ABSTRACT
As the main active ingredient of the traditional Chinese medicine Glycyrrhiza uralensis Fisch, liquiritin has multiple biological activities, including anti-inflammatory, antihepatotoxicity, immune regulation, anti-virus and anti-cancer. In addition, liquiritin has been recognized as an allelochemical that displays markedly inhibitory effects on the growth of target plants, G. uralensis and lettuce. However, its phytotoxic mechanism remains unknown. In the present study, the mode of action of liquiritin against root growth of lettuce seedling was researched. After treatments with liquiritin, the cell division in root tips of lettuce seedlings was partly arrested, and the cell viability and root vitality were obviously lost. At the same time, overproduction of reactive oxygen species (ROS), malondialdehyde (MDA) and proline (Pro) in lettuce seedlings were induced by liquiritin. The results indicated that the phytotoxic effects of liquiritin was probably dependent on the induction of ROS overproduction, resulting in membrane lipids peroxidation following with cell death and mitosis process disorder.
1. Introduction
Glycyrrhiza herbs are traditional Chinese medicines that have been widely used in China, Korea and Japan with a long history. They commonly used to treat diseases in the respiratory system, digestive system and immune system, and relieve the toxicity of other drugs.Citation1 Modern medicine researches showed that the medicinal function of Glycyrrhiza herbs was depend on the chemicals in their roots. Up to now, secondary metabolisms isolated from Glycyrrhiza roots include triterpene saponins, flavonoids, coumarin, alkaloids, ammonia acids, volatile substances and polysaccharides. Among them, triterpene saponins and flavonoids are the main active ingredients, which displayed various medicinal functions, such as anti-inflammatory, anti-virus, anti-tumor, anti-ulcer, anti-depression, anti-oxidation, enhance memory, protect nerves and lower cholesterol.Citation2,Citation3
In addition to the medicinal value, Glycyrrhiza herbs are also used in industries such as food, cosmetics, tobacco and animal husbandry.Citation4–Citation6 The usage amount of Glycyrrhiza herbs is so huge, and the wild licorice resources are unable to meet the demand. Therefore, Glycyrrhiza herbs were gradually cultured in many countries.Citation1 For a greater production, continuous cropping mode was used as an effective cultivation. However, Glycyrrhiza herbs were frequently hampered by replanting failure, resulting in reduction in both the yield and medicinal quality.Citation7 Glycyrrhiza uralensis Fisch is one of the most common planted Glycyrrhiza herbs in China. Our previous study indicated that auto-toxicity of the allelochemicals which released by G. uralensis into the rhizosphere soils played an important role in the replanting failure. The main auto-allelochemicals were identified as liquiritin, isoliquiritigenin and glycyrrhizic acid, which displayed strong inhibitory effects on target plants and with relative high concentrations in rhizosphere soils of G. uralensis.Citation8
Liquiritin is one of the main active ingredients of Glycyrrhiza herbs and showed several medicinal activities, including anti-inflammatory, antihepatotoxicity, immune regulation, anti-virus and anti-cancer.Citation9 Because of the great medicinal value, the pharmacodynamic mechanism of liquiritin has been well studied. As an allelochemical, liquiritin effectively inhibited the root and shoot elongation of lettuce and G. uralensis seedlings, showed obviously phytotoxic effects.Citation8 However, the mechanism related to the phytotoxicity of liquiritin has never been reported. In this study, the influences of liquiritin on ROS production, cell division, cell viability, root vitality, malondialdehyde (MDA) and Proline (Pro) contents in the root of lettuce seedlings were investigated. The aim of this study is to claim the physiological mechanisms of the allelopathic activity of liquiritin on lettuce seedlings root growth.
2. Results
2.1. The influence of liquiritin on cell division in root tips of lettuce seedlings
After treatments with liquiritin, the mitosis processes in lettuce root tips were disturbed. The mitotic index (MI) was significantly decreased under concentrations of 20 μM and more (). The inhibitory effects of liquiritin on MI were concentration dependent. At the highest test concentration, the MI was less than half of the control group. The number of cells in each mitotic phase was also recorded, and the results showed that the trend of ratio of each phase was not strictly gone down with the increasing of liquiritin concentrations. However, in general, liquiritin reduced the ratio of each phase at high concentrations ().
