ABSTRACT
Plasmopara viticola, the casual oomycete of grapevine downy mildew, could cause yield loss and compromise berry quantity. Previously, we have identified several PvRXLR effectors that could suppress plant immunity to promote infection and disease development. In this study, the role of effector, PvRXLR53, in plant–microbe interaction was investigated. PvRXLR53 has several orthologs in other oomycetes and contains a functional signal peptide. Expression level of PvRXLR53 was already detected upon inoculation, further induced in the early stage after P. viticola inoculation and decreased to low level in the late infection stage in grapevine (Vitis vinifera ‘Cabernet Sauvignon’). PvRXLR53 is localized in both nucleus and cytoplasm. When transiently expressed in Nicotiana benthamiana, PvRXLR53 suppressed oomycete elicitor INF1-triggered programmed cell death and defense gene expression, and Phytophthora capsici-induced reactive oxygen species production (ROS) and eventually resistance to P. capsici. In summary, these findings suggest that P. viticola secretes PvRXLR53 to suppress host immunity from the very early stage of infection.
Introduction
Through long-term co-evolutionary struggle, plants have evolved complicated and highly effective innate immune defense system to resist attack by microbial pathogens.Citation1,Citation2 Recent molecular studies of the plant–pathogen interaction reveal that plants have evolved two layers of immune responses to resist pathogens. PAMP-triggered immunity (PTI) acts as the first one, which effectively prevents a broad range of potential pathogens by detection of conserved pathogen-associated molecular patterns (PAMPs).Citation1,Citation3 However, a variety of effectors are secreted by plant pathogens to manipulate plant immunity thereby interfering with PTI and facilitating infection.Citation4,Citation5 In response, plants acquire resistance proteins (R proteins) to monitor effectors specifically, resulting in effector-triggered immunity (ETI).Citation2 ETI is a kind of faster and stronger disease resistance response. Due to the natural selection, pathogens are compelled to avoid ETI either by escaping from the recognition via shedding or diversifying, or by acquiring additional effectors. Programmed cell death (PCD), callose depositions, production of reactive oxygen species (ROS), and a series of defense-related genes expression are common plant immune responses.Citation6 Numerous studies have shown that pathogen effectors tend to suppress these defense responses to promote pathogenicity.
The obligately biotrophic Plasmopara viticola is the agent of grapevine downy mildew, one of the most destructive disease of the global grape and wine industry.Citation7–9 It is known that P. viticola secrets a series of effectors containing the RXLR motif (for Arg, any amino acid, Leu and Arg) to suppress host immunity.Citation7 At least 188 candidate P. viticola RXLR effectors have been reported in previous studies, and some of which have been functionally characterized.Citation10–15 For example, the virulence function of PvRXLR131 is mediated by interacting with plant BRI1 kinase inhibitor 1 to promote infection.Citation16 During P. viticola infection, PvRXLR159 is induced dramatically and secreted into plant to suppress host resistance.Citation17 Here, we unravel the role of PvRXLR53 in pathogen–plant interaction to understand the infection strategies of the plant pathogens.
Materials and methods
Bioinformatics analysis
Multiple amino acid sequences were aligned using BioEdit3.3.19.0 software with ClustalW. The signal peptide of PvRXLR53 was predicted by SingalP5.0 Server (http://www.cbs.dtu.dk/services/SignalP/).Citation18
Plant materials and culture conditions
The grapevine (Vitis vinifera ‘Cabernet Sauvignon’) and Nicotiana benthamiana were both grown in a greenhouse at 25°C under 16/8 h light/dark.
Gene cloning and vector construction
The full-length of predicted PvRXLR53 genes was amplified using 2 × PrimeSTAR Max DNA Polymerase (Takara Bio Inc., Beijing, China). The open reading frame of PvRXLR53 lacking the signal peptide sequence was ligated into the vector both pGR106 (Potato virus X-based binary expression vector, PVX) and pHB-GFP. The signal peptide of PvRXLR53 was ligated into vector pSUC2 (pSUC2T7M13jORI).Citation19 Green fluorescent protein, GFP. The primers are listed in Table S1.
