ABSTRACT
Regulator of G-protein signaling (RGS) protein, the best-characterized accelerating GTPase protein in plants, regulates G-protein signaling and plays important role in abiotic stress tolerance. However, the detailed molecular mechanism of RGS involved in G-protein signaling mediated abiotic stress responses remains unclear. In this study, a mulberry (Morus alba L.) RGS gene (MaRGS) was transformed into tobacco, and the ectopic expression of MaRGS in tobacco decreased the tolerance to salt stress. The transgenic tobacco plants had lower proline content, higher malonaldehyde and H2O2 contents than wild type plants under salt stress condition. Meanwhile, MaRGS overexpression in mulberry seedlings enhances the sensitivity to salt stress and RNAi-silenced expression of MaRGS improves the salt stress response and tolerance. These results suggested that MaRGS negatively regulates salt stress tolerance. Further analysis suggested that D-glucose and autophagy may involve in the response of RGS to salt stress. This study revealed the role of MaRGS in salt stress tolerance and provides a proposed model for RGS regulates G-protein signaling in response to salt stress.
KEYWORDS:
Introduction
Signal transduction processes mediated by heterotrimeric guanine-nucleotide-binding protein (hereafter G-protein) complex consisting of Gα, Gβ and Gγ subunits play fundamental roles in regulating various cellular processes in all eukaryotes.Citation1,Citation2 In animals, when G-protein-coupled receptors (GPCRs) bind their cognate ligands and the activated GPCRs serve as guanine nucleotide exchange factors that stimulate the exchange of GDP for GTP on Gα subunit.Citation3 And then, G-protein was dissociated into Gα-GTP and a Gβγ dimer, and both of these components trigger diverse signaling pathways through interacting with their respective downstream effectors.Citation4
Unlike in animals, plants do not have canonical GPCRs and G-proteins are self-activated, and the regulation of G-protein activation in plants occurs at the deactivation step.Citation5 Regulator of G-protein signaling (RGS) protein, the best-characterized GTPase accelerating protein in plants, plays important role in regulating G-protein complex in its inactive state through accelerating GTP hydrolysis of Gα subunit.Citation6 The first RGS protein, called AtRGS1, was characterized in Arabidopsis, which has a seven-transmembrane domain at the N terminus and a cytoplasmic RGS box at the C terminus and stimulates the rate-limiting GTPase activity of Gα subunit.Citation7 After that, RGS proteins have been found in various plants, including the green alga Chara braunii, the lycophyte Selaginella moellendorffii, monocot and all eudicot plants.Citation8 Previous studies suggested that the interplay between RGS and Gα proteins is quite important in regulating G-protein mediated signaling pathways in plants, and RGS acts as a key point of ligand-dependent signal modulation of G-protein complex.Citation2,Citation9 In Arabidopsis, D-glucose recruits the With No Lysine kinases (WNKs), AtWNK1, AtWNK8 and AtWNK10, to phosphorylate AtRGS1 and then the phosphorylated AtRGS1 was internalized, which uncouples AtGPA1 from AtRGS1 and causes the subsequent sustained activation of G-protein signaling.Citation10,Citation11 It is suggested that autophagy pathway may play an important role in regulating AtRGS1 endocytosis after D-glucose stimulation, and the endocytosis of AtRGS1 was inhibited after the autophagy pathway was interrupted.Citation12 A previous study reported that at least 10 of 70 detected leucine-rich repeat receptor-like kinases (LRR RLKs) attribute to phosphorylate AtRGS1, which results in AtRGS1 endocytosis.Citation9 Therefore, several LRR RLKs were proposed to regulate the activity of AtRGS1 through phosphorylating AtRGS1 in a ligand-dependent manner.Citation9 In soyabean, nod factor receptor 1, a lysM-type receptor kinase, phosphorylates and activates RGS proteins, which help to maintain the G proteins in their inactive state and then result in nodule development.Citation13 Additionally, some other regulators in Arabidopsis, such as phosphatidic acid and RGS1-HXK1 interacting protein (RHIP1), modulate RGS-mediated regulation of G-protein signaling pathway by regulating RGS activity.Citation1,Citation14
RGS proteins regulate many aspects of the plant life, including morphogenesis, cell growth and proliferation, seed germination, stomatal movements, sugar sensing, ion channel regulation, and abscisic acid and environmental stress responses.Citation7,Citation15–Citation19 Previous studies suggested that RGS plays important role in tolerance to abiotic stresses. The overexpression of Arabidopsis AtRGS1 enhances the tolerance of the transgenic plants to drought stress through changing the expression levels of genes involved in ABA biosynthesis.Citation16 The loss of AtRGS1 confers hyposensitivity to saline stress.Citation19 However, little is known about the detailed molecular mechanisms of RGS involved in abiotic stress tolerance.
