ABSTRACT
Acute and chronic arsenic (As) toxicity is a global health issue affecting millions of people, which leads to inactivation of over 200 enzymes, particularly those involved in cellular energy pathways and DNA synthesis and repair. The fern Pteris vittata acts as a hyperaccumulator of As and may be useful for phytoremediation to reduce disposal risks by utilizing metal-enriched plant biomass in energy and metal recovery. However, these ferns grow in limited environments and its transplantation and transport can be challenging. Therefore, we generated a transgenic Arabidopsis plant as a seed plant model, capable of accumulating As in their vacuole lumen. This was achieved by transforming the As-resistant bacterial As transporter, ArsB, via fusion with a organelle-targeting signal to the vacuolar membrane, N-ethyl-maleimide-sensitive factor attachment protein receptors (SNAREs) protein, VAMP711. In this study, we developed the iVenus assay as a method for detecting whether the N- or C-terminus of a membrane protein is located on the cytoplasmic or exoplasmic side, and from the result of the iVenus assay, we generated the transgenic plant introduced N-terminal end of ArsB with VAMP711, localized to the central vacuolar membrane to accumulate As in the shoot and differentiation zone of root.
KEYWORDS:
Introduction
Highly toxic arsenic (As) has two oxidation states in nature: arsenate, As(V), and the more toxic arsenite [As(III)]. Since As(V) is structurally analogous to inorganic phosphate, it readily enters the cell via the phosphate membrane transport system, thereby impairing metabolic reactionsCitation1. Alternatively, As(III) enters the cell via aquaglyceroporins and membrane channels that transport water and small neutral molecules,Citation2,Citation3 and exerts its toxicity by binding to thiol groups on various proteins.Citation4,Citation5 Across the world, As contaminates soil and groundwater, entering the food chain through contaminated drinking water and vegetables.Citation6 Thus, As contamination is a major health hazard, and reducing its accumulation in groundwater and cultivation soils is critical for human health.
Since As is highly toxic to plants, various plants have developed metabolic systems for its detoxification. After As enters the plant root cells via aquaglyceroporins or phosphate transporters in the form of As(III) or As(V), respectively, the latter is reduced to As(III), which is then detoxified through various pathways. In angiosperms, As becomes detoxified in the cell primarily by being sequestered in the vacuole in the form of phytochelatins (PCs)-As conjugates. AtABCC1 and AtABCC2, members of the ABC transporter superfamily, localize to the vacuolar membrane and transport PCs-As to protect cellular components from toxic As.Citation7 Meanwhile, ferns employ an alternate mechanisms for sequestering As into the vacuole. For instance, in the Chinese brake fern (Pteris vittata), identified as the first As-hyperaccumulating plant, As(III) is translocated to the fronds without synthesizing PC-As conjugates, and becomes stored in vacuoles via the vacuole membrane protein PvACR3 (Arsenic Compounds Resistance 3).Citation8
One strategy for phytoremediation is to remove hazardous materials, such as heavy metals or metalloids, from polluted soil and water by using plants that hyperaccumulate heavy metals and eliminate As, such as the fern, P. vittata.Citation9 However, this fern exhibits an extremely restricted geographic and ecological distribution to warm, damp areas, such as tropical rainforests, while avoiding dry, cold areas. Hence, alternative phytoremediation technologies are needed to eliminate As from contaminated soils in various environments. Previous studies reported that transgenic Arabidopsis plants expressing As(III)-exporter ACR3 from yeast (ACR3), or P. vittata (PvACR3), were capable of tolerating As, however, did not cause its hyperaccumulation.Citation10,Citation11 Meanwhile, transgenic plants expressing PvACR3;1 increased accumulation of As by 19% in the root, but not in the shoot.Citation12,Citation13 However, generation of transgenic plants for phytoremediation requires advancement to ensure that As is readily accumulated within the shoots.
