agron Sustain Dev. 2009;29:185–212. doi:10.1051/agro.
- Bartels D, Sunkar R. Drought and salt tolerance in plants. CRC Crit Rev Plant Sci. 2005;24:23–58. doi:10.1080/07352680590910410.
- Chen X, Zhang J, Liu Q, Guo, W., Zhao, T., Ma, Q., Wang, G. Transcriptome sequencing and identification of cold tolerance genes in hardy Corylus species (C. heterophylla fisch) floral buds. PLoS One. 2014. doi:10.1371/journal.pone.0108604.
- Guo H-S. MicroRNA directs mRNA cleavage of the transcription factor NAC1 to downregulate auxin signals for Arabidopsis lateral root development. Plant Cell Online. 2005;17:1376–1386. doi:10.1105/tpc.105.030841.
- Raineri J, Wang S, Peleg Z, Blumwald E, Chan RL. The rice transcription factor OsWRKY47 is a positive regulator of the response to water deficit stress. Plant Mol Biol. 2015;88:401–413. doi:10.1007/s11103-015-0329-7.
- Song S-Y, Chen Y, Chen J, Dai X-Y, Zhang W-H. Physiological mechanisms underlying OsNAC5-dependent tolerance of rice plants to abiotic stress. Planta. 2011;234:331–345. doi:10.1007/s00425-011-1403-2.
- Xiang Y, Tang N, Du H, Ye H, Xiong L. Characterization of OsbZIP23 as a key player of the basic leucine zipper transcription factor family for conferring abscisic acid sensitivity and salinity and drought tolerance in rice. Plant Physiol. 2008;148:1938–1952. doi:10.1104/pp.108.128199.
- Tang N, Zhang H, Li X, Nishizawa K, Ishimoto M, Arisaka F, Takagi K, Urade R. Constitutive activation of transcription factor OsbZIP46 improves drought tolerance in rice. Plant Physiol. 2012;158:1755–1768. doi:10.1104/pp.111.190389.
- Guo Y, Gan S. AtNAP, a NAC family transcription factor, has an important role in leaf senescence. Plant J. 2006;46:601–612. doi:10.1111/j.1365-313X.2006.02723.x.
- Bevilacqua CB, Basu S, Pereira A, Tseng T-M, Zimmer PD, Burgos NR, Baisakh N. Analysis of stress-responsive gene expression in cultivated and weedy rice differing in cold stress tolerance. PLoS One. 2015;10:1–22. doi:10.1371/journal.pone.0132100.
- Goyal K, Walton LJ, Tunnacliffe A. LEA proteins prevent protein aggregation due to water stress. Biochem J. 2005;388:151–157. doi:10.1042/BJ20041931.
- Redillas MCFR, Jeong JS, Kim YS, Jung H, Bang SW, Choi YD, Ha S-H, Reuzeau C, Kim J-K. The overexpression of OsNAC9 alters the root architecture of rice plants enhancing drought resistance and grain yield under field conditions. Plant Biotechnol J. 2012;10:792–805. doi:10.1111/j.1467-7652.2012.00697.x.
- Somerville C. Direct tests of the role of membrane lipid composition in low-temperature-induced photoinhibition and chilling sensitivity in plants and cyanobacteria. Proc Natl Acad Sci U S A. 1995;92:6215–6218. doi:10.1073/pnas.92.14.6215.
- Peng Y, Arora R, Li G, Wang X, Fessehaie A. Rhododendron catawbiense plasma membrane intrinsic proteins are aquaporins, and their over-expression compromises constitutive freezing tolerance and cold acclimation ability of transgenic Arabidopsis plants. Plant Cell Environ. 2008;31:1275–1289. doi:10.1111/j.1365-3040.2008.01840.x.
- Thomashow MF. Plant cold acclimation: freezing tolerance genes and regulatory mechanisms. Annu Rev Plant Physiol Plant Mol Biol. 1999;50:571–599. doi:10.1146/annurev.arplant.50.1.571.
- Agarwal PK, Agarwal P, Reddy MK, Sopory SK. Role of DREB transcription factors in abiotic and biotic stress tolerance in plants. Plant Cell Rep. 2006;25:1263–1274. doi:10.1007/s00299-006-0204-8.
- Uno Y, Furihata T, Abe H, Yoshida R, Shinozaki K, Yamaguchi-Shinozaki K. Arabidopsis basic leucine zipper transcription factors involved in an abscisic acid-dependent signal transduction pathway under drought and high-salinity conditions. Proc Natl Acad Sci. 2000;97:11632–11637. doi:10.1073/pnas.190309197.
