ABSTRACT
In higher plants, male meiosis is a key process of microsporogenesis and is crucial for plant fertility. Male meiosis programs are prone to be influenced by altered temperature conditions. Studies have reported that an increased temperature (28°C) within a fertile threshold can affect the frequency of meiotic recombination in Arabidopsis. However, not much has been known how male meiosis responses to an extremely high temperature beyond the fertile threshold. To understand the impact of extremely high temperature on male meiosis in Arabidopsis, we treated flowering Arabidopsis plants with 36-38°C and found that the high-temperature condition significantly reduced pollen shed and plant fertility, and led to formation of pollen grains with varied sizes. The heat stress-induced unbalanced tetrads, polyad and meiotic restitution, suggesting that male meiosis was interfered. Fluorescence in situ hybridization (FISH) assay confirmed that both homologous chromosome separation and sister chromatids cohesion were influenced. Aniline blue staining of tetrad-stage pollen mother cells (PMCs) revealed that meiotic cytokinesis was severely disrupted by the heat stress. Supportively, immunolocalization of ɑ-tubulin showed that the construction of spindle and phragmoplast at both meiosis I and II were interfered. Overall, our findings demonstrate that an extremely high-temperature stress over the fertile threshold affects both chromosome segregation and cytokinesis during male meiosis by disturbing microtubular cytoskeleton in Arabidopsis.
Introduction
Meiosis is a specialized type of cell division during which DNA duplicates once while chromosomes separate twice.Citation1 At prophase of meiosis I, meiotic recombination occurs which ensures the reciprocal exchange of genetic information between parental chromosomes and allows the novel combination of gene alleles. Initiation of meiotic recombination is launched by the formation of double-strands breaks (DSBs) that is catalyzed by a highly conserved topoisomerase VI protein SPO11.Citation2 In Arabidopsis, both SPO11-1 and SPO11-2 are required for meiotic recombination.Citation3–Citation5 Thereafter, recombinases RAD51 and DMC1 process the DSBs for recombination, with RAD51 acting as an accessory factor of DMC1.Citation6–Citation9 After the completion of meiotic recombination, homologous chromosomes separate at anaphase of meiosis I. At early stages of meiosis II, the centromeric cohesions between sister chromatids are still retained, and are released at anaphase II, leading to the disjoining of sister chromatids at the end of meiosis with a resultant four haploid chromosome sets, reviewed by .Citation10 In Arabidopsis, many key cohesion factors, including AtSMC1, AtSMC3, SYN1 (AtRec8), AtSCC3 and AtSGO1, have been identified and characterized.Citation11–Citation16 Meiotic cytokinesis takes place afterward of chromosome segregation, while dicot plants and monocot plants have distinct meiotic cytokinesis patterns. For dicot plants, i.e., Arabidopsis, meiotic cell walls are formed after the separation of sister chromatids at the end meiosis II, and this pattern is named ‘simultaneous cytokinesis.’Citation17 In contrast, monocot plants, e.g., rice and maize, undergo successive-type of cytokinesis. For this kind of meiotic cytokinesis, each round of chromosome segregation is followed by a construction of cell wall formation, which leads to a dyad formation after meiosis I and a tetrad formation after meiosis II.Citation17 In Arabidopsis, male meiotic cytokinesis is regulated by a PAMK signaling cascade AtNACK2/TES/STUD-AtANP3-AtMKK6-AtMPK4.Citation18–Citation22
Male meiosis in plants is sensitive to either increase or decrease of environment temperatures.Citation23–Citation25 In both dicot and monocot plants, cold stress has been found to interfere with cytoskeleton organization and cell wall formation during male meiosis and consequently lead to the production of unreduced gametes.Citation26–Citation28 In addition, studies have reported that a minor decrease of temperature (e.g., 19°C) can influences the frequency of crossover (CO) in Arabidopsis.Citation29,Citation30 Compared with cold stress, high-temperature stress seems to affect more aspects of meiosis. Male meiotic progression is interfered in hexaploid wheat (Triticum aestivum L.) incubated shortly under high temperatures (30 and/or 35°C).Citation31 An increased but within fertile threshold temperature (28°C) causes a higher meiotic recombination rate in A. thaliana, which was shown to act through the class I CO pathway.Citation29,Citation32,Citation33 Higher temperatures beyond fertile range have been found to influence chromatin configuration at early meiotic stages, and induces polyploid and/or aneuploid spore formation.Citation34,Citation35 Nevertheless, the cellular mechanism underpinning how plant male meiosis responses to an extremely increased temperature that impairs plant fertility, is not understood yet. To this end, we studied the impact of a high-temperature condition (36–38°C) on male meiosis in Arabidopsis. We found that the heat stress severely impairs pollen shed and plant fertility. Cytological analysis of developing PMCs revealed that the heat stress interferes with both chromosome segregation and cytokinesis, probably by impacting the construction of tubulin-dependent spindle and phragmoplast. This study contributes to the understanding how plant male meiosis responses to high-temperature stress.