2.2. Cell viability in root tips of lettuce seedlings under liquiritin phytotoxicity
Fluorescein diacetate (FDA) and propidium iodide (PI) double staining were used to evaluate the cell viability in root tips of lettuce. As shown in , red fluorescence of PI, which representing dead cells, was induced in the roots by 5 μM liquiritin. With the increasing of liquiritin concentration, the red fluorescence in the root tips was gradually enhanced.
Figure 2. Cell viability in lettuce root tips after treatments with liquiritin. Lettuce seedlings were treated with liquiritin at concentrations of (a) 0, (b) 0.1, (c) 1, (d) 10, (e) 100 and (f) 1000 μM for 48 h. Roots were stained with FDA/PI. Green fluorescence and red fluorescence indicate viable and dead cells in root tips, respectively. Bar = 500 μm.
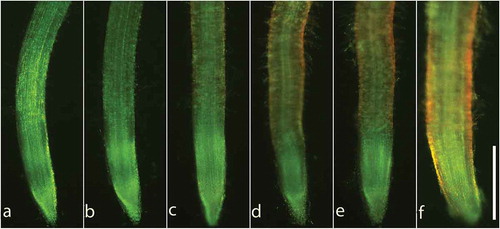
Moreover, the ratios of dead cells in lettuce root were quantified by the determination of Evans blue uptake. Under 1 and 5 μM of liquiritin, the relative uptake of Evans blue by lettuce roots was similar with control. However, at 20 μM and more, the relative uptake of Evans blue was significantly increased ().
2.3. Root vitality of lettuce seedlings after treatments with liquiritin
As shown in , under the concentrations of 1–20 μM, the root vitality of lettuce seedlings treated with liquiritin was similar to that of control. When the concentrations of liquiritin reached 100 μM and higher, the root vitality was significantly decreased.
2.4. ROS production and lipid peroxidation in lettuce seedling roots after treatments with liquiritin
Superoxide radical (OCitation2−), one of the main ROS in plants, was analyzed by staining with dihydroethidium (DHE). Under the lowest test concentration of liquiritin, weak bright fluorescence in the root was detected (). Then, with the increasing of the concentration used, the fluorescence intensity in treated roots was gradually enhanced with very strong fluorescence at high concentrations of 100 and 400 μM ().
Figure 4. ROS production in lettuce roots after treatments with liquiritin. Lettuce seedlings were treated with liquiritin at concentrations of (a) 0, (b) 0.1, (c) 1, (d) 10, (e) 100 and (f) 1000 μM for 48 h. Roots were stained with DHE. Bright fluorescence shows ROS production (presumably O2−). Bar = 200 μm.
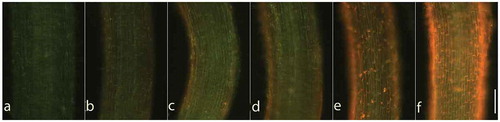
Hydrogen peroxide (H2O2), another main ROS in plants, was quantitated to evaluate the ROS level. As shown in , at 1 and 5 μM, liquiritin has no significant effects on the content of H2O2 in lettuce roots. However, under concentration of 20 μM and more, the contents of H2O2 were rapidly increased by liquiritin stress.
Figure 5. H2O2 and MDA contents in roots of lettuce seedlings after treatments with liquiritin. The results presented are mean of three replicates ± SE, different letters denote significant differences at p < .05 according to oneway ANOVA with an LSD test.
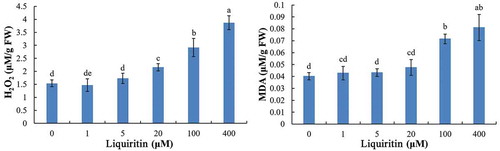
Lipid peroxidation was measured by detecting the content of MDA, an end product of lipid peroxidation. Under low concentrations of 1 and 5 μM, the MDA level induced by liquiritin was similar with the control. At 20 μM, the content of MDA was slightly increased. At high concentrations of 100 and 400 μM, the MDA level was significantly increased, indicating strong lipid peroxide formation in lettuce roots ().