- Choi H-I, Hong J-H, Ha J-O, Kang J-Y, Kim SY. ABFs, a family of ABA responsive element binding factors. J Biol Chem. 2000;275:1723–1730. doi:10.1074/jbc.275.3.1723.
- MoonHee L, Fukai S, Basnayake J Low temperature tolerance in rice: the Korean experience. In: Increased lowland rice production in the Mekong Region: Proceedings of an International Workshop; 2001. pp 109–117 30 October-2 November 2000; held in Vientiane, Laos.
- Zhang Q, Chen Q, Wang S, Hong Y, Wang Z. Rice and cold stress: methods for its evaluation and summary of cold tolerance-related quantitative trait loci. Rice (N Y). 2014;7(1):24. doi:10.1186/s12284-014-0024-3.
- Xu LM, Zhou L, Zeng YW, Wang F-M, Zhang H-L, Shen S-Q, Li Z-C. Identification and mapping of quantitative trait loci for cold tolerance at the booting stage in a japonica rice near-isogenic line. Plant Sci. 2008;174:340–347. doi:10.1016/j.plantsci.2007.12.003.
- Moraes de Freitas GP, Basu S, Ramegowda V, Eb B, Pereira A. Comparative analysis of gene expression in response to cold stress in diverse rice genotypes. Biochem Biophys Res Commun. 2016;471(1):253–259. Epub 2016 Feb 6. doi:10.1016/j.bbrc.2016.02.004.
- Ramegowda V, Basu S, Krishnan A, Pereira A. Rice growth under drought kinase is required for drought tolerance and grain yield under normal and drought stress conditions. Plant Physiol. 2014;166:1634–1645. doi:10.1104/pp.114.248203.
- Ambavaram MMR, Basu S, Krishnan A, Ramegowda, V., Batlang, U., Rahman, L., Baisakh, N., Pareira, A. Coordinated regulation of photosynthesis in rice increases yield and tolerance to environmental stress. Nat Commun. 2014;5:5302. doi:10.1038/ncomms6302.
- Livak KJ, Schmittgen TD. Analysis of relative gene expression data using real-time quantitative PCR and the 2−ΔΔCT Method. Methods. 2001;25:402–408. doi:10.1006/meth.2001.1262.
- Basu S, Roychoudhury A. Expression profiling of abiotic stress-inducible genes in response to multiple stresses in rice. Biomed Res Int. 2014;2014:12. doi:10.1155/2014/706890.
- White AJ, Dunn MA, Brown K, Hughes MA. Comparative analysis of genomic sequence and expression of a lipid transfer protein gene family in winter barley. 1994;45:1885–1892.
- Pintó-Marijuan M, Munné-Bosch S. Ecophysiology of invasive plants: osmotic adjustment and antioxidants. Trends Plant Sci. 2013;18:660–666. doi:10.1016/j.tplants.2013.08.006.
- Gu J, Yin X, Stomph T-J, Wang H, Struik PC. Physiological basis of genetic variation in leaf photosynthesis among rice (Oryza sativa L.) introgression lines under drought and well-watered conditions. J Exp Bot. 2012;63:5137–5153. doi:10.1093/jxb/err313.
- Aro EM, Virgin I, Andersson B. Photoinhibition of Photosystem II. Inactivation, protein damage and turnover. BBA - Bioenerg. 1993;1143:113–134. doi:10.1016/0005-2728(93)90134-2.
- Salminen TA, Blomqvist K, Edqvist J. Lipid transfer proteins: classification, nomenclature, structure, and function. Planta. 2016;244:971–997. doi:10.1007/s00425-016-2585-4.
- Sablowski RWM, Meyerowitz EM. A homolog of NO APICAL MERISTEM is an immediate target of the floral homeotic genes APETALA3/PISTILLATA. Cell. 1998;92:93–103. doi:10.1016/S0092-8674(00)80902-2.
- Kim Y-S, Kim S-G, Park J-E, Park H-Y, Lim M-H, Chua N-H, Park C-M. A membrane-bound NAC transcription factor regulates cell division in Arabidopsis. Plant Cell Online. 2006;18:3132–3144. doi:10.1105/tpc.106.043018.
- Balazadeh S, Kwasniewski M, Caldana C, Mehrnia M, Zanor MI, Xue G-P, Mueller-Roeber B. ORS1, an H2O2-responsive NAC transcription factor, controls senescence in Arabidopsis thaliana. Mol Plant. 2011;4:346–360. doi:10.1093/mp/ssq080.