Certain bacteria possess a plasmid-determined bacterial resistance system for As and antimony. Specifically, in Escherichia coliCitation14 this system consisting of five genes (arsR, arsD, arsA, arsB, and arsC),Citation15–19 and three genes in Staphylococcus species (arsR, arsB, and arsC).Citation15,Citation20 The well-characterized ArsB functions alone as an As(III) efflux pump using energy supplied by the membrane potential of the cellCitation16,Citation17 in bacteria, such as E. coli and Staphylococcus species. Additionally, ArsB associated with the ATPase ArsA, pumps out As(III) in an ATP-dependent process.Citation18
We have previously demonstrated that the Arabidopsis N-ethyl-maleimide-sensitive factor attachment protein receptors (SNAREs), AtVAM3 (SYP22), and SYP121, can be used as organelle-targeting signals to direct the bacterial heavy metal transporter MerC to the vacuolar membrane or plasma membrane in both yeast and Arabidopsis. Moreover, the plants exhibited cadmium and mercury tolerance, as well as an increased accumulation capacity.Citation19
In the current study we, therefore, aimed to generate a plant with a new vacuolar membrane transporter, other than the ABCC1/2 transporter, to sequester free-As(III) in seed plants. Thus, we fused the vacuolar membrane R-SNARE protein, VAMP711, or GFP to the N-terminal end of ArsB to develop Arabidopsis plants expressing VAMP711-ArsB- GFP (VBG). We observed VBG localization to the central vacuoles and some accumulation of As, without As tolerance in VBG-expressing plants.
Results
ArsB lacking an organelle-targeting sequence localizes to SNX1-endosomes
Although the bacterial arsenic transporter ArsB has been shown to localize to the plasma membrane in bacteria and transport As(III) out of the cell, the specific localization of ArsB in ArsB-expressing plant cells is unclear.Citation21 Therefore, to determine the subcellular localization of GFP-ArsB in plant cells, we transiently expressed GFP-ArsB under control of the Cauliflower mosaic virus 35S promoter in various mRFP-tagged organelle marker lines. We found that GFP-ArsB predominantly co-localized with the late endosome marker SNX1-mRFP, and not with the Golgi marker ST-mRFP, trans-Golgi marker mRFP-VHAa1, or vacuolar membrane marker mRFP-AtVam3, indicating that transiently expressed GFP-ArsB was localized in late endosomes in Arabidopsis cells ().
Figure 1. ArsB localizes to the endosome, and N- and C-terminal ends of ArsB are exoplasmic
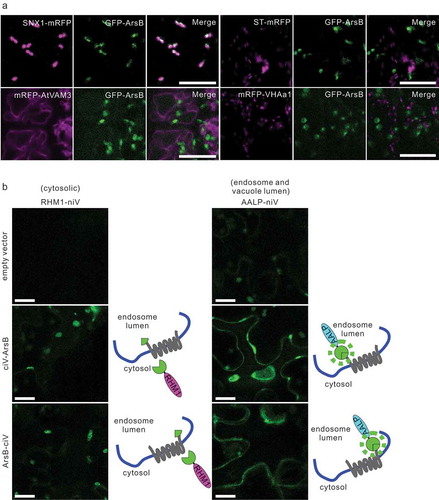
The N- and C-terminal ends of ArsB are oriented toward the vacuole lumen
To accumulate As in the vacuolar lumen, we next aimed to localize ArsB to the vacuolar membrane by applying organelle-targeting technology as reported in our previous study.Citation19 Localization to a targeted organelle requires the attachment of a SNARE-localization tag to the cytoplasmic side of the N- or C-terminus of the protein. However, the orientation of the N- and C-terminal ends of ArsB protein was unknown, although both ends should have the same orientation in the cytoplasm and exoplasm since ArsB is a lipophilic protein with 12 transmembrane helices that are crucial for the transport of As(III) or antimonite across the PM, according to the previous reportCitation22 and our topology predictions in silico. Therefore, we developed a new assay using the split-Venus, designated auto-interactive Venus (iVenus assay), based on an earlier report describing self-assembly between the N- and C-terminal fragments of Venus,Citation23 which can be used to examine the orientation of both N- or C-terminal ends of a transmembrane protein. First, we prepared plasmids of the iVenus marker set; the C-terminals of cytosolic protein rhamnose biosynthesis 1 (RHM1) or the vacuole lumen protein aleurain-like protease (AALP) were fused with the N-terminal fragment (M1–I189) of Venus to express RHM1-niV or AALP-niV, which ensured its localization to the cytoplasm, or the vacuole lumen in N. benthamiana (Fig. S1). Next, we attached the C-terminal fragment (E116–K239) of Venus (ciV), carrying the point mutation A207K, to the N- or C-terminus ends of ArsB to express the ciV-ArsB or ArsB-ciV proteins, respectively. These fragments were then subcloned into the plant expression vector pGWB402. To visualize the interaction between ciV-ArsB or ArsB-ciV and two markers in living cells with the Agrobacterium-infiltrated leaves of N. benthamiana, the markers and ciV-ArsB or ArsB-ciV were transiently co-expressed. As a result, both the ciV-ArsB and ArsB-ciV proteins fluoresced when combined with the AALP-niV proteins, but not with RHM1-niV (); however, the expression of marker proteins alone did not cause fluorescence, suggesting that the N- and the C-terminal ends of ArsB were the vacuole lumen.