2.5. Effect of liquiritin on proline accumulation levels in lettuce seedling roots
Free proline, which generally accumulates in plant tissues under stress, was determined to evaluate the stress strength of liquiritin. Free proline levels in lettuce roots at 1 and 10 μM of liquiritin were similar to control. However, free proline content was markedly increased at 20 μM and more ().
3. Discussion
Crude extracts of G. uralensis tissues showed significant inhibitory effects on the seed germination and seedling growth of some plant species, indicating that G. uralensis has allelopathy potential.Citation10–Citation13 In our previous study, the secondary metabolites in rhizosphere soils of G. uralensis were isolated and identified. Through bioassay and content detection, the main allelochemicals released by G. uralensis were characterized as liquiritin, isoliquiritigenin and glycyrrhizic acid. Liquiritin is a flavone compound and strongly inhibited the root and shoot elongation of lettuce seedlings in a concentration-dependent manner, displaying a typical phytotoxicity feature.Citation8 In addition, liquiritin is also one of the main active ingredients of Glycyrrhiza herbs, exhibiting various pharmacological activities, such as anti-tumor, anti-virus and antidepressant.Citation9
Liquiritin could suppress the migration and proliferation, and induce apoptosis and ROS generation in human gastric cancer cell lines.Citation14 Moreover, liquiritin strongly decreased viability and induced apoptosis, as well as promoted the accumulation of ROS in HepG2 cells.Citation15 These results suggested that the anti-cancer effects of liquiritin was involved in the generation of ROS.
ROS is a class of oxygen-containing groups with high biological activity. In higher plants, several types of ROS, such as H2O2,Citation2− hydroxyl radical (-OH) and hydroxide ion (OH−), are produced as byproducts of the normal metabolism of oxygen mainly in chloroplast, peroxide body and mitochondria.Citation16,Citation17 As a signal molecular, ROS play important roles in mediating plant growth and development in response to biological and abiotic stresses, protein modification, and gene expression regulation.Citation18 However, excessive ROS with high concentrations is very harmful to plant cells, will result in oxidative damage to proteins, DNA and lipids, and induce programmed cell death (PCD).Citation19
In this study, the production of two main ROS, O2− and H2O2 in lettuce roots after treatments with liquiritin was determined. The O2− was intuitively estimated by staining with DHE, a specific dye reacts in proportion to the intracellular level of O2−.Citation20 Florescence of DHE in root tips of lettuce seedlings was remarkably enhanced after treatments, indicating that liquiritin could efficiently induce the production and accumulation of O2− in the target plant. The level of H2O2 was quantitatively determined, and the result showed that the content of H2O2 in lettuce roots was increased after treatments, suggesting that liquiritin induces the overproduction of H2O2 in lettuce. These results confirmed that liquiritin could induce the accumulation of ROS in plant cells, which is consistent with the results of ROS overproduction in cancer cells exogenous application with liquiritin.Citation14,Citation15
Oxidative stress produced by excess ROS will cause lipid peroxidation in the cell membranes.Citation19 MDA, a highly reactive compound that exists as the enol, results from lipid peroxidation of polyunsaturated fatty acids. MDA is usually considered as a marker for oxidative stress.Citation21 In the present study, the level of MDA in lettuce roots increased under liquiritin treatments, indicating that the phytotoxicity of liquiritin involved in lipid peroxidation and damage of membranes. Alternatively, liquiritin could alleviate the oxidative stress induced by other medicals in animals.Citation22
The lipid peroxidation and the breakage of lipids will change the permeability and fluidity of the membrane lipid bilayer, resulted in dramatic alternation of cell integrity and even cell death.Citation23 In this study, the cell integrity was determined by three methods, including doubly staining with FDA and PI, detecting the uptake of Evans blue and 2,3,5-Triphenyltetrazolium chloride (TTC) reduction. All the results showed that the cell viability in root tips of lettuce was decreased and the number of death cells was increased. Together with the results of ROS and MDA, we proposed that liquiritin could induce ROS overproduction in lettuce roots, and then the excessive ROS caused membrane disruption and loss of cell viability, as well as cell death. Therefore, ROS might play a central role in the phytotoxic effect of liquiritin.