Results
Heat stress reduces plant fertility and induces pollen grains with varied sizes
To examine the impact of extremely high temperature on Arabidopsis, flowering wild-type Col-0 plants were incubated at 36–38°C, which has been shown to severely influence the seed set in plants, Citation36–Citation38 for 24 h and were transferred back to 20°C for recovery. After 10 d post the heat stress, we checked the silique development and counted the number of seeds in the influenced siliques. Compared with control plants, which displayed normal silique development (), heat-stressed plants produced shorter siliques on both main shoots and lateral shoots (, see red arrow). The number of seeds yielded in the heat-affected siliques was significantly reduced (). Alexander staining was then performed to check the pollen development at 5 d later upon the heat treatment. In contrast to control anthers, the 24 h-heat stress severely reduced pollen shed (). The 12 h heat treatment influenced pollen shed slightly, and the 6 h treatment did not show an obvious impact (). Remarkably, pollen grains with different sizes were observed in all the three treatment conditions (, see blue boxes). Germination assay was then performed to verify the bioactivity of pollen grains with different sizes. At 24 h later after setting on germination medium, pollen grains from control plants germinated and produced pollen tubes (). However, differently sized-pollen grains from 24 h heat-stressed plants did not germinate (). Overall, these findings indicated that the heat stress significantly affects male sterility and the seed set of Arabidopsis. Additionally, since differently sized-pollen grains could have different chromosome numbers, Citation39 earlier processes during microsporogenesis were likely to be impacted by the heat stress.
Figure 1. Heat stress reduces pollen viability and plant fertility. (a), Siliques from main shoots and lateral shoots in control plants and heat-stressed plants. Red arrows indicate short siliques induced by the heat stress. Scale bar = 5 cm. (b), Histogram showing the average number of seeds yielded by the siliques of control plants and the heat-stressed plants. For both control and heat stress conditions, three individuals were used for quantification. Error bars represent standard deviation. (c), Histogram showing the diameter of 10 pollen grains produced by control, 24 h-stressed, 12 h-stressed and 6 h-stressed plants. (d–g), Alexander staining of anthers in control plants (d), and in the plants treated by 24 h (e), 12 h (f) and 6 h (g) heat stresses. Blue boxes indicate the pollen grains with different sizes. Scale bar = 100 μm. (h–k), Germinated pollen grain in control plant (h), and non-germinated pollen grains with varied sizes from the 24 h heat-stressed plants (i–k). n indicates the number of observed pollen grains with corresponding phenotypes. Scale bar = 20 μm.
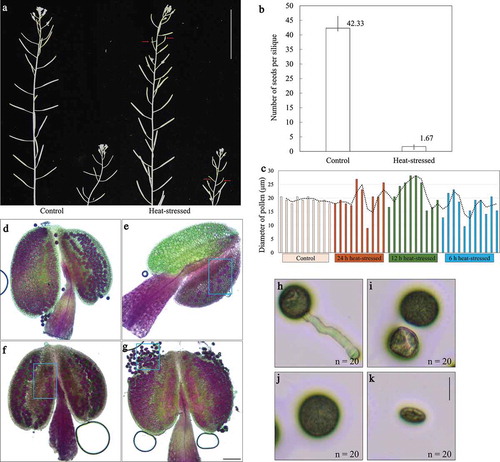
Heat stress induces aberrant tetrad formation
To elucidate whether heat-induced pollen grains with varied sizes were caused by alterations in male meiosis, we analyzed tetrad stage PMCs by performing orcein staining. Flowering Col-0 plants were treated by 36–38°C for 24 h, and were transferred back to 20°C for 6 h recovery. In control plants, PMCs produced normal tetrads with four equal-sized spores (). However, in the heat-stressed plants, about 56% irregular meiotic products were observed (). Unbalanced-triads, which harbor two or more nuclei in one spore, occupied the highest proportion among the abnormal products (, around 34%; F and G). Unbalanced-dyads occurred at 9.21% (). Balanced-dyads (), unbalanced-tetrads that contain four spores but with more than one nucleus in each spore (), and polyads (), were consistently visualized, either, and occurred at a same frequency (, 3.95%). Group of multiple nuclei with varied sizes in one spore () and occurrence of polyad () suggested that chromosome segregation is unbalanced. Dyad formation () indicated that meiotic cytokinesis is affected. These figures thus suggest that the heat stress-induced pollen grains with different sizes originated from these abnormal meiotic products, and the chromosome dynamics and/or cytokinesis during male meiosis were influenced.
Figure 2. Heat stress induces abnormal meiotic products. (a), Pie chart showing the frequency of meiotic products from control plants and heat-stressed plants. For control 50 meiotic products were counted, and for heat stress treatment 76 meiotic products were quantified. (b–h), Orcein staining of tetrad in control plant (b), and unbalanced-tetrads with multiple nuclei in one spore (c), unbalanced-dyad (d), polyad (e), unbalanced-triad with multiple nuclei in one spore (f,g), and balanced-dyad (h) in the heat-stressed plants. Scale bar = 10 μm.
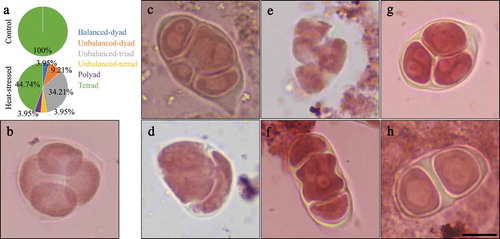
Heat stress induces unbalanced chromosome segregation
To elucidate the impact of heat stress on male meiosis, we analyzed the chromosome dynamics by performing fluorescence in situ hybridization (FISH) on meiotic spread using a centromere-specific DNA probe. At diakinesis and metaphase Ι, control plants displayed five pairs of bivalents with paired centromeric foci that represent a full synapsis of homologous chromosomes and a regular formation of CO (). In contrast, the heat-stressed plants exhibited 10 univalents together with 10 centromeric foci, which suggested that either homology pairing and/or synapsis, or meiotic recombination are compromised by the heat stress (). The formation of univalents caused unbalanced separation of homologous chromosomes after meiosis Ι (); moreover, centromeric foci with a number over 10 at anaphase Ι and interkinesis were occasionally observed, which suggested an occurrence of premature loss of sister chromatid cohesion (). Finally, at metaphase ΙΙ and telophase ΙΙ, unbalanced chromosome sets were formed in the heat-stressed plants (). These data demonstrate that the heat stress interferes with chromosome dynamics of male meiosis and leads to unbalanced chromosome segregation.