Mulberry (Morus alba L.), an economic and ecological perennial tree, can adapt well to adverse abiotic stresses, especially saline condition, but the molecular mechanisms responsible for salt stress remain unclear. We previously identified a RGS-encoding gene (MaRGS) from mulberry and preliminarily analyzed its functions in abiotic stress responses.Citation20 Here, MaRGS was transformed into tobacco (Nicotiana tabacum L.) and the salt stress tolerances of the transgenic plants were detected. The overexpression of MaRGS decreased the tolerance to salt stress in tobacco. The mulberry seedlings transiently overexpressing MaRGS are hypersensitive to salt stress, while RNAi-silenced expression of MaRGS improves the salt stress tolerance. Moreover, our data suggested that D-glucose and autophagy may regulate RGS protein in response to salt stress. Overall, our results suggest a proposed pathway for RGS regulates G-protein signaling in plant tolerance to salt stress.
Results and discussion
G-proteins play important roles in abiotic stress responses and tolerances, including salt stress. Studies in Arabidopsis, rice (Oryza sativa L.), maize (Zea mays L.) and mulberry suggested that G-proteins act as the key regulatory factors in response to salt stress,Citation4,Citation19,Citation21,Citation22 but how do G-proteins respond, recognize and transduce stress signal under salt stress remains unclear. RGS protein acts as a GTPase-activating protein and plays critical role in plant G-protein cycle regulation, which indicates that RGS protein may be significant for G-protein in response to salt stress.
To evaluate the role of RGS protein in tolerance to salt stress, the full-length sequence of a RGS gene from mulberry was transformed into wild type (WT) tobacco plants. In total, four independent MaRGS transgenic lines were obtained using β-D-glucosidase (GUS) staining, genomic PCR and qRT-PCR analysis (). Finally, MaRGS lines 2, 3 and 4 were selected for further analysis. The 7-d-old seedlings were cultivated in 1/2 MS (Murashige and Skoog) agar medium containing 200 mM NaCl, the roots of MaRGS line 3 and 4 were shorter than the WT roots under salt stress condition (,c)). The 5-week-old transgenic plants showed relatively worse growth than WT plants under 200 mM NaCl treatment for 10 d ()). The H2O2 and MDA contents were higher, while proline content was lower in MaRGS transgenic plants than WT tobaccos under salt stress condition (-f)). These data suggested the constitutive overexpression of MaRGS in tobacco is adverse to resist salt stress. In this study, the pFGC:MaRGS-OE and pFGC:MaRGS-RNAi vectors were transiently transformed into the mulberry seedlings and generated the MaRGS-overexpressing and RNAi-silenced MaRGS plants, respectively. Compared with control plants (transiently transformed with empty pFGC5941 vector), the expression of MaRGS increased in the overexpressing plants while decreased in the RNAi-silenced MaRGS plants ()). The H2O2 content was higher in MaRGS-overexpressing plants and lower in RNAi-silenced MaRGS plants ()). The proline content was lower in MaRGS-overexpressing plants but was higher in RNAi-silenced MaRGS plants ()). Above data suggested that MaRGS may be a negative factor in salt stress tolerance, which is similar to the role of Arabidopsis AtRGS1 in salt stress response.Citation19
Figure 1. Confirmation of transgenic tobacco plants.
(a) Genomic PCR analysis and histochemical GUS staining of transgenic lines. (b) Quantitative real-time PCR. Data are means ± SDs (n = 3), P < .05. Significant differences are indicated by different letters above the bars.
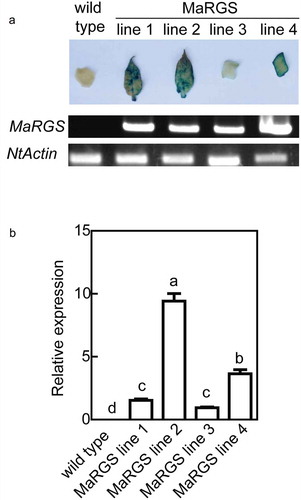
Figure 2. Salt stress tolerance analysis of MaRGS transgenic tobacco plants.