As an anti-cancer agent, liquiritin displayed strong anti-proliferation activities on several cancer lines, and effectively arrest cell division at G0/G1 or G2/M transition.Citation14,Citation24,Citation25 In this study, liquiritin treatments also influenced the root tip cell division. The MI and the numbers of cells in all mitotic phases were gradually decreased with the increasing of liquiritin concentration, indicating that liquiritin might interfere with a certain phase of cell cycle and prevent cells from interphase entering into mitosis in target plant. Thus, the phytotoxic effects of liquiritin on lettuce root growth were at least partly dependent on the arrest of mitosis. Oxidative stress is thought to affect cell cycle progression in plants.Citation26,Citation27 Therefore, the liquiritin-induced inhibition of cell division was likely involved in ROS overproduction.
Once under the environmental stresses, free proline will rapidly accumulate in plants. As a signaling molecule, proline conducts multifunctions, including osmotic adjustment, detoxification of ROS and protection of membrane integrity.Citation28 So proline is essential for the recovery of plant from stress. Previous study showed that proline accumulation was associated with ROS production and oxidative stress under environmental stresses in plants.Citation29,Citation30 In this study, a rise of proline level and accumulation of ROS appeared in lettuce roots under liquiritin-treatments, suggesting that liquiritin induces strong anti-stress response of lettuce, and ROS might be involved in the accumulation of proline to regulate liquiritin-induced stress.
Recent studies revealed that the generated ROS in receptor plants was involved in the phytotoxicity of several allelochemicals.Citation31–Citation36 The results highlighted the essential role of ROS in allelochemical-induced stress in plants. In addition, allelochemicals also have the ability to arrest mitosis in plant cells. They can arrest cells in all of the mitotic phases or sometimes only in the metaphase.Citation31–Citation33,Citation37 So the influence of mitosis of allelochemicals was also an important factor for the growth inhibition of plants. However, more studies will be needed to clarify the molecular target and the signal transportation pathway of a certain allelochemical.
4. Materials and methods
4.1. Plant culture
Lettuce seeds were purchased from Henan Fengyuan Seed Company. Petri dish (Φ = 9 cm) containing 2 pieces of filter paper (Qualitative, Whatman-Xinhua, Hangzhou, China) was soaked with 2 mL distilled H2O. Then, the seeds were transferred to the Petri dish and germinated at 25 ± 1°C in a plant growth chamber. After 24 h, the germinated seeds were used for treatments.
4.2. Liquiritin treatments
Liquiritin (≥98%, Aladdin-reagent Inc., Shanghai, China) was dissolved in DMSO (Solarbio, Beijing, China) with a concentration of 200 mM, and then serially diluted to 50, 10, 2.5 and 0.5 mM, respectively, as stock solutions. In all experiments, each well of a 6-well plate (NUNC, Shanghai, China) was supplied with 2 μL stock solution and 998 μL distilled H2O, to make the final treated concentrations as 400, 100, 20, 5 and 1 μM, respectively. The same ratio (2‰, v/v) of the solvent DMSO was used as control. For each treated group, five germinated lettuce seeds with similar size were transferred into each well with six wells. The plates were then incubated at 25 ± 1°C in a plant growth chamber. After 48 h, roots of the seedlings were used for testing.
4.3. Mitotic index (MI)
The roots of treated lettuce seedlings were cut off and immediately transferred to Carnoy’s fluid (ethanol/acetic acid, 3/1, v/v) at 4°C for 24 h. Then, the fixed roots were hydrolyzed using 5% HCl for 5 min, following with Schiff’s reagent (Leagene, Beijing, China) staining for 30 min. Root tips (about 2 mm) were excised and gently squashed, and then observed by a digital microscope (Yetech, Beijing, China). At least 800 cells were analyzed in each treatment. The ratio between the number of cells during mitosis and the number of total observed cells was calculated as the MI. The proportions of cells in each division phase including prophase, metaphase, anaphase and telophase were also analyzed.
4.4. Cell viability
The cell viability in lettuce root tips was evaluated by double staining with FDA and PI according to Pan et al.Citation38 with minor modification. After treatments, root segments were collected and stained in a mixture of FDA (12.5 μg/mL, MP Biomedicals, CA, USA) and PI (5.0 μg/mL, MP Biomedicals, CA, USA) for 10 min. The stained roots were washed with distilled H2O to remove excess dye in the surface of the roots, and then observed by a fluorescence microscope (Leica DMI4000B, Wetzlar, Germany) with excitation 488 nm and emission > 510 nm. At least 15 roots of each treatment were analyzed and the representative results were shown in the figures.