VBG localizes to the vacuole in differentiated leaf and root cells
The vacuolar R-SNARE VAMP711 is comprised of an N-terminal longin domain, central R-SNARE domain, and a C-terminal transmembrane region from aa 190 to 212 (). The N-terminal cytosolic longin domain of VAMP711 is required for recognition by the t-SNARE SYP22/AtVAM3 to localize to the vacuolar membrane.Citation24 We found that both, the N- and C-termini of ArsB, were exoplasmic (). Therefore, we fused VAMP711 and GFP to the N- and C-terminal ends of ArsB (VAMP711-ArsB-GFP), designated as VBG, and generated the VBG-expressing line under the 35S promoter (). The fluorescence of VBG overlapped with that of the central vacuolar membrane marker, mRFP-AtVAM3, in the cells within the differentiation zone of the root, and pavement cells in the leaves (), however, was not merged with mRFP-AtVAM3, or the late endosome marker, mRFP-SNX21, in the cell division zone of the roots (). Taken together, these results suggest that VBG localized to the central vacuole membrane in differentiated leaf and root tissue, but not within the cell division zone.
Figure 2. VAMP711-ArsB-GFP (VBG) localizes to the vacuole membrane
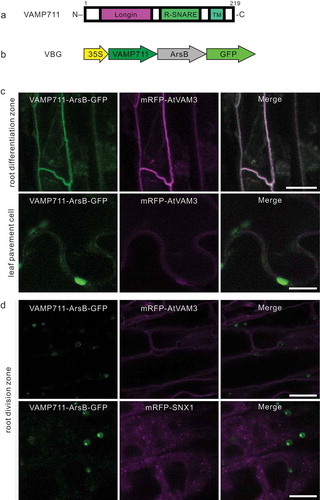
The VBG longin domain is located on the cytoplasmic side
VBG was transiently expressed in Agrobacterium-infiltrated leaves of N. benthamiana,Citation25,Citation26 and the leaves were treated with endomembrane marker FM4-64, which stains the PM. The fluorescence of VBG protein did not overlap with the plasma membrane marker FM4-64 (), suggesting that VBG was localized in the central vacuole membrane. The N-terminal portion of VBG was estimated to be on the cytoplasmic side as one transmembrane region of VAMP711 was fused to the exoplasmic N-terminus of ArsB (). In contrast, the C-terminal portion of VBG was predicted to be on the exoplasmic side as soluble GFP proteins lacking the transmembrane region fused to the exoplasmic C-terminus of ArsB (). To confirm these hypotheses, we evaluated the interaction between ciV-VAMP711-ArsB or VAMP711-ArsB-ciV and niV-fused markers using the iVenus assay. As shown in , ciV-VAMP711-ArsB and RHM1-niV (cytosolic marker), as well as VAMP711-ArsB-ciV and AALP-niV, were the fluorescent combinations, however, AALP-niV (vacuole lumen marker) and RHM1-niV were not observed to overlap, although fluorescence was not observed in cells expressing each marker separately (). Together these data suggest that the vacuole-targeting signal of VAMP711 in VBG is exposed on the cytoplasmic side. Moreover, the orientation and localization data for VBG, indicate that the VSMP711-tag functions as a vacuole-targeting signal to localize ArsB to the central vacuolar membrane in differentiated leaf and root cells.