Figure 3. Centromeric FISH analysis of heat-stressed PMCs. A-F, Diakinesis- (a), metaphase Ι- (b), anaphase Ι- (c), interkinesis- (d), metaphase ΙΙ- (e) and telophase ΙΙ-stage (f) PMCs in control plants. G-L, diakinesis- (g), metaphase Ι- (h), anaphase Ι- (i), interkinesis- (j) metaphase ΙΙ- (k) and telophase ΙΙ-stage (l) PMCs in heat-stressed plants. Chromosomes are stained by DAPI, and centromeres are labeled by centromere-specific cen180_oligo2/5/6 probe, and displayed by green. Bifluorescence are shown by merged cells. Numbers displayed in the Centromere columns represent the number of chromosomes. n in the Merged columns indicates the number of cells observed with the corresponding phenotypes. Scale bars = 10 μm.
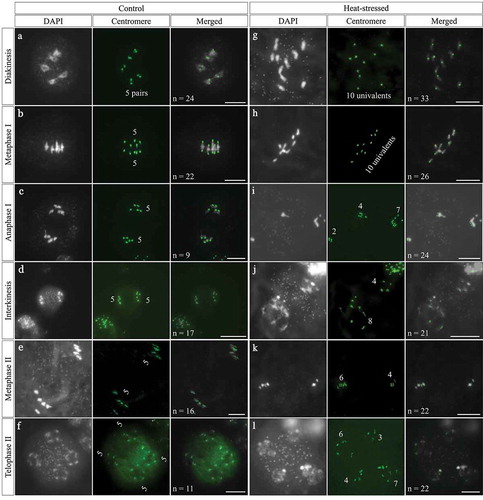
Heat stress interferes with male meiotic cytokinesis
Next, we analyzed meiotic cell wall formation by performing aniline blue staining that marks callosic cell walls to examine the impact of heat stress on male meiotic cytokinesis. Tetrads in control plants displayed ‘cross’-shaped meiotic cell walls between the four separated nuclei (); in contrast, the PMCs from heat-stressed plants produced abnormal meiotic products with unbalanced cell walls (). The smaller and larger cavities represented spores containing different chromosome numbers, which were supportive for the chromosome segregation defects. Moreover, we found that the callosic cell walls in the heat-induced aberrant products were thicker than that in the control tetrads (). These findings revealed that the high-temperature stress interferes with male meiotic cytokinesis.
Heat stress influences the construction of microtubule cytoskeleton
The formation of meiotic cell walls relies on the construction of cytoskeleton between the separated nuclei.Citation26 In order to clarify the cause of abnormal meiotic cytokinesis upon the heat stress, we performed immunolocalization of ɑ-tubulin in both control and heat-stressed PMCs. In control plants, homologous chromosomes are fully paired at pachytene (), and COs are formed at diakinesis, when five bivalents are visible and microtubular network is constructed surrounding the chromosomes (). At metaphase Ι, a spindle structure is shaped at two sides of aligned chromosomes (), which pulls the homologous chromosomes to the opposite polar of the cell at anaphase Ι (). Phragmoplast is temporarily formed between the separated homologies after meiosis Ι (). At metaphase ΙΙ, two pairs of spindles are constructed () and conduct the disjoining of sister chromatids, which consequently contributes to tetrad formation, when mini-phragmoplast; i.e., radial microtubule arrays are generated surrounding the haploid nuclei (). Balanced chromosome segregation and complete meiotic cytokinesis during male meiosis lead to haploid spore production ().
Figure 5. Microtubular cytoskeleton in PMCs of control plants. A-H, PMCs at pachytene (a), diakinesis (b), metaphase Ι (c), anaphase Ι (d), interkinesis (e), metaphase ΙΙ (f), tetrad (g) and unicellular microspore (h) stages in control plants. White: DAPI signal; green: ɑ-tubulin signal. Scale bar = 10 μm.
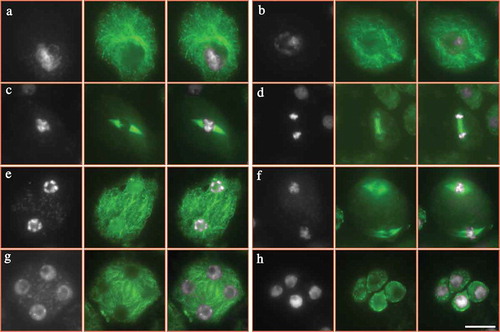
On the contrary, heat-stressed plants displayed alterations in the microtubular cytoskeleton formation. At early stages of male meiosis, the heat stress seemed to have no obvious impact on the construction of microtubule around the chromosomes (). However, at metaphase Ι, irregular spindle with reduced microtubule abundance and dotted fibers was observed (); and occasionally the typical bipolar configuration of spindle was not formed with the chromosomes linked by linear microtubules (). This suggests that the heat stress severely impaired the formation and/or stability of spindle at meiosis Ι. The mis-segregation of chromosomes was thereafter induced with the chromosomes unevenly grouped at anaphase Ι (). These aneuploid chromosome sets are likely to develop into the mini-nuclei at later stages (, see red arrows). ‘Extra’ phragmoplast was found to construct between the aneuploid chromosome sets at anaphase Ι (, see yellow arrows). These ‘extra’ phragmoplast would define the location of cell walls and therefore support the observation by aniline blue staining (). Meanwhile, significantly reduced microtubule fibers occurred suggesting that the phragmoplast stability was influenced, either (). Mini-chromosome groups underwent nucleation at interkinesis (, see red arrows). At metaphase ΙΙ, spindle-like structures were visualized but were incomplete and the direction was abnormal (). Unbalanced chromosome sets occurred either at telophase ΙΙ and irregular phragmoplasts were formed between the dispersive chromosome groups (). At tetrad stage, both adjacent nuclei and multiple nuclei were found, and for the former case a complete omission of RMAs occurred between the unseparated nuclei which indicates for meiotic restitution ( and M, see yellow arrows). Consequently, aneuploid microspores were yielded (). These findings demonstrate that the high-temperature stress has a significant impact on microtubular cytoskeleton during male meiosis, and suggests that the meiotic cytokinesis defects result from the misstructured microtubules.