Root cell viability was also quantitatively evaluated by detecting the relative uptake of Evans blue.Citation39 Root segments of the treated lettuce seedlings were soaked in 0.25% (w/v) Evans blue (Solarbio, Beijing, China) for 1 h at 25°C. The stained roots were washed with distilled H2O, and then soaked in 1 mL N,N-dimethylformamide for 24 h at 25°C in darkness to extract the Evans blue that had been absorbed into the lettuce roots. Absorbance of the released Evans blue was measured at 600 nm by a spectrophotometer (UV-1750, Shimadzu Corp., Kyoto, Japan). The relative Evans blue uptake was calculated as the ratio between the OD values of the treated group and the control.
4.5. Root vitality
Root vitality of lettuce seedlings was evaluated by the method in literature.Citation40 About 200 mg root sample was collected and put into a tube containing 5 mL of phosphate buffer (pH, 6.9). Then, 5 mL of 0.4% TTC solution was added to the tube and place in a 37°C water bath for 90 min. Sulfuric acid (2 mL) of 1 M was added to stop the reaction, and 10 mL of ethyl acetate was used to extract red triphenylbenzidine (TTF). The absorbance of the solution was determined spectrophotometrically at 485 nm. The root vitality was estimated by comparing with standard graph and expressed in µg/g/h.
4.6. ROS production
ROS production in root tips of the treated lettuce seedlings was estimated by staining with DHE (Solarbio, Beijing, China) according to Yamamoto et al.Citation41 Lettuce roots were soaked in the dye solution (DHE, 10 mM; acetone, 0.01%; CaCl2, 100 mM; pH 4.75) with gentle shake for 10 min at 25°C in the dark. After washing with 100 mM CaCl2 for 20 min, the fluorescence was observed and photographed using a fluorescence microscope (Leica DMI4000B, Wetzlar, Germany) with excitation 488 nm and emission > 510 nm. At least 15 roots per treatment were analyzed and the representative results were shown in the figures.
4.7. Determination of H2O2
Endogenous H2O2 in roots of lettuce seedlings was estimated according to Frew et al.Citation42 About 100 mg lettuce roots were homogenized in 0.1 M phosphate buffer (pH 6.9), and then centrifuged at 8000 g, 4°C for 10 min (3k15, Sigma, Niedersachsen, Germany). The supernatant (4 mL) was taken and mixed with 0.3 mL reagent solution (phenol, 2.34 g/L; 4-aminoantipyrine, 1 g/L; phosphate buffer, 1 mM, pH 6.9) to determine the absorbance at 505 nm spectrophotometrically. The content of H2O2 in lettuce roots is compared with standard graph and expressed in µM/g FW.
4.8. Lipid peroxidation
The content of MDA was measured to evaluate the lipid peroxidation.Citation43 Lettuce roots (about 100 mg) were homogenized in 5 mL trichloroacetic acid (TCA, 10%, w/v), and then centrifuged at 4,000 g for 10 min. The supernatant (1.0 mL) was added to 2.0 mL of thiobarbituric acid (TBA, 0.6%, w/v) in 10% TCA. The tube containing the mixture was incubated in boiling water for 15 min, following with ice bath for 10 min. After centrifugation at 9,000 g for 5 min, the absorbance of the supernatant at 440, 532 and 600 nm was measured, and the levels of MDA were calculated in the following manner:
[MDA] = [6.452 (A532 – A600) – 0.56 A450] ·VT/(V·W)
[MDA] represents the concentration of MDA expressed in µM g−1. A450, A532 and A600 are the absorbance values at 450 nm, 532 nm and 600 nm, respectively. VT and V represent total volume of the extracting solution and the volume used in measurement, respectively. W is the fresh weight of the lettuce roots used.
4.9. Free proline
Free proline was determined according to the method of Bates et al.Citation44 About 100 mg lettuce roots were homogenized in 3 mL sulfosalicylic acid (3%, w/v) and then centrifuged at 2,000 g for 10 min. The supernatant (1.0 mL) was mixed with acid ninhydrin and glacial acetic acid at a ratio of 1:1:1, and incubated in boiling water for 1 h, and then in an ice bath for 10 min. Toluene (4.0 mL) was added to the mixture, and after the organic and inorganic phases were separated, the organic phase was monitored at 520 nm spectrophotometrically. Proline content was read from a calibration line constructed with pure proline (Alfa Aesar, Shanghai, China) standards.
