ABSTRACT
Thiol-disulfide redox exchanges are widely distributed modifications of great importance for metabolic regulation in living cells. In general, the formation of disulfide bonds is controlled by thioredoxins (TRXs), ubiquitous proteins with two redox-active cysteine residues separated by a pair of amino acids. While the function of plastidial TRXs has been extensively studied, the role of the mitochondrial TRX system is much less well understood. Recent studies have demonstrated that the mitochondrial TRXs are required for the proper functioning of the major metabolic pathways, including stomatal function and antioxidant metabolism under sub-optimal conditions including drought and salinity. Furthermore, inactivation of mitochondrial TRX system leads to metabolite adjustments of both primary and secondary metabolism following drought episodes in arabidopsis, and makes the plants more resistant to salt stress. Here we discuss the implications of these findings, which clearly open up several research avenues to achieve a full understanding of the redox control of metabolism under environmental constraining conditions.
Involvement of mitochondrial TRX in abiotic stress responses
Redox reactions substantially influence the activity of several proteins and participate in the regulation of crucial cellular processes.Citation1 Accordingly, a growing body of evidence has highlighted the importance of the thioredoxins (TRXs) for the redox control of plant metabolism.Citation2,Citation3 TRXs are small proteins containing a redox active disulfide group within its catalytic domain. Being widely distributed throughout most living cells,Citation4 TRXs are involved in a variety of cellular redox reactions. Numerous isoforms of TRXs are present in plants, differing in both amino acid sequence and subcellular localization. Over 20 isoforms have been identified in the genome of arabidopsis and grouped into seven subfamilies (f-, m-, h-, o-, x- y-, and z-types).Citation5,Citation6 The vast majority of TRXs isoforms are located in the chloroplast, but TRXs are also present in mitochondria (TRXs o and h) and cytosol (TRX h), where the presence of highly similar isoforms of NADPH-dependent TRX reductase (NTR), A and B, complete a functional TRX system.Citation7,Citation8
Non-plastid TRX system also includes proteins located at the nucleus (TRXs h-type in wheat seeds,Citation9,Citation10 NTRA in arabidopsisCitation11 and peaCitation12 and TRX o1 in pea leavesCitation13), endoplasmic reticulumCitation14 (TRXs h2, h7, and h8) and attached to the plasma membraneCitation15 (TRX h9). The redox regulation of chloroplast function has received considerable attention over the last decades; however, the functional role of redox processes in other cell compartments remains unclear. For instance, the functional role of the extraplastidial NTR/TRX system remains poorly understood, most likely due to the extensive functional redundancies between TRXs and glutaredoxins (GRXs) and also between NTRs found in both mitochondria and cytosol.Citation5,Citation16 The introduction of proteomics-based approaches to TRX studies have greatly contributed to the identification of putative TRX targets in plants, especially in the mitochondria.Citation7,Citation17–Citation20 Since then, hundreds of putative targets involved in a broad spectrum of mitochondrial processes have been identified, including photorespiration, tricarboxylic acid (TCA) cycle and associated reactions, ATP synthesis, hormone synthesis, and stress-related reactions.Citation17,Citation18 However, it is important to emphasize that such proteomic approaches provide substantial false-positive interactions and also lack the information whether the TRX-target interaction identified in vitro also occurs in vivo. Thus, further experiments with mutants and/or recombinant proteins are needed to fully elucidate the functionality of the TRX-target proteins interaction. In this vein, experimental validation successfully demonstrated that alternative oxidase (AOX)Citation21 and some of the enzymes of the TCA cycle including isocitrate dehydrogenase,Citation22 citrate synthase,Citation23,Citation24 succinate dehydrogenase, and fumarase are regulated by the mitochondrial TRX system in vitro and/or in vivo.Citation25 Furthermore, cytosolic malate dehydrogenase has also been demonstrated to be regulated by TRXs.Citation26