(a) The root growth of MaRGS transgenic tobacco and WT plants under normal and stress conditions. (b) The growth of MaRGS transgenic tobacco and WT plants under normal and stress conditions. (c) Statistical analysis of root lengths of MaRGS transgenic tobacco and WT plants. Data are means ± SDs (n = 15), *P < .05. (d–f) The H2O2 content (d), MDA content (e) and proline content (f) in MaRGS transgenic tobacco and WT plants under normal and stress conditions. Data are means ± SDs (n = 3), *P < .05.
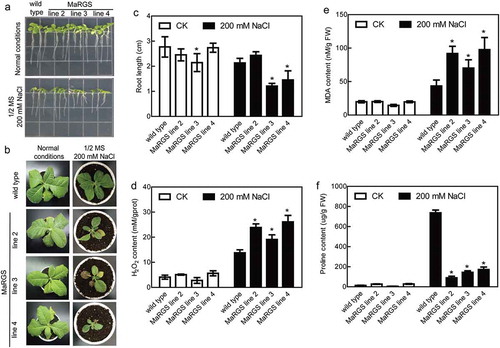
Figure 3. Salt stress analysis of MaRGS in mulberry by transient expression.
(a) Analysis of the expression of MaRGS in the control, MaRGS-overexpressing and RNAi-silenced MaRGS plants. (b,c) The H2O2 content (b) and proline content (c) in the control, MaRGS-overexpressing and RNAi-silenced MaRGS plants. Data are means ± SDs (n = 3), P < .05. Significant differences are indicated by different letters above the bars
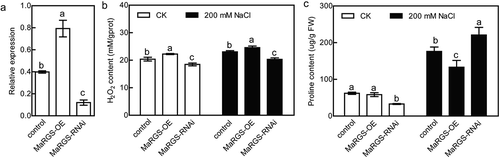
To survive under salt stress condition, how do plants respond to stress through regulating RGS protein? Previous study in Arabidopsis showed that NaCl may activate G-protein signaling to improve stress survival via promoting AtRGS1 internalization,Citation19 so it is proposed that the endocytosis of RGS protein may help plants tolerate salt stress. In Arabidopsis, D-glucose recruits AtWNK kinases to phosphorylate AtRGS1, which induces the internalization of AtRGS1.Citation10,Citation11 Additionally, the endocytosis of AtRGS1 is also regulated by the autophagy pathway after D-glucose stimulation,Citation12 and the autophagy is rapidly induced by salt stress and is required for salt tolerance.Citation23 Therefore, D-glucose and autophagy may regulate salt stress response by inducing the endocytosis of RGS. In this study, mulberry seedlings and tobacco plants were independently treated with 200 mM NaCl, and then, their soluble sugar contents were determined. The soluble sugar contents in mulberry and tobacco were significantly increased by NaCl treatment (,b)). The qRT-PCR analysis showed that the expression levels of the WNK genes, MaWNK1 and MaWNK8, and the autophagy-related (ATG) genes, MaATG7 and MaATG8, were significantly regulated by NaCl and D-glucose treatments ()). Tobacco NtWNK8, NtWNK9, NtATG6, NtATG8a and NtATG8f also respond to NaCl and D-glucose treatments ()). In addition, the expression levels of the MaWNK and MaATG genes are more sensitive to D-glucose in the MaRGS-overexpressing mulberry seedlings than control plants, but the expression levels of these genes were inhibited in the RNAi-silenced MaRGS plants ()). The expression levels of the NtWNK and NtATG genes respond to D-glucose treatments in the tobacco plants overexpressing the MaRGS gene ()). Above results indicated that D-glucose and autophagy may regulate the RGS-mediated salt stress response, but more studies are needed to investigate it.
Figure 4. The soluble sugar content and the expression levels of ATG and WNK genes in mulberry and tobacco.
(a,b) The soluble sugar contents in mulberry (a) and tobacco (b) after NaCl treatment. (c,d) The expression levels of WNK and ATG genes of mulberry (c) and tobacco (d) in response to NaCl and D-glucose treatments. (e) The fold change of MaWNK1, MaWNK8, MaATG8 and MaATG7 expression in the mulberry seedlings transiently transformed with pFGC:MaRGS-OE and pFGC:MaRGS-RNAi after D-glucose treatment. (f) The fold change of NtATG8f, NtATG6, NtATG8a, NtWNK8 and NtWNK9 expression in the transgenic tobacco plants overexpressing MaRGS after D-glucose treatment. Data are means ± SDs (n = 3), *P < .05.