Figure 3. N-terminus of VBG is located on the cytosolic side and C-terminus is on the vacuole lumen side
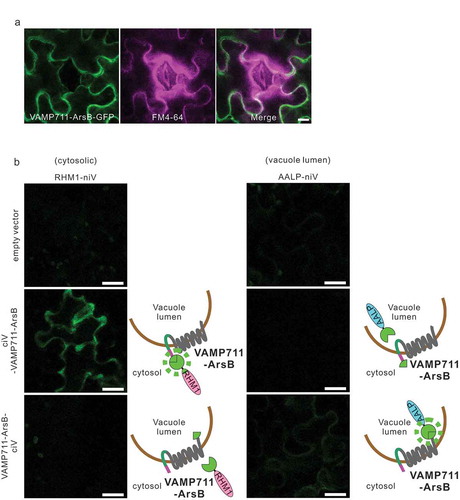
VBG-expressing plant exhibits arsenic accumulation but not arsenic tolerance
We then examined the tolerance of VBG-expressing plants against As by measuring their root length following growth in 1/2 MS medium containing 0, 5, or 10 μM of As(III). No difference in As(III) tolerance was detected between the wild-type (WT) and three distinct VGB lines (). Next, we measured the arsenic concentration in VBG-expressing plants by ICP-OES. Although only one VBG line (VBG #4-5) accumulated a some higher concentration of As(III) in the shoots than the WT (), other VBG-expressing lines (VBG #2-1, VBG #3-1) tended to accumulate more As(III) in the shoot and root compared to WT plants ( and ). Further, we measured the transcript levels of the VBG-expressing lines by real-time RT-PCR and observed that the VBG #4-5 exhibited the highest expression level of VBG transcript (Fig. S2) and As accumulation in shoot.
Figure 4. Growth assay of VBG plant at various As(III) concentrations
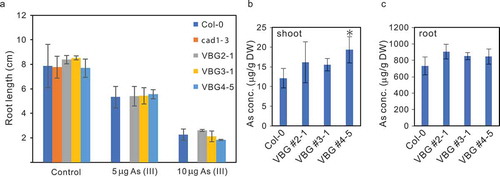
VBG-expressing plants exhibit high accumulation of various elements
Lastly, we measured the concentrations of other elements (Ca, Cu, Fe, Mg, Mn, Zn, S, and P), all of which, save for Cu and S, were found to be higher in the shoot of VBG-expressing plants compared to WT plants ().
Figure 5. Various microelement concentrations in lines expressing VBG under As(III) stress
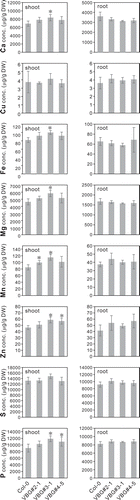
Discussion
Usage of VAMP711-tag led to generation of ArsB-introduced transgenic lines
The use of the VAMP711-tag led to generation of plant transgenic lines expressing ArsB. However, it is noted that we failed to generate transgenic Arabidopsis lines expressing GFP-ArsB as in the past studies, likely due to the toxicity of GFP-ArsB protein on plant cells. In the present study, we obtained transgenic plants expressing the chimerical construct VAMP711-ArsB-GFP, indicating that fusion with VAMP711 stabilized the structure of ArsB, thereby reducing its toxicity in plants.
Central vacuolar sequestration of As into VBG plants
VBG fluorescence did not merge with mRFP-AtVAM3 nor mRFP-SNX1 in root cell division zone (), indicating that VBG protein may reside in the small vacuole or an aberrant compartment induced by the chimerical construct in the root division zone.Citation27 In contrast, unlike the endosomal localization of GFP-ArsB during its transient expression (), VBG localized to the membrane of central vacuole () since the VAMP711-tag functions as a transport tag for the vacuole membrane in differentiated leaf and root tissues. Furthermore, VBG plants showed a tendency to accumulate As in the shoots, suggesting that the bacterial As transporter ArsB is functional in seed plants. To the best of our knowledge, this is the first study to demonstrate that accumulation of As increased by localizing free-As transporter to the vacuolar membrane in seed plants.
VBG plants did not show increased tolerance to As, compared with WT, which may be explained by root health being affected by VBG preferentially localizing to the membrane of the small vacuole or aberrant compartment, with a low capacity for As accumulation in root division zone cells (), but not in the central vacuole membrane. The As transporter VBG is illustrated in the intracellular map in Fig. S3.
