ABSTRACT
Local action of stressors induces generation and propagation of electrical signals (ESs), which influence numerous physiological processes (including photosynthesis, expression of genes, production of phytohormones, etc.) in undamaged parts of plants; i.e. they induce a systemic stress response. Development of methods of remote sensing of this response (in particular, optical methods) is an important practical task for agricultural and ecological monitoring. However, this problem is not sufficiently researched. Earlier, we reported that ESs influence the photochemical reflectance index, which can be calculated on the basis of reflected light at 531 and 570 nm, and these changes are connected with photosynthetic changes. The aim of the current work is investigation of the influence of ESs on reflectance at broad spectral bands (400–500 nm, 500–600 nm, 600–700 nm and 700–800 nm). We showed that burning-induced ESs caused transient increase of intensity of reflected light at the all investigated spectral bands of visible light: reflectance at 600–700 nm had the maximal magnitude of changes and reflectance at 700–800 nm had the minimal magnitude of changes. Dynamics of the reflectance changes were distinguished from dynamics of photosynthetic changes, induced by ESs; i.e. ESs-induced changes in reflectance seem to be weakly connected with the photosynthetic response. Thus, our results show that changes in reflectance at broad spectral bands can also be used for remote sensing of the ESs-induced systemic stress response in plants.
Action of many stressors (excessive light, high and low temperatures, drought, mechanical wounding, insect attack, etc.) on terrestrial plants can be spatially heterogeneous; a systemic physiological response on their action requires generation and propagation of long-distance stress signals. Electrical signals (ESs) play important roles in the induction of the systemic response,Citation1–Citation3 which includes changes in photosynthesis, expression of genes, production of phytohormones, respiration, transpiration, phloem mass-flow, etc. Potentially, the ESs-induced systemic physiological response can be used in the agricultural and ecological monitoring for early revealing of stressor action on plants.Citation3 Numerous spectral indices, which are based on measurements of reflected light at different spectral bands, are effective tools for the remote sensing of the plant physiological state.Citation4–Citation12 However, potential influence of ESs on parameters of the leaf reflectance is not sufficiently researched now.
Previously, we showedCitation13 that ESs decreased a photochemical reflectance index, which is calculated on the basis of intensities of the reflected light at 531 and 570 nm. The effect was strongly connected with changes in photosynthetic processes, including decrease of a quantum yield of photosystem II (ФPSII) and increase of a non-photochemical quenching of chlorophyll fluorescence (NPQ). The aim of the current work was investigation of the influence of ESs on reflectance at broad spectral bands (400–500 nm, 500–600 nm, 600–700 nm and 700–800 nm). Revealing of ESs-induced changes in the reflectance at these spectral bands can be the basis of future investigation of the possibility of the remote sensing of the ESs-induced systemic response with using of the RGB-imaging.
Pea seedlings (Pisum sativum L., 14–21 days old) were investigated in our work. The experimental procedure was described in our previous workCitation13 in detail. Briefly, the first mature leaf was burned (flame, 3–4 s, approximately 1 cm2). Surface electrical potential, photosynthetic parameters and reflectance were investigated in the second and fourth leaves. Electrical potential was measured by extracellular Ag+/AgCl electrodes (EVL-1M3.1, RUE “Gomel Measuring Equipment Plant,” Gomel, Belarus) and a high-impedance amplifier IPL-113 (Semico, Novosibirsk, Russia). Electrodes were placed on the leaflet of the leaf and stem near this leaf; the reference electrode was placed in the root solution. Photosynthetic parameters (ФPSII and NPQ) were measured by a Dual-PAM-100 (Heinz Walz GmbH, Effeltrich, Germany); saturation pulses of the red light (630 nm, 10 000 µmol m−2s−1, 300 ms) and weak pulses of the blue measuring light (460 nm, 24 µmol m−2s−1, 2.5 μs) were used. Spectrum of the reflected light was measured by a wide-range spectrometer S100 (SOLAR Laser Systems, Minsk, Belarus); a white card (QPcard 101 Calibration Card v3, Argraph Corp., Carlstadt, USA) was used for calibration. A halogen lamp (Osram Decostar, 3000К, 20 W, 12 V, Germany) was used as a source of the white actinic light (630 µmol m−2s−1); its spectrum is shown in ). ) shows a spectrum of the reflected light in pea leaves which was very similar to a reflectance spectrum in leaves of other plants.Citation5,Citation14 Relative intensities of the reflected light at 400–500 nm, 500–600 nm, 600–700 nm and 700–800 nm were calculated as sums of reflected light intensities at these ranges; the sums before induction of ESs were assumed as 100%.