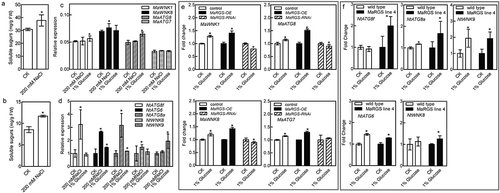
In conclusion, the role of mulberry RGS in response to salt stress was revealed and our study indicated that MaRGS negatively regulates salt stress response and tolerance. Meanwhile, the above analysis suggested a proposed pathway for RGS in response to salt stress (). When plants are exposed to salt stress, the accumulations of D-glucose and ATG proteins are induced, and WNK proteins are recruited. Then, RGS is internalized and the G-protein α subunit is uncoupled from RGS. G-proteins are subsequently dissociated into Gα-GTP and a Gβγ dimer. The Gβγ dimer may eventually promote the activation of downstream stress-responsive proteins and ROS detoxification.Citation4,Citation22 However, more studies are needed to elucidate the mechanisms of RGS-mediated G-protein signaling in response to salt stress. Overall, our data will help improve the understanding of G-protein and RGS mediated salt stress tolerance in plants.
Figure 5. A possible model for RGS-regulated G-protein signaling in response to salt stress.
(a) Under normal conditions, the inactivated and activated states of G-proteins are balanced, and Gα and Gβγ subunits perform negative and positive roles, respectively, in plant response to salt stress. (b) When plants are exposed to salt stress, the accumulation of D-glucose and ATG proteins are induced, and WNK proteins are also recruited. This leads to the endocytosis of RGS and the dissociation of heterotrimeric G-proteins. Gβγ dimers eventually promote plant tolerance to salt stress.
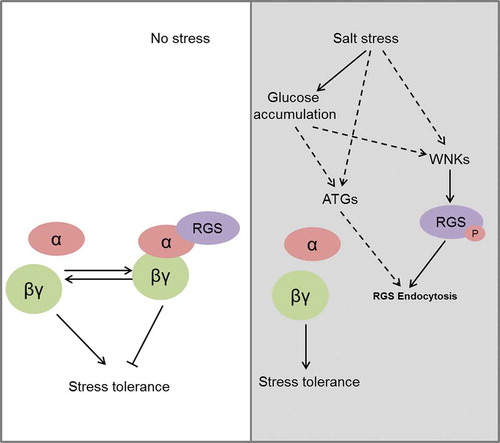
Methods and methods
Tobacco transformation
MaRGS (KX099868) gene was cloned into the pLGNL expression vector and the recombinant plasmid was transformed into Agrobacterium tumefaciens strain GV3101. The A. tumefaciens harboring the transgenic plasmid was transformed into tobacco (K326) plants using the leaf disc method. The methods of transformation and identification of transgenic plants are similar to those described in previous studies.Citation4,Citation24
Stress tolerance analysis of transgenic tobacco plants
For the root growth analysis, the 7-d-old seedlings were incubated in 1/2 MS agar medium supplemented with 200 mM NaCl for 7 d, and the root growth was recorded. The 5-week-old plants were treated with 200 mM NaCl until significant differences appeared between the transgenic lines and WT tobacco. The stress treatment methods, measurements of physiological parameters, and statistical analysis as described in our previous study.Citation4
Stress tolerance analysis of MaRGS in mulberry by transient expression assays
The full-length and an inverted repeat cDNA fragment of MaRGS gene were inserted into pFGC5941 expression vector and generated the pFGC:MaRGS-OE and pFGC:MaRGS-RNAi vectors, respectively. And then, the recombinant plasmids were transformed into A. tumefaciens strain GV3101. The A. tumefaciens harboring pFGC:MaRGS-OE and pFGC:MaRGS-RNAi vectors were independently transformed into the 35-d-old mulberry seedlings using a transient transformation system,Citation25 which can be used for functional analysis of genes involved in stress tolerances.Citation26 After Agrobacterium treatment, the seedlings were grown on 1/2 MS agar medium (3% (w/v) sucrose, pH 5.8) for 48 h. Finally, the seedlings were incubated in 1/2 MS agar medium containing 200 mM NaCl for 24 h, and the treated seedlings were collected for detection of physiological parameters.
Measurement of the soluble sugar content
The 2-month-old mulberry seedlings were treated with water containing 200 mM NaCl for 14 d. The 5-week-old tobacco plants were treated with 200 mM NaCl for 7 d. Each treatment was replicated three times. The soluble sugar content of the treated seedlings was determined using a plant soluble sugar content test kit (Jiancheng Bioengineering Institute, Nanjing, China) according to the manufacturer’s instructions.