The mechanisms that coordinate mitochondrial TRX regulation and its implications for the dynamic of plant metabolism under different stresses conditions are poorly understood. However, the significance of mitochondrial redox metabolism in cellular signaling processes coupled with studies of different loss-of-function mutants have recently enabled us to grasp the importance of mitochondrial TRXs under stress conditions.Citation25,Citation27–Citation29 In order to gain further insights into the physiological and metabolic function of the mitochondrial TRX/NTR system, we have recently investigated the significance of the mitochondrial TRX system under consecutive drought episodes. Using the arabidopsis ntra ntrb double mutant and two independent mitochondrial trxo1 mutants, our study demonstrated that the lack of a functional mitochondrial NTR/TRX system enhances drought tolerance following single and multiple events of drought.Citation27Extensive metabolic and physiological analyses of these mutants revealed multiple and complex responses following both single and repetitive drought episodes.Citation27 Notably, TRX o1 transcripts were more highly expressed under drought, an effect that was even stronger during repetitive drought/recovery events.Citation27 Compelling evidence from several plant species indicates that AOX transcript and protein increase during drought (reviewed inCitation30). Given that TRX o1 may function in the reductive activation of AOX,Citation12 further investigation to elucidate whether the proposed protective role for TRX o1 under droughtCitation27 and salinityCitation28,Citation29 is possibly also associated to the redox modulation of AOX. Notwithstanding, we further showed that the levels of a large number of secondary metabolites (glucosinolates, anthocyanins, flavonol glycosides, and hydroxycinnamates) increased in at least one of the TRX mutants following two cycles of drought.Citation27 This result reinforces the idea that secondary metabolism is redox-regulated by TRX system.Citation25,Citation31 What remains unclear and thus deserve further investigation is which enzymes of the secondary metabolism are the target of TRXs. Additionally, our study demonstrated that TRX-mediated redox regulation seems to be crucial for stomatal function following the plant recovery from a second drought event.Citation27
Similar to the situation observed under water limitation, compensatory readjustments were also observed in the responses of plants lacking the mitochondrial TRX o1 following salt stress. These responses included alterations in the glutathione redox state and/or up-regulation of AOXCitation32 and antioxidant enzymesCitation28,Citation29 (). Moreover, Attrxo1 seeds germinated faster and accumulated higher H2O2 content under salinity, suggesting that TRX o1 could act as a sensor under salinity and/or an inducer of H2O2 accumulation.Citation28 The expression of the antioxidant enzymes AtPRXIIF and AtSRX was not altered during germination in either water or NaCl.Citation28 Conversely, in pea plants the expression of TRX o1 and PRXIIF increased in response to short-term salt stressCitation32,Citation33 highlighting to the heterogeneity of the antioxidant system depending on the stress condition, which may in turn be related to specific TRX o1 targets following seed germination, a point that clearly deserves further investigation.Citation28 Collectively, these observations are in good agreement with our proposal that perturbation of mitochondrial TRX affects cellular redox metabolism in general. It seems likely that in the absence of a functional mitochondrial TRX system the main mechanisms used to maintain redox homeostasis under drought/salt include an increased concentration of secondary metabolites and higher activities of enzymes of redox metabolism such as superoxide dismutase and catalase (). Moreover, stomatal behavior – allowing higher stomatal closure during salt stressCitation29 and better recovery of stomatal conductance following rehydrationCitation27 () – was shown to be a key factor for the maintenance of plant growth.
Figure 1. Schematic model showing the main responses of TRX o1 mutant plants to salt and/or drought/drought recovery. Two independent studies have recently demonstrated that several physiological processes related to metabolism, stomatal function and antioxidant metabolism are affected in Arabidopsis thaliana plants with knockout of the mitochondrial TRX o1.Citation27,Citation29 The inactivation of TRX o1 leads to increases and decreases in both primary and secondary metabolism during drought and recovery, respectivelyCitation27 (further details are found in the main text). Moreover, stomatal function is affected in the mutants under salinity and drought recovery: lower water loss and higher stomatal closure under salinity but higher stomatal conductance (gs) following drought recovery were observed. Although higher levels of H2O2 and lipid peroxidation were found in these plants, it seems that increased activities of antioxidative enzymes such as Mn-SOD, Fe-SOD, Cu/Zn-SOD, GR and catalase are likely able to counteract TRX o1 deficiency in arabidopsis mutant plants under salinity.