Figure 1. (a). The light spectrum of the white actinic light, which was used in the current work. (b). The reflectance spectrum of the pea leaf. (c). Local burning-induced electrical signals in leaflets of the second and fourth leaves and in the stem near these leaves (n= 5). (d). Averaged dynamics of the quantum yield of photosystem II (ФPSII) and the non-photochemical quenching in chlorophyll fluorescence (NPQ) in the second and fourth leaves after local burning (n= 5). The first leaf was burned (flame, 3–4 s, approximately 1 cm2), it is marked by the arrow. The white card was used for calibration and calculation of the leaf reflectance.
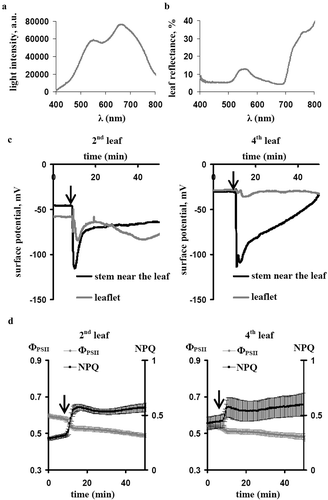
Local burning of the first leaf induced propagation of ESs (variation potentials) in the second leaf; however, the signals were weak in the fourth leaf ()) that is in a good accordance with our early works.Citation13,Citation15,Citation16 ) shows averaged tracks of ФPSII and NPQ after burning: the significant changes in photosynthetic parameters were observed in the second leaf and were absent in the fourth leaf, that was in a good accordance with the propagation of ESs.
shows that the local burning of the first leaf and propagation of ESs transiently increased relative intensities of reflected light at 400–500 nm, 500–600 nm, 600–700 nm and 700–800 nm in the second leaf. The effect was maximal for reflectance at 600–700 nm and minimal for reflectance at 700–800 nm. Maximal values of increase were in 25–30 min after stimulation; small decrease of reflectance was observed after that. It is important that dynamics of changes in the reflected light intensity was not similar with dynamics of changes in photosynthetic parameters; in particular, correlation coefficients between these changes were not significant (). Significant changes in reflectance in the fourth leaf were absent that was in a good accordance with weak ESs in this leaf ()).
Figure 2. Averaged dynamics of relative intensities of the reflected light (RL) at 400–500 nm (a), 500–600 nm (b), 600–700 nm (c) and 700–800 nm (d) in the second and fourth leaves after local burning (n= 5). The first leaf was burned (flame, 3–4 s, approximately 1 cm2), it is marked by the arrow. The intensity of the reflected light before burning was assumed as 100%. Averaged values are shown as black bold lines; the grey shadows mark standard errors of these values.