Figure 6. Microtubular cytoskeleton in PMCs of heat-stressed plants. A-N, PMCs at pachytene (a), diakinesis (b), metaphase Ι (c–e), anaphase Ι (f,g), interkinesis (h,i), metaphase ΙΙ (j), telophase ΙΙ (k), tetrad (l,m) and unicellular microspore (n) stages in heat-stressed plants. White: DAPI signal; green: ɑ-tubulin signal. Red arrows indicate mini-nucleus (h,i,l), and yellow arrows indicate extra phragmoplast or omission of RMAs (f,i,l). Scale bar = 10 μm.
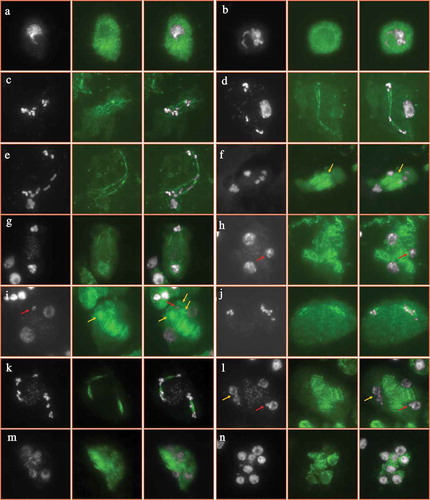
Discussion
In flowering plants, male meiosis ensures the stability of genome ploidy of life cycle by halving the chromosome number to generate haploid gametes. Alterations occurring during meiosis either cause unbalanced chromosome segregation that leads to aneuploid gametes and impaired plant fertility, or evoke meiotic restitution with resultant unreduced gametes.Citation14,Citation40 As sessile organisms, plants are prone to be influenced by environmental conditions, and the male reproductive development is especially sensitive to temperature changes.Citation23,Citation24 In this study, we found that a 36–38°C heat stress condition that severely reduces plant fertility interferes with both chromosome dynamics and cytokinesis in Arabidopsis. This report demonstrates that an extreme increase of environmental temperature condition would not only affect fertility but also disturb genomic ploidy consistency of plants by influencing with male meiosis. As in Populus, Citation41 the heat stress induced about 55% meiotic defects, but caused a severely impacted male sterility and seed set in Arabidopsis (), which suggests that high-temperature stress induces male sterility of plants not merely by affecting male meiosis, but also by damaging post-meiosis developmental programs; e.g., tapetum development and microspore maturation.Citation42
Heat stress affects chromosome dynamics in both meiosis Ι and ΙΙ. At diakinesis, PMCs with 10 univalents () suggested that homology synapsis and/or recombination was omitted by the heat. Since the pairing and synapsis of homologous chromosomes depend on proper construction of chromosome axis and synaptonemal complex (SC), the univalents could be induced by heat-interfered chromosome structures.Citation43–Citation46 Examination of the key axis element ASY1 and the SC central element ZYP1 would contribute to the understanding of univalent formation. On the other hand, abnormalities of machineries that catalyze meiotic recombination and/or CO formation also cause univalents.Citation9,Citation46-Citation48 In wheat, high temperature has been shown to induce a significantly increased number of univalent and irregularities in chromosome alignment at metaphase Ι, and DMC1 was proposed to mediate the heat tolerance.Citation49 It is possible that heat stress predominantly interferes with DSB repair and meiotic recombination during male meiosis. To this end, a detection of recombinases DMC1 and RAD51 should be applied in future study. At the same time, we observed a premature separation of sister chromatids prior to anaphase ΙΙ in heat-stressed plants (), which suggests an impacted protection of sister centromeric cohesion under the high-temperature stress. This could be caused by alterations occurring in loading or stability of cohesion factors; e.g., AtREC8, AtSGO1 or other cohesion complex members on the chromosomes. In Arabidopsis, meiotic recombination rate increases under a milder heat stress (28°C), suggesting that a limited elevation of temperature has a positive effect on CO formation.Citation29,Citation32 The fully impaired bivalent formation visualized in our study points to a notion that meiotic recombination machineries of plants can only endure a relatively small range of temperature increase. Monitoring of multiple temperature conditions at a wider range may favor the understanding of how plant meiotic recombination responses to temperature alterations. In Populus and persimmon, similar meiotic defects and unreduced gamete formation were recorded.Citation41,Citation50 This suggests that the response of male meiosis to high-temperature stress may be conserved among plant species.