Abbreviations: Mn-SOD, manganese superoxide dismutase; Fe-SOD, iron superoxide dismutase; Cu/Zn-SOD, copper-zinc, GR, glutathione reductase
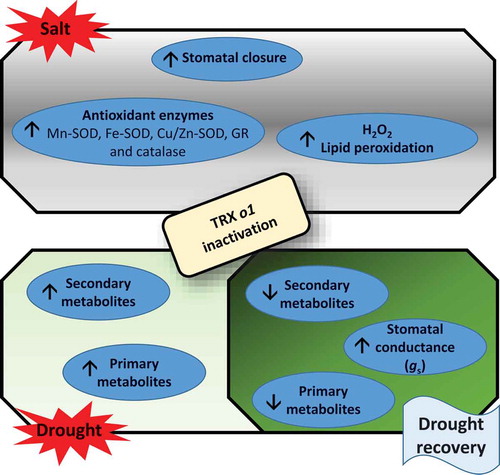
Figure 2. Relationship between the ratio of electron transport rate (ETR) and net photosynthesis (AN) versus stomatal conductance (gs) in TRX arabidopsis knockout mutants. The genotypes used here were: ntra ntrb, trxo1-1 and trxo1-2, and wild-type plants (WT) during dehydration and following rehydration. Data was calculated from da Fonseca-Pereira et al. 2019.Citation27 Regression coeficiente and P value are shown.**Means the significant difference of the relationship between AN/ETR x gs. in a two-tailed Fisher’s exact test (P< 0.001).
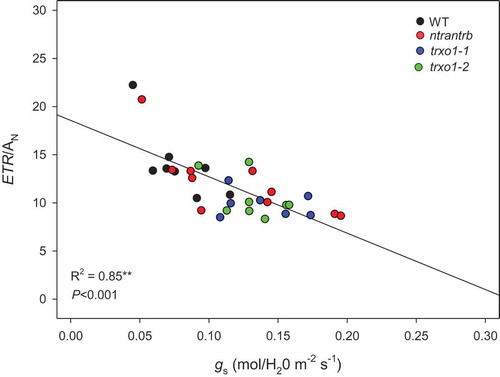
To further elucidate the physiological performance of the TRX mutant lines after drought, here we compared the relationship between the ratio of electron transport rate (ETR) and net photosynthesis (AN) versus stomatal conductance (gs) (extracted fromCitation27). Both parameters can be employed as physiological status indicators.Citation34,Citation35 ETR/AN ratio reflects the energy transfer to the photosynthesis. Under optimum conditions, AN is the main sink, however, under stress AN is more sensitive and reduces faster than ETR, promoting the ratio increase and indicating an energy impairment that can be associated to ROS production.Citation34 Accordingly, following drought recovery after drought (irrigation for 3 days) an inverse relationship between both parameters was observed, where WT plants displayed higher ETR/AN (indicating higher physiological stress) accompanied by lower gs; meanwhile TRX mutant lines showed comparatively lower ratios with higher gs for the same recovery period ().
Perspectives in understanding mitochondrial redox regulation
While the concept that perturbations in mitochondrial homeostasis exert a major influence on different cellular processes,Citation36 many uncertainties remain concerning the interacting pathways that underpin each process. Moreover, many of the mechanisms and components involved in the redox control of plant responses to stress situations, in general, remain to be fully identified.
Given the multiplicity of targets that TRXs are able to reduce and the yet unknown processes in which they are likely involved,Citation37 it will be interesting, in the coming years, to focus on determining the specific targets of the mitochondrial TRX enzymes in vivo. Furthermore, the characterization of mutants lacking multiple TRXs or compensatory TRX proteins such as GRXs, peroxiredoxins, and sulfiredoxins will be important to unravel the redundant and the complementarity of the different components of the redox metabolism, especially under stress conditions. The combination of proteomics with metabolomics seems to be an excellent strategy to improve our understanding regarding redox regulation of the metabolism. Such approaches will likely be of paramount importance in providing useful information for improved metabolic engineering to develop stress-tolerant plants.