On the other hand, root-to-shoot translocation of essential metals (Fe, Mn, Cu, and Zn) is inhibited in Arabidopsis growing on medium containing As.Citation28 However, among the concentrations of eight elements (Ca, Cu, Fe, Mg, Mn, Zn, S, and P) measured in the shoot and root, most elements, save for Cu and S, showed higher concentrations in VBG plants than in WT plants (), indicating that sequestration of As into the vacuole decreased the cytosolic As concentration in VBG plants, thereby increasing the root-to-shoot translocation of many nutrients.
In contrast, hyperaccumulators with high tolerance may be generated by leaf-specific expression of VBG while avoiding As accumulation in the root. Additionally, expressing the bacterial ATPase subunit ArsA in VBG plants may be effective for achieving hyperaccumulation and high tolerance of As.
Materials and methods
Plant materials
Arabidopsis thaliana ecotype Columbia (Col-0) was used as the WT species in all experiments. The following transgenic marker lines were used in accordance with previous studies: ST-mRFP,Citation29 mRFP-VHAa1,Citation29 SNX1-mRFP,Citation30 and mRFP-AtVAM3.Citation31 Six-week-old Nicotiana benthamiana plants grown on soil in a short-day light cycle (8 h light/16 h dark) at 22°C were used for Agrobacterium-mediated transient gene expressionCitation25,Citation26and the iVenus assay.
Generation of transgenic Arabidopsis lines expressing VAMP711-ArsB-GFP in the WT background
The cDNA fragments of ArsB and VAMP711 were prepared by amplification from the plasmid pI258 or Arabidopsis cDNA with primer ArsB-N-f/ArsB-C-r and VAMP711-N-f/VAMP711-C-r (Table 1). The resulting PCR fragments were cloned into the pENTR vector to generate the pENTR-VAMP711-ArsB construct. After confirming the sequences, these inserts were introduced into pGWB405 by LR cloning to generate the pGWB405-VAMP711-ArsB construct. Next, transgenic Arabidopsis expressing VAMP711-ArsB-GFP was generated by Agrobacterium-mediated floral dip transformation. Positive transformants were selected based on their kanamycin resistance. Homozygous T3 plants were used for all analyzes.
Arsenic tolerance assays
Arabidopsis seeds were surface-sterilized and germinated on 1/2 Murashige–Skoog (MS) gellan gum plates (1/2 MS salts, 1% sucrose, pH 5.7, solidified with 1.2% gellan gum) with 0, 5, or 10 μM As(III). All plates were incubated at 4°C in the dark for 2 days to synchronize their germination. The plates were then placed vertically, and the plants were grown under white light at 22°C under a 16-h/8-h (light/dark) photoperiod. Homozygous mutant plants were selected from the F2 segregating population based on genotyping and marker fluorescence. The root length was determined after several days of growth. All experiments were performed in a minimum of three independent biological replicates.
Total RNA isolation and cDNA synthesis
Total RNA was extracted from 6-day-old WT plants using the NucleoSpin RNA kit (TAKARA, Shiga, Japan) following the manufacturer’s instructions. The extracted total RNA was used for cloning of VAMP711, RHM1, and AALP and quantitative reverse transcription (RT)-PCR. For cDNA synthesis, 1 μg of total RNA was reverse-transcribed using ReverTra Ace qPCR RT Master Mix (TOYOBO, Osaka, Japan).
Plasmid constructs
All constructs were generated by Gateway cloning technology. To generate the ArsB, RHM1, AALP, and VAMP711-ArsB without the ArsB stop codon, their cDNA fragments were PCR-amplified and cloned into pENTR/D-TOPO vectors (Supplementary Table S1 and Table S2). Recombination with the Gateway recombinant cloning technology was performed to assemble multiple DNA fragments to generate the various constructs.
Quantitative RT-PCR
ArsB and UBQ10 cDNAs were amplified at an annealing temperature of 55°C for 40 cycles. The following primer pairs were used in qRT-PCR: ArsB-qRT-f/ArsB-qRT-r for ArsB and UBQ10-F/UBQ10-R for UBQ10.