Our tubulin immunostaining assay and the report by Wang et al. suggested that heat-induced cytokinesis irregularities are caused by omission and/or deconstruction of phragmoplast, or RMAs ().Citation41 It was evidenced that high-temperature stress interferes with microtubular organization independently of affecting protein synthesis.Citation51 It is thus plausible that during meiotic cytokinesis in both Arabidopsis and Populus, the stability of microtubules decreases upon temperature increasing, and the restoration of phragmoplast after incubation under normal temperature is due to recovered tubulin polymerization. The generation of RMAs is mediated by the interaction of microtubule organizing center (MTOC)-originated actin fibers and microtubule, and MTOCs localize at the surface of newly formed nuclei at telophase ΙΙ.Citation52–Citation54 It was proposed that the positioning of separated chromosome sets and the later nucleation determines the spatial sites where RMAs are set.Citation55–Citation57 Therefore, the cytokinesis defects caused by the heat-interfered RMA structuring could be owing to chromosome segregation irregularities and the consequent position of unbalanced and adjacent nuclei. When the formed nuclei get too close to each other, RMAs are not able to form which then causes meiotic restitution (, see yellow arrows). This possibility suggests that the spindle alteration may be the primary effect of heat stress on male meiosis in plants. A real-time monitoring of GFP-tagged tubulin dynamics would contribute to the understanding how tubulin-dependent cytoskeleton during male meiosis responses to temperature increase.Citation58,Citation59
Cold stress can induce alteration in the landscape of male meiotic recombination.Citation24 In addition, cold stress seems to specifically influence meiotic cytokinesis in both Arabidopsis and crops.Citation26–Citation28 It thus is likely that the formation of meiotic cell walls is more sensitive to cold than other meiotic programs, e.g., chromosome condensation and segregation. Moreover, compared with cold stress that does not influence spindle formation, Citation26,Citation27 heat stress impacts multiple meiotic processes, which suggests that the response of male meiosis to low and high temperature is mediated by different signalings. Transcriptome study and examination on the expression of meiosis genes in both cold- and heat-stressed meiosis-staged anthers may contribute to the understanding how male meiosis responses to different temperature alterations.
Material and methods
Plant materials and growth conditions
Arabidopsis (Arabidopsis thaliana) Columbia-0 plants were obtained from the Nottingham Arabidopsis Stock Center. Seeds were germinated in soil for 6–8 d and seedlings were transferred to soil and cultivated in growth chambers with a 16 h day/8 h night, 20°C, and 50% humidity condition.
Heat stress treatment
Young flowering plants were transferred to a humid chamber with a 16 h day/8 h night, 36–38°C stress condition and cultivated for 24 h. For fertility analysis, siliques and anthers were examined after 10 and 5 d of heat treatment, respectively. For tetrad analysis, heat-treated plants were transferred to control condition for 6 h for recovery and tetrad stage flower buds were examined. For immunolocalization experiment, samples were fixed right away upon the finish of heat treatment.
Cytology and immunolocalization
Pollen viability was examined by Alexander staining.Citation60 Tetrad analysis by orcein and aniline blue staining was performed as described previously.Citation61 Buds producing significant numbers of mature meiotic products were used for quantification. FISH experiment was performed by using the protocol reported previously.Citation62 Immunolocalization analysis of ɑ-tubulin was performed as described previously.Citation27 ɑ-tubulin monoclonal antibody (YL1/2) (Invitrogen, MA1-80017) was used with a dilution of 1:200, and goat anti-rat IgG (H + L) secondary antibody conjugated by FITC (Invitrogen, 31629) was diluted by 1:400.
Pollen germination assay
Mature pollen grains from control plants or the 24 h heat-stressed plants with a 5 d recovery at normal temperature conditions, are soiled on pollen growth medium (5 mM CaCl2, 1 mM MgSO4, 5 mM KCl, 0.01 mM H3BO3, 18% Suc, 1.5% agarose, pH at 7.5 modified by 0.1 M NaOH), and the pollen tubes are observed after incubation in a humid chamber under dark for 24 h.
Microscopy
Bright-field images was pictured using M-Shot ML31 microscope equipped with a MS60 camera. Fluorescence microscopy was performed using an Olympus IX83 inverted fluorescence microscope equipped with an X-Cite lamp and a Prime BSI camera. Bifluorescent images and Z-stacks were processed using Image J. Brightness and contrast settings were adjusted using PowerPoint.
Disclosure statement
All authors claim that there is no interest of conflict in this work.
Additional information
Funding
References
- Mercier R, Mézard C, Jenczewski E, Macaisne N, Grelon M. The molecular biology of meiosis in plants. Annu Rev Plant Biol. 2015;66(1):1–10. doi:10.1146/annurev-arplant-050213-035923.
- Bergerat A, de Massy B, Gadelle D, Varoutas P-C, Nicolas A, Forterre P. An atypical topoisomerase II from archaea with implications for meiotic recombination. Nature. 1997;386(6623):414–417. doi:10.1038/386414a0.
- Grelon M, Vezon D, Gendrot G, Pelletier G. AtSPO11-1 is necessary for efficient meiotic recombination in plants. Embo J. 2001;20(3):589–600. doi:10.1093/emboj/20.3.589.
- Hartung F, Wurz-Wildersinn R, Fuchs J, Schubert I, Suer S, Puchta H. The catalytically active tyrosine residues of both SPO11-1 and SPO11-2 are required for meiotic double-strand break induction in Arabidopsis. Plant Cell. 2007;19(10):3090–3099. doi:10.1105/tpc.107.054817.