- Kim JH, Woo HR, Kim J, Lim PO, Lee IC, Choi SH, Hwang D, Nam HG. Trifurcate feed-forward regulation of age-dependent cell death involving miR164 in Arabidopsis. Science. 2009;323(5917):1053–1057. doi:10.1126/science.1166386.
- Yamaguchi M, Ohtani M, Mitsuda N, Kubo M, Ohme-Takagi M, Fukuda H, Demura T. VND-INTERACTING2, a NAC domain transcription factor, negatively regulates xylem vessel formation in Arabidopsis. Plant Cell. 2010;22:1249–1263. doi:10.1105/tpc.108.064048.
- Zhong R, Richardson EA, Ye ZH. Two NAC domain transcription factors, SND1 and NST1, function redundantly in regulation of secondary wall synthesis in fibers of Arabidopsis. Planta. 2007;225:1603–1611. doi:10.1007/s00425-007-0498-y.
- Mitsuda N, Ohme-Takagi M. NAC transcription factors NST1 and NST3 regulate pod shattering in a partially redundant manner by promoting secondary wall formation after the establishment of tissue identity. Plant J. 2008;56:768–778. doi:10.1111/j.1365-313X.2008.03633.x.
- Yamaguchi M, Kubo M, Fukuda H, Demura T. Vascular-related NAC-DOMAIN7 is involved in the differentiation of all types of xylem vessels in Arabidopsis roots and shoots. Plant J. 2008;55:652–664. doi:10.1111/j.1365-313X.2008.03533.x.
- Zheng X, Chen B, Lu G, Han B. Overexpression of a NAC transcription factor enhances rice drought and salt tolerance. Biochem Biophys Res Commun. 2009;379:985–989. doi:10.1016/j.bbrc.2008.12.163.
- Nakashima K, Takasaki H, Mizoi J, Shinozaki K, Yamaguchi-Shinozaki K. NAC transcription factors in plant abiotic stress responses. Biochim Biophys Acta Gene Regul Mech. 2012;1819:97–103. doi:10.1016/j.bbagrm.2011.10.005.
- Wang X, Basnayake BMVS, Zhang H, Li G, Li W, Virk N, Mengiste T, Song F. The Arabidopsis ATAF1, a NAC transcription factor, is a negative regulator of defense responses against necrotrophic fungal and bacterial pathogens. Mol Plant Microbe Interact. 2009;22:1227–1238. doi:10.1094/MPMI-22-10-1227.
- Jeong JS, Kim YS, Baek KH, Jung H, Ha SH, Do Choi Y, Kim M, Reuzeau C, Kim JK. Root-specific expression of OsNAC10 improves drought tolerance and grain yield in rice under field drought conditions. Plant Physiol. 2010;153:185–197. doi:10.1104/pp.110.154773.
- Schluttenhofer C, Yuan L. Regulation of specialized metabolism by WRKY transcription factors. Plant Physiol. 2015;167:295–306. doi:10.1104/pp.114.251769.
- Dey A, Samanta MK, Gayen S, Sen SK, Maiti MK, Subudhi PK. Enhanced gene expression rather than natural polymorphism in coding sequence of the OsbZIP23 determines drought tolerance and. PLoS One. 2016;11:1–26. doi:10.1371/journal.pone.0150763.
- Kanneganti V, Gupta AK. Overexpression of OsiSAP8, a member of stress associated protein (SAP) gene family of rice confers tolerance to salt, drought and cold stress in transgenic tobacco and rice. Plant Mol Biol. 2008;66:445–462. doi:10.1007/s11103-007-9284-2.
- Tian C, Wan P, Sun S, Li J, Chen M. Genome-wide analysis of the GRAS gene family in rice and Arabidopsis. Plant Mol Biol. 2004;54:519–532. doi:10.1023/B:PLAN.0000038256.89809.57.
- Zhu G, Ye N, Zhang J. Glucose-induced delay of seed germination in rice is mediated by the suppression of ABA catabolism rather than an enhancement of ABA biosynthesis. Plant Cell Physiol. 2009;50:644–651. doi:10.1093/pcp/pcp022.
- Ding X, Hou X, Xie K, Xiong L. Genome-wide identification of BURP domain-containing genes in rice reveals a gene family with diverse structures and responses to abiotic stresses. Planta. 2009;230:149–163. doi:10.1007/s00425-009-0929-z.
- Liu H, Ma Y, Chen NA, Guo S, Liu H, Guo X, Chong K, Xu Y. Overexpression of stress‐inducible OsBURP16, the β subunit of polygalacturonase 1, decreases pectin content and cell adhesion and increases abiotic stress sensitivity in rice. Plant Cell Environ. 2014 May 1;37(5):1144–1158. doi:10.1111/pce.12223.