Disclosure of potential conflicts of interest
No potential conflicts of interest were disclosed.
Acknowledgments
We acknowledge the helpful comments made by the anonymous referees on previous versions of our manuscript.
Additional information
Funding
References
- Kocsy G, Tari I, Vanková R, Zechmann B, Gulyás Z, Poór P, Galiba G. Redox control of plant growth and development. Plant Sci. 2013;211:77–91. PMID: 23987814. doi:10.1016/j.plantsci.2013.07.004.
- Geigenberger P, Thormählen I, Daloso DM, Fernie AR. The unprecedented versatility of the plant thioredoxin system. Trends Plant Sci. 2017;22:249–262. PMID: 28139457.doi:10.1016/j.tplants.2016.12.008.
- Buchanan BB. The path to thioredoxin and redox regulation in chloroplasts. Annu Rev Plant Biol. 2016;67:1–24. PMID: 27128465. doi:10.1146/annurev-arplant-043015-111949.
- Yano H. Ongoing applicative studies of plant thioredoxins. Mol Plant. 2014;7:4–13. PMID: 23966635. doi:10.1093/mp/sst124.
- Belin C, Bashandy T, Cela J, Delorme-Hinoux V, Riondet C, Reichheld JP. A comprehensive study of thiol reduction gene expression under stress conditions in Arabidopsis thaliana. Plant Cell Environ. 2015;38:299–314. PMID: 24428628. doi:10.1111/pce.12276.
- Thormählen I, Meitzel T, Groysman J, Öchsner AB, von Roepenack-Lahaye E, Naranjo B, Cejudo FJ, Geigenberger P. Thioredoxin f1 and NADPH-dependent thioredoxin reductase C have overlapping functions in regulating photosynthetic metabolism and plant growth in response to varying light conditions. Plant Physiol. 2015;169:1766–1786. PMID: 26338951. doi:10.1104/pp.15.01122.
- Montrichard F, Alkhalfioui F, Yano H, Vensel WH, Hurkman WJ, Buchanan BB. Thioredoxin targets in plants: the first 30 years. J Proteomics. 2009;72:452–474. PMID: 19135183.doi:10.1016/j.jprot.2008.12.002.
- Reichheld JP, Meyer E, Khafif M, Bonnard G, Meyer Y. AtNTRB is the major mitochondrial thioredoxin reductase in Arabidopsis thaliana. FEBS Lett. 2005;579:337–342. doi:10.1016/j.febslet.2004.11.094.
- Serrato AJ, Cejudo FJ. Type-h thioredoxins accumulate in the nucleus of developing wheat seed tissues suffering oxidative stress. Planta. 2003;217:392–399. PMID: 14520565. doi:10.1007/s00425-003-1009-4.
- Pulido P, Cazalis R, Cejudo FJ, Ame A. An antioxidant redox system in the nucleus of wheat seed cells suffering oxidative stress. Plant J. 2009;132–145. PMID: 18786001. doi:10.1111/j.1365-313X.2008.03675.x.
- Marchal C, Delorme-Hinoux V, Bariat L, Siala W, Belin C, Saez-Vasquez J, Riondet C, Reichheld JP. NTR/NRX define a new thioredoxin system in the nucleus of Arabidopsis thaliana cells. Mol Plant. 2014;7:30–44. PMID: 24253198. doi:10.1093/mp/sst162.
- Martí MC, Olmos E, Calvete JJ, Díaz I, Barranco-Medina S, Whelan J, Lázaro JJ, Sevilla F, Jiménez A. Mitochondrial and nuclear localization of a novel pea thioredoxin: identification of its mitochondrial target proteins. Plant Physiol. 2009;150:646–657. PMID: 19363090. doi:10.1104/pp.109.138073.