4.10. Statistical analysis
All experiments list above were repeated at least three times and the results are represented as the means ± standard error (SE). SPSS 16.0 (SPSS Inc., Chicago, USA) was used for data statistical analysis. The differences between control and liquiritin-treated samples were determined using one-way ANOVA with an LSD test.
5. Conclusion
Liquiritin showed significant inhibitory effects on lettuce growth. In the cells, it induced ROS overproduction followed by lipid peroxidation and reduction of root vitality and cell death. Simultaneously, the mitotic phases and the contents of proline in root tip cells were affected by the liquiritin application. In brief, ROS probably plays a key role in the phytotoxic effects of liquiritin.
Author contributions
ZQY and LGY designed the research. ZQY and KG performed most of the experiments. JT conducted the data analysis. ZQY and LGY wrote the manuscript. All the authors contributed to improving the paper and approved the final manuscript.
Disclosure of Potential Conflicts of Interest
The authors declare no conflict of interest.
Additional information
Funding
References
- Damle M. Glycyrrhiza glabra (liquorice)-A potent medicinal herb. Int J Herb Med. 2014;2:1–6.
- Wang L, Yang R, Yuan B, Liu Y, Liu C. The antiviral and antimicrobial activities of licorice, a widely-used Chinese herb. Acta Pharm Sin B. 2015;5:310–315. doi:10.1016/j.apsb.2015.05.005.
- Hosseinzadeh H, Nassiri-Asl M. Pharmacological effects of Glycyrrhiza spp. and its bioactive constituents: update and review. Phytother Res. 2015;29:1868–1886. doi:10.1002/ptr.5487.
- Hayashi H, Sudo H. Economic importance of licorice. Plant Biotechnol. 2009;26:101–104. doi:10.5511/plantbiotechnology.26.101.
- Ishimi Y, Takebayashi J, Tousen Y, Yamauchi J, Fuchino H, Kawano T, Inui T, Yoshimatsu K, Kawahara N. Quality evaluation of health foods containing licorice in the Japanese market. Toxicol Rep. 2019;6:904–913. doi:10.1016/j.toxrep.2019.08.013.
- Alagawany M, Elnesr SS, Farag MR, Abd El-Hack ME, Khafaga AF, Taha AE, Tiwari R, Yatoo MI, Bhatt P, Marappan G, et al. Use of licorice (Glycyrrhiza glabra) herb as a feed additive in poultry: current knowledge and prospects. Animals. 2019;9:536. doi:10.3390/ani9080536.
- Zhang ZY, Lin WX. Continuous cropping obstacle and allelopathic autotoxicity of medicinal plants. Chin J Eco-Agric. 2009;17:189–196. doi:10.3724/SP.J.1011.2009.00189.
- Ren X, Yan ZQ, He XF, Li XZ, Qin B. Allelochemicals from rhizosphere soils of Glycyrrhiza uralensis fisch: discovery of the autotoxic compounds of a traditional herbal medicine. Ind Crop Prod. 2017;97:302–307. doi:10.1016/j.indcrop.2016.12.035.
- Mamedov NA, Egamberdieva D. Phytochemical constituents and pharmacological effects of licorice: A review. Plant Hum Health. 2019;3:1–21.
- Navaey HN, Tilebeni HG, Ghaderi M, Sanei M. Allelopathic effect of water extract of liquorice (Glycyrrhiza glabra) on germination and chlorophyll content of maize. J Nov Appl Sci. 2013;2:1220–1223.
- Liu F, Teng F, Zhang WD, Li L. Allelopathy of Glycyrrhiza pallidiflora maxim and its impact on seed germination and seedling growth of crops. Hubei Agric Sci. 2009;4:904–905.
- Zhang DD, Liang XH, Wang J. Effect of aqueous extracts from Glycyrrhiza uralensis Fisch seeds on its seed germination and genes relative expression of GuSQS1 and GubAS . J Nucl Ag Ric Sci. 2016;30:28–34.