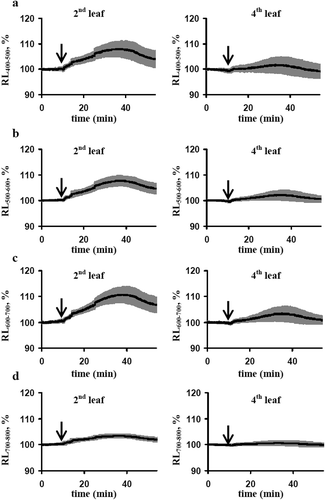
Figure 3. Dependences of changes in relative intensities of the reflected light (∆RL) at 400–500 nm (a), 500–600 nm (b), 600–700 nm (c) and 700–800 nm (d) on changes in the quantum yield of photosystem II (∆ФPSII) and the non-photochemical quenching in chlorophyll fluorescence (∆NPQ) in the second leaf of pea (n= 20). Changes were calculated as differences between values of reflected light intensities and photosynthetic parameters at 10, 20, 30 and 40 min after burning and these values before induction of variation potential; the changes were calculated for each experimental pea seedling. R is the correlation coefficient.
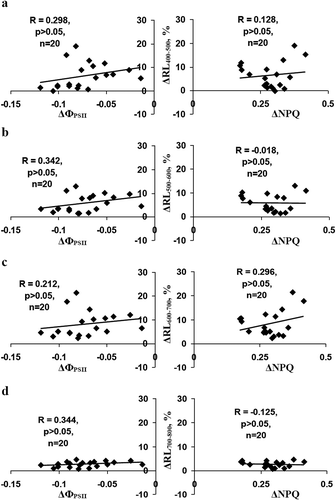
Thus, our results show that local burning can influence reflectance in pea leaves at broad spectral bands. This effect is connected with ESs because it was observed at the ESs propagation (the second leaf) and was absent at absence of the ESs propagation (the fourth leaf). However, this response seems to be weakly connected with photosynthetic response because the time courses of photosynthetic changes ()) and reflectance increases () were different. This difference was supported by low correlation coefficients between changes in parameters of reflectance and photosynthetic parameters (). Mechanisms of the changes require future investigations; it is probable that they can be connected with changes in the total reflectance of the leaf. It can be speculated that the effect may be connected with changes in a leaf thickness because (i) the generation of ESs can be associated with increase of the thickness of plant organs (the increase is connected with propagation of hydraulic wave through plant body);Citation17 (ii) ESs induce a long-term decrease of transpirationCitation18,Citation19 (the decrease is connected with inactivation of H+-ATPase in the plasma membrane) that may also increase the leaf thickness; and (iii) increase of this thickness is positively correlated with the increase of reflectance.Citation20 It is interesting that minimal changes in the intensity of the reflected light were observed at 700–800 nm; i.e. at a low absorption of light.Citation20 The result shows that value of the light absorption can, potentially, influence magnitude of ESs-induced changes in reflectance; however, revealing the mechanisms of the influence requires future investigation, which will be, possibly, based on analysis of the optical model of a plant leaf. Additionally, our results show that the ESs-induced systemic physiological response, potentially, can be detected by the standard digital camera because changes in reflectance were observed at the broad spectral range; however, this possibility also requires future investigations.
Disclosure of Potential Conflicts of Interest
No potential conflicts of interest were disclosed.
Additional information
Funding
References
- Fromm J, Lautner S. Electrical signals and their physiological significance in plants. Plant Cell Environ. 2007;30:249–257. doi:10.1111/j.1365-3040.2006.01614.x.
- Sukhov V. Electrical signals as mechanism of photosynthesis regulation in plants. Photosynth Res. 2016;130:373–387. doi:10.1007/s11120-016-0270-x.
- Sukhov V, Sukhova E, Vodeneev V. Long-distance electrical signals as a link between the local action of stressors and the systemic physiological responses in higher plants. Progr Biophys Mol Biol. 2019. online early doi:10.1016/j.pbiomolbio.2018.11.009
- Rouse JW, Haas RH Jr, Schell JA, Deering DW, Harlan JC Monitoring the vernal advancement and retrogradation (green wave eff ect) of natural vegetation. Type III fi nal rep. NASA/GSFC, Greenbelt (MD). 1974.
- Rondeaux G, Steven M, Baret F. Optimization of soil-adjusted vegetation indices. Remote Sens Environ. 1996;55:95–107. doi:10.1016/0034-4257(95)00186-7.