Real-time quantitative reverse transcription PCR (qRT-PCR)
The 14-d-old mulberry seedlings were treated with water containing 200 mM NaCl and 1% (w/v) D-glucose for 30 min. The 4-week-old tobacco plants were treated with water containing 200 mM NaCl for 1 h, and were treated with water containing 1% D-glucose for 30 min. The treated seedlings were collected for total RNA extraction using TRIzol reagent (Invitrogen, Carlsbad, CA, USA). The first-strand cDNA synthesis and qRT-PCR analysis were performed as described in a previous study.Citation27 MaACTIN3 (HQ163775) and NtActin (U60489) were used as internal controls for mulberry and tobacco, respectively, and the relative expression was defined as 2−[Ct(target gene) Ct(control gene)]. The primers used are specified in Supplemental Table 1.
Statistical analysis
The statistical analysis methods as described in our previous study.Citation4 The results were analyzed by Excel 2013 (Microsoft, Redmond, USA) and GraphPad Prism 6.0 (GraphPad Software, La Jolla, USA). The results are presented as mean values ± SDs. Comparisons among means were conducted using Duncan’s test calculated at P < .05.
Disclosure of potential conflicts of interest
No potential conflicts of interest are disclosed.
Supplemental Material
Download MS Word (19.7 KB)Supplemental material
Supplemental data for this article can be accessed on the publisher’s website.
Additional information
Funding
References
- Choudhury SR, Pandey S. Phosphatidic acid binding inhibits RGS1 activity to affect specific signaling pathways in Arabidopsis. Plant J. 2017;90:1–6. doi:10.1111/tpj.13503.
- Kumar R, Bisht NC. Duplicated RGS (Regulator of G-protein signaling) proteins exhibit conserved biochemical but differential transcriptional regulation of heterotrimeric G-protein signaling in Brassica species. Sci Rep. 2018;8:2176. doi:10.1038/s41598-018-20500-3.
- Wang Y, Wang Y, Deng D. Multifaceted plant G protein: interaction network, agronomic potential, and beyond. Planta. 2019;249:1259–1266. doi:10.1007/s00425-019-03112-7.
- Liu C, Xu Y, Feng Y, Long D, Cao B, Xiang Z, Zhao A. Ectopic expression of mulberry G-proteins alters drought and salt stress tolerance in tobacco. Int J Mol Sci. 2019;20:89. doi:10.3390/ijms20010089.
- Urano D, Jones AM. Heterotrimeric G protein-coupled signaling in plants. Annu Rev Plant Biol. 2014;65:365–384. doi:10.1146/annurev-arplant-050213-040133.
- Stateczny D, Oppenheimer J, Bommert P. G protein signaling in plants: minus times minus equals plus. Curr Opin Plant Biol. 2016;34:127–135. doi:10.1016/j.pbi.2016.11.001.
- Chen JG, Willard FS, Huang J, Liang J, Chasse SA, Jones AM, Siderovski DP. A seven-transmembrane RGS protein that modulates plant cell proliferation. Science. 2003;301:1728–1731. doi:10.1126/science.1087790.
- Hackenberg D, McKain MR, Lee SG, Choudhury SR, McCann T, Schreier S, Harkess A, Pires JC, Wong GK, Jez JM, et al. Gα and regulator of G-protein signaling (RGS) protein pairs maintain functional compatibility and conserved interaction interfaces throughout evolution despite frequent loss of RGS proteins in plants. New Phytol. 2017;216:562–575. doi:10.1111/nph.14180.
- Tunc-Ozdemir M, Urano D, Jaiswal DK, Clouse SD, Jones AM. Direct modulation of heterotrimeric G protein-coupled signaling by a receptor kinase complex. J Biol Chem. 2016;291:13918–13925. doi:10.1074/jbc.C116.736702.
- Urano D, Phan N, Jones JC, Yang J, Huang JR, Grigston J, Taylor JP, Jones AM. Endocytosis of the seven-transmembrane RGS1 protein activates G-protein-coupled signalling in Arabidopsis. Nat Cell Biol. 2012;14:1079–1088. doi:10.1038/ncb2568.
- Fu Y, Lim S, Urano D, Tunc-Ozdemir M, Phan NG, Elston TC, Jones AM. Reciprocal encoding of signal intensity and duration in a glucose-sensing circuit. Cell. 2014;156:1084–1095. doi:10.1016/j.cell.2014.01.013.