- Stacey NJ, Kuromori T, Azumi Y, Roberts G, Breuer C, Wada T, Maxwell A, Roberts K, Sugimoto-Shirasu K. Arabidopsis SPO11-2 functions with SPO11-1 in meiotic recombination. Plant J. 2006;48(2):206–216. doi:10.1111/j.1365-313X.2006.02867.x.
- Kobayashi W, Liu E, Ishii H, Matsunaga S, Schlogelhofer P, Kurumizaka H. Homologous pairing activities of Arabidopsis thaliana RAD51 and DMC1. J Biochem. 2019;165(3):289–295. doi:10.1093/jb/mvy105.
- Kurzbauer MT, Uanschou C, Chen D, Schlogelhofer P. The recombinases DMC1 and RAD51 are functionally and spatially separated during meiosis in Arabidopsis. Plant Cell. 2012;24(5):2058–2070. doi:10.1105/tpc.112.098459.
- Li W, Chen C, Markmann-Mulisch U, Timofejeva L, Schmelzer E, Ma H, Reiss B. The Arabidopsis AtRAD51 gene is dispensable for vegetative development but required for meiosis. Proc Natl Acad Sci U S A. 2004;101(29):10596–10601. doi:10.1073/pnas.0404110101.
- Su H, Cheng Z, Huang J, Lin J, Copenhaver GP, Ma H, Wang Y. Arabidopsis RAD51, RAD51C and XRCC3 proteins form a complex and facilitate RAD51 localization on chromosomes for meiotic recombination. PLoS Genet. 2017;13(5):e1006827. doi:10.1371/journal.pgen.1006827.
- Zamariola L, Tiang CL, De Storme N, Pawlowski W, Geelen D. Chromosome segregation in plant meiosis. Front Plant Sci. 2014b;5:279. doi:10.3389/fpls.2014.00279.
- Bai X, Peirson BN, Dong F, Xue C, Makaroff CA. Isolation and characterization of SYN1, a RAD21-like gene essential for meiosis in Arabidopsis. Plant Cell. 1999;11(3):417–430. doi:10.1105/tpc.11.3.417.
- Chelysheva L, Diallo S, Vezon D, Gendrot G, Vrielynck N, Belcram K, Rocques N, Márquez-Lema A, Bhatt AM, Horlow C, et al. AtREC8 and AtSCC3 are essential to the monopolar orientation of the kinetochores during meiosis. J Cell Sci. 2005;118(20):4621–4632. doi:10.1242/jcs.02583.
- Lam WS, Yang X, Makaroff CA. Characterization of Arabidopsis thaliana SMC1 and SMC3: evidence that AtSMC3 may function beyond chromosome cohesion. J Cell Sci. 2005;118(14):3037–3048. doi:10.1242/jcs.02443.
- Yuan G, Ahootapeh BH, Komaki S, Schnittger A, Lillo C, De Storme N, Geelen D. PROTEIN PHOSHATASE 2A B’alpha and beta maintain centromeric sister chromatid cohesion during meiosis in Arabidopsis. Plant Physiol. 2018;178(1):317–328. doi:10.1104/pp.18.00281.
- Zamariola L, De Storme N, Vannerum K, Vandepoele K, Armstrong SJ, Franklin FC, Geelen D. SHUGOSHINs and PATRONUS protect meiotic centromere cohesion in Arabidopsis thaliana. Plant J. 2014a;77(5):782–794. doi:10.1111/tpj.12432.
- Zhang YL, Zhang H, Gao YJ, Yan LL, Yu XY, Yang YH, Xu WY, Pu CX, Sun Y. Protein phosphatase 2A B’alpha and B’beta protect centromeric cohesion during meiosis I. Plant Physiol. 2019;179(4):1556–1568. doi:10.1104/pp.18.01320.
- De Storme N, Geelen D. Cytokinesis in plant male meiosis. Plant Signal Behav. 2013;8(3):e23394. doi:10.4161/psb.23394.
- Hulskamp M, Parekh NS, Grini P, Schneitz K, Zimmermann I, Lolle SJ, Pruitt RE. The STUD gene is required for male-specific cytokinesis after telophase II of meiosis in Arabidopsis thaliana. Dev Biol. 1997;187(1):114–124. doi:10.1006/dbio.1997.8554.
- Spielman M, Preuss D, Li FL, Browne WE, Scott RJ, Dickinson HG. TETRASPORE is required for male meiotic cytokinesis in Arabidopsis thaliana. Development. 1997;124:2645–2657.
- Takahashi Y, Soyano T, Kosetsu K, Sasabe M, Machida Y. HINKEL kinesin, ANP MAPKKKs and MKK6/ANQ MAPKK, which phosphorylates and activates MPK4 MAPK, constitute a pathway that is required for cytokinesis in Arabidopsis thaliana. Plant Cell Physiol. 2010;51(10):1766–1776. doi:10.1093/pcp/pcq135.
- Yang CY, Spielman M, Coles JP, Li Y, Ghelani S, Bourdon V, Brown RC, Lemmon BE, Scott RJ, Dickinson HG. TETRASPORE encodes a kinesin required for male meiotic cytokinesis in Arabidopsis. Plant J. 2003;34(2):229–240. doi:10.1046/j.1365-313X.2003.01713.x.
- Zeng QN, Chen JG, Ellis BE. AtMPK4 is required for male-specific meiotic cytokinesis in Arabidopsis. Plant J. 2011;67(5):895–906. doi:10.1111/j.1365-313X.2011.04642.x.