Agrobacterium-mediated transient expression in Arabidopsis
GFP-ArsB was transiently expressed in intact Arabidopsis seedlings using the Agrobacterium-mediated transient expression system, AGROBEST (Agrobacterium-mediated enhanced seedling transformation).Citation32
Topology prediction
The web-versions of two different topology prediction methods were used to estimate membrane topology of ArsB and these were: MEMSATCitation33 and phobias.Citation34
iVenus, a self-assembled split fluorescent protein analysis
iVenus (auto-interactive Venus) is a split fluorescent protein method that can be used to examine the orientation of both ends of a protein. Venus shows strong fluorescence intensity, and fast and efficient maturation properties. We, therefore, developed a modified split fluorescent protein method in which the engineered self-assembling fragments of only a yellow fluorescent protein reconstitute and gives a fluorescent signal when the two domains co-localize in the same cellular compartment. The sites at which the N-terminus (niV) and C-terminus (ciV) domains split were selected as described by Ohashi et al.Citation23 The N-terminus Venus (M1–I189) was fused with marker proteins for the vacuole lumen, AALP; the cytoplasm, RHM1, and C-terminus Venus (ciV) fragments (E116–K239) were fused with the N- or C-terminus of VBG. A point mutation of A to K at residue 206 was introduced into the ciV tag to reduce dimer formation.Citation35 The niV-fused marker protein and ciV-fused VBG were transiently expressed in the leaves of Nicotiana benthamiana by Agrobacterium infiltration, and the leaves were examined using a Leica TCS SP8 confocal microscope (Wetzlar, Germany).
Vector constructs for iVenus
The ciV or niV fragments were amplified by PCR and inserted into the plasmid pGWB402, and digested by XbaI or AfeI/SacI to generate the binary vectors pGWB402NciV or pGWB402 CciV and pGWB402 CniV.
FM4-64 staining
Tobacco leaf epidermal cells were stained by infiltration of leaves with 10 µM of the red fluorescent FM4-64[N-(3-triethylammoniumpropyl)-4-(6-(4-(diethylamino)phenyl)hexatrienyl)pyridiniumdibromide].
Confocal microscopy
GFP, TagRFP, and Venus fluorescence signals, as well as differential interference contrast images were obtained using a Leica TCS SP8 laser scanning microscope, and the captured images were processed using Leica LAS X or ImageJ software (NIH, Bethesda, MD, USA). Imaging parameters for GFP and Venus were excitation at 488 nm and emission detection at 500–530, and FM4-64 TagRFP and mRFP, were excitation at 522 nm and emission detection at 574–594 nm, respectively.
Element analysis with ICP-OES
Element analysis of Arabidopsis plants was performed as described by Uraguchi et al. Briefly, Arabidopsis Col-0 and transgenic Arabidopsis lines were grown on 1/10th of modified Hoagland medium with 1.5% agar for 10 days. The seedlings were then transferred to control plates or plates containing 5 μM As(III) and incubated for an additional 4 days. Shoots and roots from each plate (15 plantlets per individual line) were separately pooled. The concentrations of elements in the samples were quantified by inductively coupled plasma-optical emission spectroscopy (ICP-OES; iCAP7400Duo, Thermo Fisher Scientific, Waltham, MA, USA).
Accession numbers
The following Arabidopsis Genome Initiative locus identifiers were used for genes mentioned in this manuscript: VAMP711(AT4G32150), UBQ10 (At4g05320), AALP (At5g60360), and RHM1 (At1g78570).
Author contributions
Yusuke Deromachi, Shimpei Uraguchi, Kazuhiro Kuga, and Kohji Nishimura performed the experiments and analyzed the data; Tomoko Hirano and Masa H. Sato designed the experiments and supervised the work; Tomoko Hirano drafted the paper. The authors declare no competing financial interests. Correspondence and requests for materials should be addressed to Tomoko Hirano ([email protected]).
Highlight
The first arsenic (As) accumulator was developed using the bacterial As transporter ArsB, and vacuole-targeting signals VAMP711 in a seed plant.
Supplemental Material
Download Zip (7.7 MB)Acknowledgments
We thank T. Nakagawa (Shimane University, Japan) for providing pGWB402, pGWB405, pGWB406 and pGWB460, and T. Uemura (Ochanomizu University, Japan) and T. Ueda (National Institute for Basic Biology) for providing ST-mRFP and VHA-a1-mRFP, and A. Miyawaki (the RIKEN BSI, Japan) for providing Venus. We thank G. Endo for providing the plasmid pI258.