Yeast signal sequence trap assay
To validate the function of the predicted signal peptide of PvRXLR53, the signal sequence trap assay was performed using the yeast strain YTK12 and the recombinant plasmid pSUC2-PvRXLR53 according to previous study.Citation20–22
Agrobacterium tumefacien-mediated transient expression in N. benthamiana
Agrobacterium tumefaciens GV3101 carrying the indicated plasmids were used for transient expression in N. benthamiana according to our previous methods.Citation23,Citation24 For subcellular localization, the transferred A. tumefaciens cultures containing PvRXLR53-GFP/GFP were infiltrated in N. benthamiana leaves, respectively. The accumulation of fluorescence signals was monitored 2 days post-infiltration using a confocal microscope (Leica TCS SP5II).Citation25
Pathogen infection assay
Each grapevine leaf disc (1 cm in diameter) was inoculated with 20 μL of 1 × 104 sporangia/mL P. viticola suspension on the abaxial surface. Excess water was removed at 36 hours post inoculation (hpi).Citation26 The samples were taken at 0, 6, 12, 36, 72 and 120 hpi for qRT-PCR analysis.Citation27
For P. capsici infection assay, agar discs (5 mm in diameter) harboring mycelium were inoculated on the abaxial surface of excised N. benthamiana leaves according to the methods previously reported.Citation28 The lesion areas were measured at 48 hpi by Image J software.
DAB staining
Reactive oxygen species production was analyzed by 3, 3ʹ-diaminobenzidine (DAB) staining. The excised leaves were placed in 1 mg/mL DAB (dissolved in 50 mM Tris-HCl, pH 5.0) and vacuum infiltrated three times for 5 min until the leaves were entirely soaked. And then the soaked leaves were maintained for 24 h in the dark. The leaf tissues were decolorized in boiling 95% ethanol for 10 min and subjected to imaging.Citation29
Electrolyte leakage assay
Electrolyte leakage assay was performed to analyze the cell death of N. benthamiana.Citation30 For each sample, five leaf discs (8 mm in diameter) were immersed into 5 mL sterile water for 3 h. The conductivity was detected using a conductivity meter (DDS-307, Rex Shanghai, China). Electrolyte leakage (%) = 100 × A/B. The ‘value A’ and ‘value B’ represent the conductivity before and after boiling. All experiments were repeated three times.
RNA extraction and real-time quantitative RT-PCR
The RNA extraction kit (OMEGA, Norcross, GA, USA), iScript cDNA Synthesis kit (BIO-RAD, Hercules, CA, USA) and iTaq Universal SYBR Green Supermix (BIO-RAD, Hercules, CA, USA) were respectively used for RNA extraction, cDNA synthesis and quantitative RT-PCR reactions in our study.Citation31 The primers used are listed in Table S1.
Protein extraction and western blotting
Total proteins were extracted according to previous studies.Citation32 The proteins of plant tissues were extracted in 50 mM Tris-HCl (pH 7.5), 150 mM NaCl, 1 mM EDTA, 0.2% (v/v) Triton X-100, 1 × protease inhibitor cocktail. The extract was boiled with 5 × SDS loading buffer at 100°C for 5 min and detected by western blotting.
Statistical analysis
The SPSS 20 (SPSS Inc., Chicago, IL) statistical software was used to determine the significant differences. All reaction data of qRT-PCR were analyzed using BIO-RAD CFX software.
Results
Identification and sequence analysis of effector PvRXLR53
About 100 genes encoding candidate RXLR effectors were identified in the genome of P. viticola isolate “JL-7-2”Citation11 One of them, PvRXLR53, encompassed an N-terminal signal peptide with 20 amino acids and a conserved RXLR motif.Citation7 Generally, PvRXLRs effector shows only limited similarity to RXLR effectors identified from other oomycete species.Citation11 In the current study, however, PvRXLR53 was found being comparatively conserved across oomycetes as demonstrated that it had more than 47% sequence similarity with Plasmopara halstedii, Phytophthora infestans, Phytophthora parasitica, Phytophthora fragariae, and Phytophthora sojae (). The amino acid sequence alignments of the clustered RXLRs revealed that these proteins had an especially higher sequence similarity in the C-terminal domain, a functional domain contributing to the effector activity.