- Yan QQ, Wang JC, Fu ZQ, Chen WL. Endocytosis of AtRGS1 is regulated by the autophagy pathway after D-glucose stimulation. Front Plant Sci. 2017;8:1229. doi:10.3389/fpls.2017.01229.
- Choudhury SR, Pandey S. Phosphorylation-dependent regulation of G-protein cycle during nodule formation in soybean. Plant Cell. 2015;27:3260–3276. doi:10.1105/tpc.15.00517.
- Huang JP, Tunc-Ozdemir M, Chang Y, Jones AM. Cooperative control between AtRGS1 and AtHXK1 in a WD40-repeat protein pathway in Arabidopsis thaliana. Front Plant Sci. 2015;6:851. doi:10.3389/fpls.2015.00851.
- Chen JG, Jones AM. AtRGS1 function in Arabidopsis thaliana. Methods Enzymol. 2004;389:338–350. doi:10.1016/S0076-6879(04)89020-7.
- Chen Y, Ji F, Xie H, Liang J. Overexpression of the regulator of G-protein signalling protein enhances ABA-mediated inhibition of root elongation and drought tolerance in Arabidopsis. J Exp Bot. 2006;57:2101–2110. doi:10.1093/jxb/erj167.
- Chen Y, Ji F, Xie H, Liang J, Zhang J. The regulator of G-protein signaling proteins involved in sugar and abscisic acid signaling in Arabidopsis seed germination. Plant Physiol. 2006;140:302–310. doi:10.1104/pp.105.069872.
- Fan LM, Zhang W, Chen JG, Taylor JP, Jones AM, Assmann SM. Abscisic acid regulation of guard-cell K+ and anion channels in Gβ- and RGS-deficient Arabidopsis lines. Proc Natl Acad Sci U S A. 2008;105:8476–8481. doi:10.1073/pnas.0800980105.
- Colaneri AC, Tunc-Ozdemir M, Huang JP, Jones AM. Growth attenuation under saline stress is mediated by the heterotrimeric G protein complex. BMC Plant Biol. 2014;14:129. doi:10.1186/1471-2229-14-129.
- Liu CY, Xu YZ, Fan W, Long DP, Cao BN, Xiang ZH, Zhao AC. Identification of the genes involved in heterotrimeric G-protein signaling in mulberry and their regulation by abiotic stresses and signal molecules. Biol Plantarum. 2018;62:277–286. doi:10.1007/s10535-018-0779-2.
- Urano D, Colaneri A, Jones AM. Gα modulates salt-induced cellular senescence and cell division in rice and maize. J Exp Bot. 2014;65:6553–6561. doi:10.1093/jxb/eru372.
- Swain DM, Sahoo RK, Srivastava VK, Tripathy BC, Tuteja R, Tuteja N. Function of heterotrimeric G-protein γ subunit RGG1 in providing salinity stress tolerance in rice by elevating detoxification of ROS. Planta. 2016;245:367–383. doi:10.1007/s00425-016-2614-3.
- Luo L, Zhang P, Zhu R, Fu J, Su J, Zheng J, Wang Z, Wang D, Gong Q. Autophagy is rapidly induced by salt stress and is required for salt tolerance in Arabidopsis. Front Plant Sci. 2017;8:1459. doi:10.3389/fpls.2017.01459.
- Liu XQ, Liu CY, Guo Q, Zhang M, Cao BN, Xiang ZH, Zhao AC. Mulberry transcription factor MnDREB4A confers tolerance to multiple abiotic stresses in transgenic tobacco. PLoS One. 2015;10:e0145619. doi:10.1371/journal.pone.0145619.
- Zheng L, Liu G, Meng X, Li Y, Wang Y. A versatile Agrobacterium-mediated transient gene expression system for herbaceous plants and trees. Biochem Genet. 2012;50:761–769. doi:10.1007/s10528-012-9518-0.
- Ji X, Zheng L, Liu Y, Nie X, Liu S, Wang Y. A transient transformation system for the functional characterization of genes involved in stress response. Plant Mol Biol Rep. 2014;32:732–739. doi:10.1007/s11105-013-0683-z.
- Wei C, Liu X, Long D, Guo Q, Fang Y, Bian C, Zhang D, Zeng Q, Xiang Z, Zhao A. Molecular cloning and expression analysis of mulberry MAPK gene family. Plant Physiol Biochem. 2014;77:108–116. doi:10.1016/j.plaphy.2014.02.002.