- Zhao P, Wang W, Zhao Q. Study on allelopathic effects of five Chinese herbal medicines on Astragalus membranaceus. North Horticul. 2013;22:160–163.
- Xie R, Gao CC, Yang XZ, Wu SN, Wang HG, Zhang JL, Yan W, Ma T. Combining TRAIL and liquiritin exerts synergistic effects against human gastric cancer cells and xenograft in nude mice through potentiating apoptosis and ROS generation. Biomed Pharmacother. 2017;93:948–960. doi:10.1016/j.biopha.2017.06.095.
- Wang JR, Li TZ, Wang C, Li SM, Luo YH, Piao XJ, Feng YC, Zhang Y, Xu WT, Zhang Y, et al. Liquiritin inhibits proliferation and induces apoptosis in HepG2 hepatocellular carcinoma cells via the ROS-mediated MAPK/AKT/NF-κB signaling pathway. N -S Arch Pharmacol. 2020. doi:10.1007/s00210-019-01763-7.
- Huang H, Ullah F, Zhou DX, Yi M, Zhao Y. Mechanisms of ROS regulation of plant development and stress responses. Front Plant Sci. 2019;10:800. doi:10.3389/fpls.2019.00800.
- Mhamdi A, Van Breusegem F. Reactive oxygen species in plant development. Development. 2018;145:dev164376. doi:10.1242/dev.164376.
- Waszczak C, Carmody M, Kangasjärvi J. Reactive oxygen species in plant signaling. Annu Rev Plant Biol. 2018;69:209–236. doi:10.1146/annurev-arplant-042817-040322.
- Choudhary A, Kumar A, Kaur N. ROS and oxidative burst: roots in plant development. Plant Divers. 2020;42:33–43. doi:10.1016/j.pld.2019.10.002.
- Garnczarska M. Response of the ascorbate-glutathione cycle to re-aeration following hypoxia in lupine roots. Plant Physiol Biochem. 2005;43:583–590. doi:10.1016/j.plaphy.2005.05.003.
- Alché JD. A concise appraisal of lipid oxidation and lipoxidation in higher plants. Redox Biol. 2019;23:101136. doi:10.1016/j.redox.2019.101136.
- Huang Z, Sheng Y, Chen M, Hao Z, Hu F, Ji L. Liquiritigenin and liquiritin alleviated MCT-induced HSOS by activating Nrf2 antioxidative defense system. Toxicol Appl Pharmacol. 2018;355:18–27. doi:10.1016/j.taap.2018.06.014.
- Gaschler MM, Stockwell BR. Lipid peroxidation in cell death. Biochem Biophys Res Commun. 2017;482:419–425. doi:10.1016/j.bbrc.2016.10.086.
- Wei F, Jiang X, Gao H, Gao S. Liquiritin induces apoptosis and autophagy in cisplatin (DDP)-resistant gastric cancer cells in vitro and xenograft nude mice in vivo. Int J Oncol. 2017;51:1383–1394. doi:10.3892/ijo.2017.4134.
- Li X, Cai L, Liu J, Ma Y, Kong Y, Li H, Jiang M. Liquiritin suppresses UVB-induced skin injury through prevention of inflammation, oxidative stress and apoptosis through the TLR4/MyD88/NF-κB and MAPK/caspase signaling pathways. Int J Mol Med. 2018;42:1445–1459. doi:10.3892/ijmm.2018.3720.
- Reichheld JP, Vernoux T, Lardon F, Montagu MV, Inzé D. Specific checkpoints regulate plant cell cycle progression in response to oxidative stress. Plant J. 1999;17:647–656. doi:10.1046/j.1365-313X.1999.00413.x.
- Livanos P, Galatis B, Quader H, Apostolakos P. Disturbance of reactive oxygen species homeostasis induces atypical tubulin polymer formation and affects mitosis in root-tip cells of Triticum turgidum and Arabidopsis thaliana. Cytoskeleton. 2012;69:1–21. doi:10.1002/cm.20538.
- Kaur G, Asthir B. Proline: A key player in plant abiotic stress tolerance. Biol Plant. 2015;59:609–619. doi:10.1007/s10535-015-0549-3.