Figure 1. Amino acid sequence alignment of PvRXLR53 and the other similar RXLR effector proteins from different oomycete species
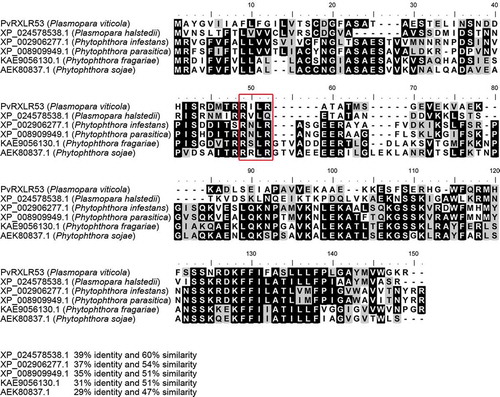
PvRXLR53 is expressed upon infection and further induced
To investigate the expression pattern of the effector PvRXLR53 during P. viticola attacking the grapevine, qRT-PCR was performed to analyze its expression levels at different time points post inoculation. Expression of PvRXLR53 was already detected upon inoculation, peaked at 6 hpi and then decreased to low level (). This indicates that the effector PvRXLR53 might contribute to the virulence during the infection of P. viticola to grapevine.
Figure 2. Functional characterization of effector PvRXLR53 during the pathogen infection
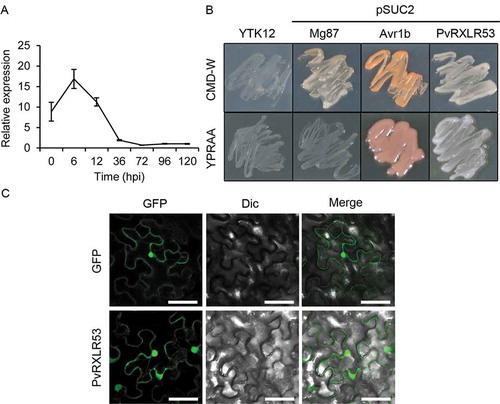
PvRXLR53 contains a functional signal peptide
The N-terminal 20 amino acids of PvRXLR53 were predicted by singalP5.0 to be a signal peptide with a high probability of 0.9494. The signal sequence trap (SST) assay was performed to validate the secretory activity of the signal peptide in PvRXLR53. The untransformed YTK12 strain and the YTK12 carrying pSUC2-Mg87 vector were used as negative controls, while the YTK12 carrying pSUC2-Avr1b vector was as a positive one. In accord with observation, YTK12 yeast cells carrying pSUC2-PvRXLR53 were able to utilize raffinose as the sole carbon source to grow on YPRAA medium consistent with the positive control, while the negative control yeast strain and the YTK12 strain without transformation could not (). This result confirmed that the effector PvRXLR53 contained a functional signal peptide.
PvRXLR53 is localized in both nucleus and cytoplasm
To determine the localization of PvRXLR53 in plants, PvRXLR53 with green fluorescent protein (GFP) fused to its C terminus was transiently expressed in N. benthamiana leaves. GFP was served as control. The accumulation of fluorescence was visualized at 48 h post-infiltration by confocal microscopy. The results indicate that PvRXLR53 is localized in both nucleus and cytoplasm ().
PvRXLR53 suppresses INF1-triggered cell death and the expression levels of the defense-related genes in N. benthamiana
Oomycete PAMP INF1 is known to trigger a series of plant immune responses.Citation33,Citation34 We investigated the effects of PvRXLR53 on INF1-triggered immunity. The PvRXLR53 and GFP were transiently expressed in N. benthamiana 24 h followed by expression of INF1, respectively. As shown in , instead of GFP, PvRXLR53 suppressed the INF1-induced PCD in N. benthamiana. The suppression by PvRXLR53 was further confirmed by ion leakage measurement ().
Figure 3. Suppression by PvRXLR53 of INF1-induced immune responses
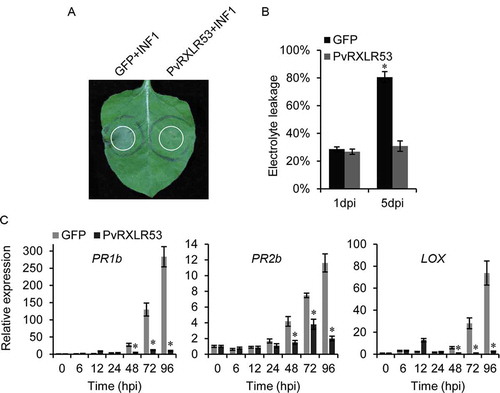
In addition, the effect of PvRXLR53 on INF1-induced transcription of defense-related genes, PR1b, PR2b and LOX,Citation35,Citation36 was also investigated in N. benthamiana by qRT-PCR. As shown in , INF1-triggered NbPR1b, NbPR2b and NbLOX were all significantly suppressed by the expression of PvRXLR53. Taken together, these results clearly indicate that PvRXLR53 could suppress plant basal immune responses in N. benthamiana.