- Peñuelas J, Pinol J, Ogaya R, Filella I. Estimation of plant water concentration by the reflectance Water Index WI (R900/R970). Int J Remote Sens. 1997;18:2869–2875. doi:10.1080/014311697217396.
- Eitel JUH, Long DS, Gessler PE, Hunt ER. Combined spectral index to improve ground-based estimates of nitrogen status in dryland wheat. Agron J. 2008;100:1694–1702. doi:10.2134/agronj2007.0362.
- Garbulsky MF, Peñuelas J, Gamon J, Inoue Y, Filella I. The photochemical reflectance index (PRI) and the remote sensing of leaf, canopy and ecosystem radiation use efficiencies. A review and meta-analysis. Remote Sens Environ. 2011;115:281–297. doi:10.1016/j.rse.2010.08.023.
- Gamon JA, Huemmrich KF, Wong CY, Ensminger I, Garrity S, Hollinger DY, Noormets A, Peñuelas J. A remotely sensed pigment index reveals photosynthetic phenology in evergreen conifers. Proc Natl Acad Scis USA. 2016;113:13087–13092. doi:10.1073/pnas.1606162113.
- Zhang C, Filella I, Garbulsky MF, Peñuelas J. Affecting factors and recent improvements of the photochemical reflectance index (PRI) for remotely sensing foliar, canopy and ecosystemic radiation-use efficiencies. Remote Sens. 2016;8:677. doi:10.3390/rs8090677.
- Sukhova E, Sukhov V. Connection of the photochemical reflectance index (PRI) with the photosystem II quantum yield and nonphotochemical quenching can be dependent on variations of photosynthetic parameters among investigated plants: A meta-analysis. Remote Sens. 2018;10:771. doi:10.3390/rs10050771.
- Sukhova E, Sukhov V. Analysis of light-induced changes in the photochemical reflectance index (PRI) in leaves of pea, wheat, and pumpkin using pulses of green-yellow measuring light. Remote Sens. 2019;11:810. doi:10.3390/rs11070810.
- Sukhov V, Sukhova E, Gromova E, Surova L, Nerush V, Vodeneev V. The electrical signal-induced systemic photosynthetic response is accompanied by changes in the photochemical reflectance index in pea. Funct Plant Biol. 2018;46:328–338. doi:10.1071/FP18224.
- Gamon JA, Peñuelas J, Field CB. A narrow-waveband spectral index that tracks diurnal changes in photosynthetic efficiency. Remote Sens Environ. 1992;41:35–44. doi:10.1016/0034-4257(92)90059-S.
- Surova L, Sherstneva O, Vodeneev V, Katicheva L, Semina M, Sukhov V. Variation potential-induced photosynthetic and respiratory changes increase ATP content in pea leaves. J Plant Physiol. 2016;202:57–64. doi:10.1016/j.jplph.2016.05.024.
- Sukhova E, Mudrilov M, Vodeneev V, Sukhov V. Influence of the variation potential on photosynthetic flows of light energy and electrons in pea. Photosynth Res. 2018;136:215–228. doi:10.1007/s11120-017-0460-1.
- Mancuso S. Hydraulic and electrical transmission of wound-induced signals in Vitis vinifera. Aust J Plant Physiol. 1999;26:55–61.
- Grams TEE, Lautner S, Felle HH, Matyssek R, Fromm J. Heat-induced electrical signals affect cytoplasmic and apoplastic pH as well as photosynthesis during propagation through the maize leaf. Plant Cell Environ. 2009;32:319–326. doi:10.1111/j.1365-3040.2008.01922.x.
- Sukhov V, Surova L, Sherstneva O, Bushueva A, Vodeneev V. Variation potential induces decreased PSI damage and increased PSII damage under high external temperatures in pea. Funct Plant Biol. 2015;42:727–736. doi:10.1071/FP15052.
- Knapp A, Carter G. Variability in leaf optical properties among 26 species from a broad range of habitats. Am J Bot. 1998;85:940–946.