- Calderón A, Ortiz-Espín A, Iglesias-Fernández R, Carbonero P. Redox Biology Thioredoxin (Trx o 1) interacts with proliferating cell nuclear antigen (PCNA) and its overexpression a ff ects the growth of tobacco cell culture. Redox Biol. 2017;11:688–700. PMID: 28183062. doi:10.1016/j.redox.2017.01.018.
- Traverso JA, Micalella C, Martinez A, Brown SC, Satiat-Jeunemaître B, Meinnel T, Giglione C. Roles of N-terminal fatty acid acylations in membrane compartment partitioning: Arabidopsis h-Type thioredoxins as a case study. Plant Cell. 2013;25:1056–1077. PMID: 23543785. doi:10.1105/tpc.112.106849.
- Meng L, Wong JH, Feldman LJ, Lemaux PG, Buchanan BB. A membrane-associated thioredoxin required for plant growth moves from cell to cell, suggestive of a role in intercellular communication. Proc Natl Acad Sci USA. 2010;107:3900–3905. PMID: 20133584. doi:10.1073/pnas.0913759107.
- Reichheld JP, Riondet C, Delorme V, Vignols F, Meyer Y. Thioredoxins and glutaredoxins in development. Plant Sci. 2010;178:420–423. doi:10.1016/j.plantsci.2010.03.001.
- Balmer Y, Vensel WH, Tanaka CK, Hurkman WJ, Gelhaye E, Rouhier N, Jacquot J-P, Manieri W, Schurmann P, Droux M, et al. Thioredoxin links redox to the regulation of fundamental processes of plant mitochondria. Proc Natl Acad Sci USA. 2004;101:2642–2647. PMID: 14983062. doi:10.1073/pnas.0308583101.
- Yoshida K, Noguchi K, Motohashi K, Hisabori T. Systematic exploration of thioredoxin target proteins in plant mitochondria. Plant Cell Physiol. 2013;54:875–892. PMID: 23444301. doi:10.1093/pcp/pct037.
- Mock HP, Dietz KJ. Redox proteomics for the assessment of redox-related posttranslational regulation in plants. Biochim Biophys Acta. 2016;1864:967–973. PMID: 26784836. doi:10.1016/j.bbapap.2016.01.005.
- Nietzel T, Mostertz J, Hochgräfe F, Schwarzländer M. Redox regulation of mitochondrial proteins and proteomes by cysteine thiol switches. Mitochondrion. 2017;33:72–83. PMID: 27456428. doi:10.1016/j.mito.2016.07.010.
- Gelhaye E, Rouhier N, Gérard J, Jolivet Y, Gualberto J, Navrot N, Ohlsson P-I, Wingsle G, Hirasawa M, Knaff DB, et al. A specific form of thioredoxin h occurs in plant mitochondria and regulates the alternative oxidase. Proc Natl Acad Sci USA. 2004;101:14545–14550. PMID: 15385674. doi:10.1073/pnas.0405282101.
- Yoshida K, Hisabori T. Mitochondrial isocitrate dehydrogenase is inactivated upon oxidation and reactivated by thioredoxin-dependent reduction in Arabidopsis. Front Environ Sci. 2014;2:1–7. doi:10.3389/fenvs.2014.00038.
- Stevens FJ, Li AD, Lateef SS, Anderson LE. Identification of potential inter-domain disulfides in three higher plant mitochondrial citrate synthases : paradoxical differences in redox-sensitivity as compared with the animal enzyme. Photosyn Res. 1997:185–197. doi:10.1023/A:1005991423503.
- Schmidtmann E, König A-C, Orwat A, Leister D, Hartl M, Finkemeier I. Redox regulation of Arabidopsis mitochondrial citrate synthase. Mol Plant. 2014;7:156–169. PMID: 24198232. doi:10.1093/mp/sst144.
- Daloso DM, Müller K, Obata T, Florian A, Tohge T, Bottcher A, Riondet C, Bariat L, Carrari F, Nunes-Nesi A, et al. Thioredoxin, a master regulator of the tricarboxylic acid cycle in plant mitochondria. Proc Natl Acad Sci USA. 2015;112:E1392–400. PMID: 25646482. doi:10.1073/pnas.1424840112.