PvRXLR53 enhances plant susceptibility to Phytophthora capsici in N. benthamiana
In order to explore the virulence contribution of PvRXLR53, we investigated the effect of PvRXLR53 during a pathogenic oomycete P. capsici infecting N. benthamiana. When PvRXLR53 and GFP were transiently expressed in N. benthamiana leaves via agroinfiltration followed by inoculation with equal area of P. capsici mycelium, effector PvRXLR53 clearly suppressed the ROS production as indicated by DAB staining (). Meanwhile, the effector also significantly enlarged the lesion size induced by P. capsici (, c) at 2 dpi. To validate the suppression of ROS production and P. capsici infectivity was indeed due to the expression of the effector, PvRXLR53 protein was confirmed by western blotting (). These results indicate that PvRXLR53 enhances N. benthamiana susceptibility to P. capsici.
Figure 4. Inhibition of ROS-mediated resistance and enhanced susceptibility to P. capsici by PvRXLR53 in N. benthamiana.
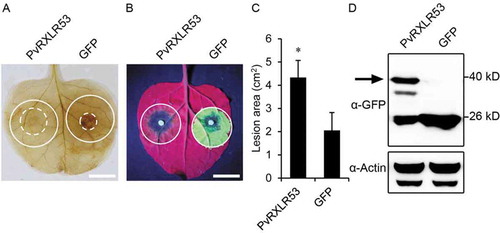
Conclusions
In this study, we characterized the function PvRXLR53 with the following results: 1) PvRXLR53 may have orthologs in P. halstedii, P. infestans, P. parasitica, P. fragariae, and P. sojae; 2) it was constitutively expressed in P. viticola and its expression only dominated in the early P. viticola infection stage on grapevine; 3) it has a functional signal peptide and is localized in nucleus and cytoplasm; 4) transient expression of PvRXLR53 suppressed INF1-triggered programmed cell death and the expression of the defense-related genes, and P. capsici-induced ROS production making N. benthamiana more susceptible to P. capsici infection. These findings lead us to hypothesize that P. viticola secretes PvRXLR53 to suppress host immunity from the very early stage of infection. Future research is needed to identify the host targets of PvRXLR53 to further understand its working modes in plants.
Disclosure of Potential Conflicts of Interest
No potential conflicts of interest were disclosed.
Supplemental Material
Download MS Power Point (43.9 KB)Acknowledgments
This work was supported by National Key R & D Program of China (2018 YFD 1000301 to J.L., 2019 YFD1002500 to S.S), Shanghai Science and Technology Commission (18391900400 to J.L.), the Start-up Fund from Shanghai Jiao Tong University (WF107115001 to J.L. and WF220515002 to W.Y.), China Agriculture Research System (CARS-29-yc-2 to J.L.), National Natural Science Foundation of China (31901984 to W.Y.) and Shanghai Natural Science Foundation (19ZR1428000 to S.S.).
Supplementary Material
Supplemental data for this article can be accessed on the publisher’s website
References
- Dodds PN, Rathjen JP. Plant immunity: towards an integrated view of plant–pathogen interactions. Nat Rev Genet. 2010;11(8):1–6. doi:10.1038/nrg2812.
- Chisholm ST, Coaker G, Day B, Staskawicz BJ. Host-microbe interactions: shaping the evolution of the plant immune response. Cell. 2006;124:803–814.
- Boller T, He SY. Innate immunity in plants: an arms race between pattern recognition receptors in plants and effectors in microbial pathogens. Science. 2009;324(5928):742–744. doi:10.1126/science.1171647.
- Kamoun SA. Catalogue of the effector secretome of plant pathogenic oomycetes. Annu Rev Phytopathol. 2006;44(41–60):41–60. doi:10.1146/annurev.phyto.44.070505.143436.
- Dangl JL, Horvath DM, Staskawicz BJ. Pivoting the plant immune system from dissection to deployment. Science. 2013;341(6147):746–751. doi:10.1126/science.1236011.