Supplementary data:
Supplemental data for this article can be accessed on the publisher’s website.
Additional information
Funding
References
- Abbas G, Murtaza B, Bibi I, Shahid M, Niazi NK, Khan MI, Amjad M. Arsenic uptake, toxicity, detoxification, and speciation in plants: physiological, biochemical, and molecular aspects. Int J Environ Res Public Health. 2018;15:59.
- Li G, Santoni V, Maurel C. Plant aquaporins: roles in plant physiology. Biochim Biophys Acta - Gen Subj. 2014;1840:1–10.
- Mukhopadhyay R, Bhattacharjee H, Rosen BP. Aquaglyceroporins: generalized metalloid channels. Biochim Biophys Acta - Gen Subj 2014; 1840: 1583–1591.
- Stocken LA,Thompson RHS. Dithiol compounds as antidotes for arsenic. Biochem J. 1946;40:529–535.
- Stochen LA, Thompson RHS. British anti-lewisite; dithiol compounds as antidotes for arsenic. Biochem J. 1946;40:535–548.
- Zhao F, Mcgrath SP, Meharg AA. Arsenic as a food chain contaminant : mechanisms of plant uptake and metabolism and mitigation strategies. 2010;61:535–559.
- Song W-Y, Park J, Mendoza-Cozatl DG, Suter-Grotemeyer M, Shim D, Hortensteiner S, Geisler M, Weder B, Rea PA, Rentsch D, et al. Arsenic tolerance in Arabidopsis is mediated by two ABCC-type phytochelatin transporters. Proc Natl Acad Sci [Internet] 2010; 107: 21187–21192.
- Lombi E, Zhao FJ, Fuhrmann M, Ma LQ, McGrath SP. Arsenic distribution and speciation in the fronds of the hyperaccumulator Pteris vittata. New Phytol. 2002;156:195–203.
- Isidoria M, Gonzaga S, Antonio J, Santos G, Ma LQ. Arsenic phytoextraction and hyperaccumulation by fern species. Sci Agric. 2006;90–101.
- Nishizawa K, Maruyama N, Satoh R, Fuchikami Y, Higasa T, Utsumi SA. C-terminal sequence of soybean beta-conglycinin alpha’ subunit acts as a vacuolar sorting determinant in seed cells. Plant J 2003; 34: 647–659.
- Chen Y, Xu W, Shen H, Yan H, Xu W, He Z. Engineering arsenic tolerance and hyperaccumulation in plants for phytoremediation by a PvACR3 transgenic approach. Environ Sci Technol. 2013;47(16):9355–9362.
- Chen Y, Hua CY, Jia MR, Fu JW, Liu X, Han YH, Liu Y, Rathinasabapathi B, Cao Y, Ma LQ. Heterologous expression of pteris vittata arsenite antiporter PvACR3;1 Reduces arsenic accumulation in plant shoots. Environ Sci Technol. 2017;51:10387–10395.
- Chen Y, Hua CY, Chen JX, Rathinasabapathi B, Cao Y, Ma LQ. Expressing arsenite antiporter PvACR3;1 in rice (Oryza sativa L.) decreases inorganic arsenic content in rice grains. Environ Sci Technol. 2019;53:10062–10069.
- Ali W, Isner JC, Isayenkov SV, Liu W, Zhao FJ, Maathuis FJM. Heterologous expression of the yeast arsenite efflux system ACR3 improves Arabidopsis thaliana tolerance to arsenic stress. New Phytol. 2012;194:716–723.
- Rosenstein R, Peschel A, Wieland B, Gotz F. Arsenate resistance operon of staphylococcus xylosus plasmid pSX267. J Bacteriol. 1992;174:3676–3683.
- Ghosh M, Shen J, Rosen BP, Kaback HR. Pathways of As(III) detoxification in saccharomyces cerevisiae (ACR3͞YCF1͞Sb(III)͞resistance͞ABC transporters. Cell Biol. 1999;96:5001–5006.
- Carlin A, Shi W, Dey S, Rosen BP. The ars operon of escherichia coli confers arsenical and antimonial resistance. J Bacteriol. 1995;177:981–986.