- Ben Rejeb K, Abdelly C, Savouré A. How reactive oxygen species and proline face stress together. Plant Physiol Biochem. 2014;80:278–284. doi:10.1016/j.plaphy.2014.04.007.
- Zhang L, Becker DF. Connecting proline metabolism and signaling pathways in plant senescence. Front Plant Sci. 2015;6:552. doi:10.3389/fpls.2015.00552.
- Yan ZQ, Wang DD, Cui HY, Zhang DH, Sun YH, Jin H, Li X, Yang X, Guo H, He X, et al. Phytotoxicity mechanisms of two coumarin allelochemicals from Stellera chamaejasme in lettuce seedlings. Acta Physiol Plant. 2016;38:248–258. doi:10.1007/s11738-016-2270-z.
- Yan ZQ, Wang DD, Ding L, Cui HY, Jin H, Yang XY, Yang JS, Qin B. Mechanism of artemisinin phytotoxicity action: induction of reactive oxygen species and cell death in lettuce seedlings. Plant Physiol Biochem. 2015;88:53–59. doi:10.1016/j.plaphy.2015.01.010.
- Zhang S, Sun SW, Shi HL, Zhao K, Wang J, Liu Y, Liu XH, Wang W. Physiological and biochemical mechanisms mediated by allelochemical isoliquiritigenin on the growth of lettuce seedlings. Plants. 2020;9:245. doi:10.3390/plants9020245.
- AlQuraidi AO, Mosa KA, Ramamoorthy K. Phytotoxic and genotoxic effects of copper nanoparticles in coriander (Coriandrum sativum-Apiaceae). Plants. 2019;8:19. doi:10.3390/plants8010019.
- Zhang W, Lu LY, Hu LY, Cao W, Sun K, Sun QB, Siddikee A, Shi RH, Dai CC. Evidence for the involvement of auxin, ethylene and ROS signaling during primary root inhibition of Arabidopsis by the allelochemical benzoic acid. Plant Cell Physiol. 2018;59:1889–1904. doi:10.1093/pcp/pcy107.
- Huang C, Xu L, Sun J, Zhang Z, Fu M, Teng H, Yi K. Allelochemical p-hydroxybenzoic acid inhibits root growth via regulating ROS accumulation in cucumber (Cucumis sativus L.). J Integr Agr. 2020;19:518–527. doi:10.1016/S2095-3119(19)62781-4.
- Cheng F, Cheng Z, Meng H, Tang X. The garlic allelochemical diallyl disulfide affects tomato root growth by influencing cell division, phytohormone balance and expansin gene expression. Front Plant Sci. 2016;7:1199. doi:10.3389/fpls.2016.01199.
- Pan J, Zhu M, Chen H. Aluminum-induced cell death in root-tip cells of barley. Environ Exp Bot. 2001;46:71–79. doi:10.1016/S0098-8472(01)00083-1.
- Tamás L, Šimonovicová M, Huttová J, Mistrík I. Aluminium stimulated hydrogen peroxide production of germinating barley seeds. Environ Exp Bot. 2004;51:281–288. doi:10.1016/j.envexpbot.2003.11.007.
- Chen TH, Gusta LV. Abscisic acid-induced freezing resistance in cultured plant cells. Plant Physiol. 1983;73:71–75. doi:10.1104/pp.73.1.71.
- Yamamoto Y, Kobayashi Y, Devi SR, Rikiishi S, Matsumoto H. Aluminum toxicity is associated with mitochondrial dysfunction and the production of reactive oxygen species in plant cells. Plant Physiol. 2002;128:63–72. doi:10.1104/pp.010417.
- Frew JE, Jones P, Scholes G. Spectrophotometric determination of hydrogen peroxide and organic hydropheroxides at low concentrations in aqueous solution. Anal Chim Acta. 1983;155:139–150. doi:10.1016/S0003-2670(00)85587-7.
- Hodges DM, DeLong JM, Forney CF, Prange RK. Improving the thiobarbituric acid-reactive-substances assay for estimating lipid peroxidation in plant tissues containing anthocyanin and other interfering compounds. Planta. 1999;207:604–611. doi:10.1007/s004250050524.
- Bates LS, Waldren RP, Teare ID. Rapid determination of free prolin for water-stress studies. Plant Soil. 1973;39:205–207. doi:10.1007/BF00018060.