- De Storme N, Geelen D. The impact of environmental stress on male reproductive development in plants: biological processes and molecular mechanisms. Plant Cell Environ. 2014;37(1):1–18. doi:10.1111/pce.12142.
- Liu B, Mo WJ, Zhang D, De Storme N, Geelen D. Cold influences male reproductive development in plants: a hazard to fertility, but a window for evolution. Plant Cell Physiol. 2019;60(1):7–18. doi:10.1093/pcp/pcy209.
- Morgan CH, Zhang H, Bomblies K. Are the effects of elevated temperature on meiotic recombination and thermotolerance linked via the axis and synaptonemal complex? Philos Trans R Soc Lond B Biol Sci. 2017;372(1736):20160470. doi:10.1098/rstb.2016.0470.
- De Storme N, Copenhaver GP, Geelen D. Production of diploid male gametes in Arabidopsis by cold-induced destabilization of postmeiotic radial microtubule arrays. Plant Physiol. 2012;160(4):1808–1826. doi:10.1104/pp.112.208611.
- Liu B, De Storme N, Geelen D. Cold-induced male meiotic restitution in Arabidopsis thaliana is not mediated by GA-DELLA signaling. Front Plant Sci. 2018;9:91. doi:10.3389/fpls.2018.00091.
- Tang Z, Zhang L, Yang D, Zhao C, Zheng Y. Cold stress contributes to aberrant cytokinesis during male meiosis I in a wheat thermosensitive genic male sterile line. Plant Cell Environ. 2011;34(3):389–405. doi:10.1111/j.1365-3040.2010.02250.x.
- Francis KE, Lam SY, Harrison BD, Bey AL, Berchowitz LE, Copenhaver GP. Pollen tetrad-based visual assay for meiotic recombination in Arabidopsis. Proc Natl Acad Sci U S A. 2007;104(10):3913–3918. doi:10.1073/pnas.0608936104.
- Saini R, Singh AK, Dhanapal S, Saeed TH, Hyde GJ, Baskar R. Brief temperature stress during reproductive stages alters meiotic recombination and somatic mutation rates in the progeny of Arabidopsis. BMC Plant Biol. 2017;17(1):103. doi:10.1186/s12870-017-1051-1.
- Draeger T, Moore G. Short periods of high temperature during meiosis prevent normal meiotic progression and reduce grain number in hexaploid wheat (Triticum aestivum L.). Theor Appl Genet. 2017;130(9):1785–1800. doi:10.1007/s00122-017-2925-1.
- Lloyd A, Morgan CH, Franklin FC, Bomblies K. Plasticity of Meiotic Recombination Rates in Response to Temperature in Arabidopsis. Genetics. 2018;208(4):1409–1420. doi:10.1534/genetics.117.300588.
- Modliszewski JL, Wang H, Albright AR, Lewis SM, Bennett AR, Huang J, Ma H, Wang Y, Copenhaver GP. Elevated temperature increases meiotic crossover frequency via the interfering (Type I) pathway in Arabidopsis thaliana. PLoS Genet. 2018;14(5):e1007384. doi:10.1371/journal.pgen.1007384.
- Pereira HS, Delgado M, Viegas W, Rato JM, Barao A, Caperta AD. Rye (Secale cereale) supernumerary (B) chromosomes associated with heat tolerance during early stages of male sporogenesis. Ann Bot. 2017;119(3):325–337. doi:10.1093/aob/mcw206.
- Wang J, Li D, Shang F, Kang X. High temperature-induced production of unreduced pollen and its cytological effects in Populus. Sci Rep. 2017b;7(1):5281. doi:10.1038/s41598-017-05661-x.
- Jagadish S, Craufurd P, Wheeler T. High temperature stress and spikelet fertility in rice (Oryza sativa L.). J Exp Bot. 2007;58(7):1627–1635. doi:10.1093/jxb/erm003.
- Prasad PVV, Djanaguiraman M, Perumal R, Ciampitti IA. Impact of high temperature stress on floret fertility and individual grain weight of grain sorghum: sensitive stages and thresholds for temperature and duration. Front Plant Sci. 2015;6:820. doi:10.3389/fpls.2015.00820.
- Zhang -S-S, Yang H, Ding L, Song Z-T, Ma H, Chang F, Liu J-X. Tissue-specific transcriptomics reveals an important role of the unfolded protein response in maintaining fertility upon heat stress in Arabidopsis. Plant Cell. 2017;29(5):1007–1023. doi:10.1105/tpc.16.00916.
- De Storme N, Zamariola L, Mau M, Sharbel TF, Geelen D. Volume-based pollen size analysis: an advanced method to assess somatic and gametophytic ploidy in flowering plants. Plant Reprod. 2013;26(2):65–81. doi:10.1007/s00497-012-0209-0.
- d’Erfurth I, Jolivet S, Froger N, Catrice O, Novatchkova M, Simon M, Jenczewski E, Mercier R. Mutations in AtPS1(Arabidopsis thaliana Parallel Spindle 1) lead to the production of diploid pollen grains. PLoS Genet. 2008;4(11): e1000274.
- Wang J, Li D, Shang F, Kang X. High temperature-induced production of unreduced pollen and its cytological effects in populus. Sci Rep. 2017a;7(1):5281.
- Begcy K, Nosenko T, Zhou L-Z, Fragner L, Weckwerth W, Dresselhaus T. Male sterility in maize after transient heat stress during the tetrad stage of pollen development. Plant Physiol. 2019;181(2):683–700. doi:10.1104/pp.19.00707.