- Bruhn DF, Li J, Silver S, Roberto F, Rosen BP. The arsenical resistance operon of IncN plasmid R46. FEMS Microbiol Lett. 1996;139:149–153.
- Kiyono M, Oka Y, Sone Y, Tanaka M, Nakamura R, Sato MH, Pan-Hou H, Sakabe K, Inoue KI. Expression of the bacterial heavy metal transporter MerC fused with a plant SNARE, SYP121, in Arabidopsis thaliana increases cadmium accumulation and tolerance. Planta. 2012;235:841–850.
- Ji G, Silver S. Regulation and expression of the arsenic resistance operon from staphylococcus aureus plasmid pI258. J Bacteriol. 1992;174:3684–3694.
- Meng YL, Liu Z, Rosen BP. As(III) and Sb(III) uptake by GlpF and efflux by ArsB in escherichia coli. J Biol Chem. 2004;279:18334–18341.
- Wu J, Tisa LS, Rosen BP. Membrane topology of the ArsB protein, the membrane subunit of an anion- translocating ATPase. J Biol Chem. 1992;267:12570–12576.
- Ohashi K, Kiuchi T, Shoji K, Sampei K, Mizuno K. Visualization of cofilin-actin and Ras-Raf interactions by bimolecular fluorescence complementation assays using a new pair of split venus fragments. Biotechniques. 2012;52:45–50.
- Uemura T, Sato MH, Takeyasu K. The longin domain regulates subcellular targeting of VAMP7 in Arabidopsis thaliana. FEBS Lett. 2005;579:2842–2846.
- Sparkes IA, Runions J, Kearns A, Hawes C. Rapid, transient expression of fluorescent fusion proteins in tobacco plants and generation of stably transformed plants. Nat Protoc. 2006;1:2019–2025.
- Kanneganti TD, Huitema E, Kamoun S. In planta expression of oomycete and fungal genes. Methods Mol Biol. 2007;354:35–43.
- Di Sansebastiano GP, Rizzello F, Durante M, Caretto S, Nisi R, De Paolis A, Faraco M, Montefusco A, Piro G, Mita G. Subcellular compartmentalization in protoplasts from Artemisia annua cell cultures: engineering attempts using a modified SNARE protein. J Biotechnol 2015; 202: 146–152.
- Uraguchi S, Sone Y, Ohta Y, Ohkama-ohtsu N, Hofmann C, Hess N, Nakamura R, Takanezawa Y, Clemens S, Kiyono M. Identification of C-terminal regions in arabidopsis thaliana phytochelatin synthase 1 specifically involved in activation by arsenite. Plant Cell Physiol. 2018;2:500–509.
- Uemura T, Kim H, Saito C, Ebine K, Ueda T, Schulze-lefert P. Qa-SNAREs localized to the trans -Golgi network regulate multiple transport pathways and extracellular disease resistance in plants. Proc Natl Acad Sci U S A. 2012;109(5):1784–1789.
- Jaillais Y, Fobis-loisy I, Miege C, Rollin C, Gaude T. AtSNX1 defines an endosome for auxin-carrier trafficking in Arabidopsis. Nature. 2006;443:106-109.
- Ebine K, Okatani Y, Uemura T, Goh T, Shoda K, Niihama M, Morita MT, Spitzer C, Otegui MS, Nakano A, et al. A SNARE complex unique to seed plants is required for protein storage vacuole biogenesis and seed development of Arabidopsis thaliana. Plant Cell. 2008;20:3006–3021.
- Wu H, Liu K, Wang Y, Wu J, Chiu W, Chen C. AGROBEST : an efficient agrobacterium -mediated transient expression method for versatile gene function analyses in Arabidopsis seedlings. Plant Methods. 2014;1–16.
- Jones DT, Taylor WR, Thornton JM. A model recognition approach to the prediction of all-helical membrane protein structure and topology. Biochemistry. 1994;33:3038–3049.
- Käll L, Krogh A, Sonnhammer ELL. Advantages of combined transmembrane topology and signal peptide prediction-the phobius web server. Nucleic Acids Res. 2007;35:429–432.
- Zacharias DA, Violin JD, Newton AC, Tsien RY. Partitioning of lipid-modified monomeric GFPs into membrane microdomains of live cells. Science ( 80-). 2002;296:913–916.