- Hara S, Motohashi K, Arisaka F, Romano PGN, Hosoya-Matsuda N, Kikuchi N, Fusada N, Hisabori T. Thioredoxin-h1 reduces and reactivates the oxidized cytosolic malate dehydrogenase dimer in higher plants. J Biol Chem. 2006;281:32065–32071. PMID: 16945919. doi:10.1074/jbc.M605784200.
- da Fonseca-Pereira P, Daloso DM, Gago J, Silva FMDO, Condori-Apfata JA, Florez-Sarasa I, Tohge T, Reichheld J-P, Nunes-Nesi A, Fernie AR, Araújo WL. The mitochondrial thioredoxin system contributes to the metabolic responses under drought episodes in arabidopsis. Plant Cell Physiol. 2019;60:213–229. PMID: 30329109. 10. doi:10.1093/pcp/pcy194.
- Ortiz-Espín A, Iglesias-Fernández R, Calderón A, Carbonero P, Sevilla F, Jiménez A. Mitochondrial AtTrxo1 is transcriptionally regulated by AtbZIP9 and AtAZF2 and affects seed germination under saline conditions. J Exp Bot PMID: 28184497. 2017;68:1025–1038. doi:10.1093/jxb/erx012.
- Calderón A, Sánchez-Guerrero A, Ortiz-Espín A, Martínez-Alcalá I, Camejo D, Jiménez A, Sevilla F. Lack of mitochondrial thioredoxin o1 is compensated by antioxidant components under salinity in Arabidopsis thaliana plants. Physiol Plant. 2018;164:251–267. PMID: 29446456. doi:10.1111/ppl.12708.
- Vanlerberghe GC, Martyn GD, Dahal K. Alternative oxidase : a respiratory electron transport chain pathway essential for maintaining photosynthetic performance during drought stress. Physiol Plant. 2016;157:322–337. PMID: 27080742. doi:10.1111/ppl.12451.
- Bashandy T, Taconnat L, Renou JP, Meyer Y, Reichheld JP. Accumulation of flavonoids in an ntra ntrb mutant leads to tolerance to UV-C. Mol Plant. 2009;2:249–258. PMID: 19825611. doi:10.1093/mp/ssn065.
- Martí MC, Florez-Sarasa I, Camejo D, Ribas-Carbó M, Lázaro JJ, Sevilla F, Jiménez A. Response of mitochondrial thioredoxin PsTrxo1, antioxidant enzymes, and respiration to salinity in pea (Pisum sativum L.) leaves. J Exp Bot. 2011;62:3863–3874. PMID: 21460385. doi:10.1093/jxb/err076.
- Barranco-Medina S, Krell T, Bernier-Villamor L, Sevilla F, Lázaro JJ, Dietz KJ. Hexameric oligomerization of mitochondrial peroxiredoxin PrxIIF and formation of an ultrahigh affinity complex with its electron donor thioredoxin Trx-o. J Exp Bot. 2008;59:3259–3269. PMID: 18632730. doi:10.1093/jxb/ern177.
- Flexas J, Bota J, Escalona JM, Sampol BMH. Effects of drought on photosynthesis in grapevines under field conditions: an evaluation of stomatal and mesophyll limitations. Funct Plant Biol. 2002;29:461–471.
- Flexas J, Medrano H. Drought-inhibition of photosynthesis in C3 Plants : stomatal and non-stomatal limitations Revisited. Ann Bot. 2002;89:183–189. PMID: 12099349. doi:10.1093/aob/mcf027.
- Zhang Y, Fernie AR. On the role of the tricarboxylic acid cycle in plant productivity. J Integr Plant Biol. 2018;60(12):1199–1216. PMID: 29917310. doi:10.1111/jipb.12690.
- Marchand C, Le Maréchal P, Meyer Y, Miginiac-Maslow M, Issakidis-Bourguet E, Decottignies P. New targets of Arabidopsis thioredoxins revealed by proteomic analysis. Proteomics. 2004;4:2696–2706. PMID: 15352244. doi:10.1002/pmic.200400805.