- Armstrong SJ, Caryl AP, Jones GH, Franklin FC. Asy1, a protein required for meiotic chromosome synapsis, localizes to axis-associated chromatin in Arabidopsis and Brassica. J Cell Sci. 2002;115((Pt 18)):3645–3655. doi:10.1242/jcs.00048.
- Barakate A, Higgins JD, Vivera S, Stephens J, Perry RM, Ramsay L, Colas I, Oakey H, Waugh R, Franklin FC, et al. The synaptonemal complex protein ZYP1 is required for imposition of meiotic crossovers in barley. Plant Cell. 2014;26(2):729–740. doi:10.1105/tpc.113.121269.
- Higgins JD, Sanchez-Moran E, Armstrong SJ, Jones GH, Franklin FC. The Arabidopsis synaptonemal complex protein ZYP1 is required for chromosome synapsis and normal fidelity of crossing over. Genes Dev. 2005;19(20):2488–2500. doi:10.1101/gad.354705.
- Sanchez-Moran E, Santos JL, Jones GH, Franklin FC. ASY1 mediates AtDMC1-dependent interhomolog recombination during meiosis in Arabidopsis. Genes Dev. 2007;21(17):2220–2233. doi:10.1101/gad.439007.
- Chelysheva L, Vezon D, Chambon A, Gendrot G, Pereira L, Lemhemdi A, Vrielynck N, Le Guin S, Novatchkova M, Grelon M. The Arabidopsis HEI10 is a new ZMM protein related to Zip3. PLoS Genet. 2012;8(7):e1002799–e1002799. doi:10.1371/journal.pgen.1002799.
- Higgins JD, Armstrong SJ, Franklin FCH, Jones GH. The Arabidopsis MutS homolog AtMSH4 functions at an early step in recombination: evidence for two classes of recombination in Arabidopsis. Genes Dev. 2004;18(20):2557–2570. doi:10.1101/gad.317504.
- Draeger T, Martin AC, Alabdullah AK, Pendle A, Rey MD, Shaw P, Moore G. Dmc1 is a candidate for temperature tolerance during wheat meiosis. Theor Appl Genet. 2019;133:809–828. doi:10.1007/s00122-019-03508-9.
- Mai Y, Li H, Suo Y, Fu J, Sun P, Han W, Diao S, Li F. High temperature treatment generates unreduced pollen in persimmon (Diospyros kaki Thunb.). Sci Hortic (Amsterdam). 2019;258:108774. doi:10.1016/j.scienta.2019.108774.
- SMERTENKO A, DRÁBER P, VIKLICKÝ V, OPATRNÝ Z. Heat stress affects the organization of microtubules and cell division in nicotiana tabacum cells. Plant Cell Environ. 1997;20(12):1534–1542. doi:10.1046/j.1365-3040.1997.d01-44.x.
- Brown RC, Lemmon BE, Brown RC, Lemmon BE. Nuclear cytoplasmic domains, microtubules and organelles in microsporocytes of the slipper orchid Cypripedium californicum A. Gray dividing by simultaneous cytokinesis. Sex Plant Reprod. 1996;9(3):145–152. doi:10.1007/BF02221394.
- Shimamura M, Brown RC, Lemmon BE, Akashi T, Mizuno K, Nishihara N, Tomizawa K-I, Yoshimoto K, Deguchi H, Hosoya H, et al. Gamma-tubulin in basal land plants: characterization, localization, and implication in the evolution of acentriolar microtubule organizing centers. Plant Cell. 2004;16(1):45–59. doi:10.1105/tpc.016501.
- TRAAS JA, BURGAIN S, Dumas DE Vaulx R. The organization of the cytoskeleton during meiosis in eggplant (Solanum Melongena (L.)): microtubules and F-actin are both necessary for coordinated meiotic division. J Cell Sci. 1989;92:541–550.
- Brown RC, Lemmon BE. Minispindles and cytoplasmic domains in microsporogenesis of orchids. Protoplasma. 1989;148(1):26–32. doi:10.1007/BF01403988.
- Brown RC, Lemmon BE. Control of division plane in normal and griseofulvin-treated microsporocytes of magnolia. J Cell Sci. 1992;103:1031–1038.
- Brown RC, Lemmon BE. The cytoskeleton and spatial control of cytokinesis in the plant life cycle. Protoplasma. 2001;215(1):35–49. doi:10.1007/BF01280302.
- Goodson HV, Dzurisin JS, Wadsworth P. Methods for expressing and analyzing GFP-tubulin and GFP-microtubule-associated proteins. Cold Spring Harb Protoc. 2010;2010(9):pdb.top85. doi:10.1101/pdb.top85.
- Ueda K, Matsuyama T, Hashimoto T. Visualization of microtubules in living cells of transgenicArabidopsis thaliana. Protoplasma. 1999;206(1):201–206. doi:10.1007/BF01279267.
- Alexander MP. Differential staining of aborted and nonaborted pollen. Stain Technol. 1969;44(3):117–122. doi:10.3109/10520296909063335.
- Liu B, De Storme N, Geelen D. Gibberellin induces diploid pollen formation by interfering with meiotic cytokinesis. Plant Physiol. 2017;173(1):338–353. doi:10.1104/pp.16.00480.
- Wang Y, Cheng Z, Lu P, Timofejeva L, Ma H. Molecular cell biology of male meiotic chromosomes and isolation of male meiocytes in Arabidopsis thaliana. Methods Mol Biol. 2014;1110:217–230.