- Jones JDG, Dangl JL. The plant immune system. Nature. 2006;444(7117):323–329. doi:10.1038/nature05286.
- Morgan W, Kamoun S. RXLR effectors of plant pathogenic oomycetes. Curr Opin Microbiol. 2007;10(4):332–338. doi:10.1016/j.mib.2007.04.005.
- Kamoun S. Molecular genetics of pathogenic oomycetes. Eukaryotic Cell. 2003;2(2):191–199. doi:10.1128/ec.2.2.191-199.2003.
- Birch PRJ, Rehmany AP, Pritchard L, Kamoun S, Beynon JL. Trafficking arms: oomycete effectors enter host plant cells. Trends Microbiol. 2006;14(1):8–11. doi:10.1016/j.tim.2005.11.007.
- Chen T, Liu R, Mengru D, Li M, Li M, Yin X, Liu G-T, Wang Y, Xu Y. Insight into function and subcellular localization of plasmopara viticola putative RxLR effectors. Front Microbiol. 2020. doi:10.3389/fmicb.2020.00692.
- Yin L, An Y, Qu J, Li X, Zhang Y, Dry I, Wu H, Lu J. Genome sequence of Plasmopara viticola and insight into the pathogenic mechanism. Sci Rep. 2017;7(1):46553. doi:10.1038/srep46553.
- Xiang J, Li X, Wu J, Yin L, Zhang Y, Lu J. Studying the mechanism of plasmopara viticola RxLR effectors on suppressing plant immunity. Front Microbiol. 2016:7. doi:10.3389/fmicb.2016.00709.
- Yin L, Li X, Xiang J, Qu J, Zhang Y, Dry IB, Lu J. Characterization of the secretome of Plasmopara viticola by de novo transcriptome analysis. Physiol Mol Plant Pathol. 2015;91(1–10):1–10. doi:10.1016/j.pmpp.2015.05.002.
- Liu Y, Lan X, Song S, Yin L, Dry IB, Qu J, Xiang J, Lu J. In planta functional analysis and subcellular localization of the oomycete pathogen plasmopara viticola candidate RXLR effector repertoire. Front Plant Sci. 2018:9. doi:10.3389/fpls.2018.00286.
- Xiang J, Li XL, Yin L, Liu YX, Zhang YL, JJ Q, Lu J. A candidate RxLR effector from Plasmopara viticola can elicit immune responses in Nicotiana benthamiana. BMC Plant Biol. 2017;17(14). doi:10.1186/s12870-017-1016-4.
- Lan X, Liu YX, Song SR, Yin L, Xiang J, JJ Q, Lu J. Plasmopara viticola effector PvRXLR131 suppresses plant immunity by targeting plant receptor-like kinase inhibitor BKI1. Mol Plant Pathol. 2019;20(6):765–783. doi:10.1111/mpp.12790.
- Lei X, Lan X, Ye W, Liu Y, Song S, Lu J. Plasmopara viticola effector PvRXLR159 suppresses immune responses in Nicotiana benthamiana. Plant Signal Behav. 2019;14(12):1682220. doi:10.1080/15592324.2019.1682220.
- Almagro Armenteros JJ, Tsirigos KD, Sønderby CK, Petersen TN, Winther O, Brunak S, von Heijne G, Nielsen H. SignalP 5.0 improves signal peptide predictions using deep neural networks. Nat Biotechnol. 2019;37(4):420–423. doi:10.1038/s41587-019-0036-z.
- Satarug S, Lang MA, Yongvanit P, Sithithaworn P, Mairiang E, Mairiang P, Pelkonen P, Bartsch H, Haswell-Elkins MR. Induction of cytochrome P450 2A6 expression in humans by the carcinogenic parasite infection, opisthorchiasis viverrini. Cancer Epidemiol Biomarkers Prev. 1996;5:795–800.
- Oh S-K, Young C, Lee M, Oliva R, Bozkurt TO, Cano LM, Win J, Bos JIB, Liu H-Y, van Damme M, et al. In planta expression screens of phytophthora infestans RXLR effectors reveal diverse phenotypes, including activation of the solanum bulbocastanum disease resistance protein Rpi-blb2. Plant Cell. 2009;21(9):2928–2947. doi:10.1105/tpc.109.068247.
- Klein RD, Gu Q, Goddard A. Selection for genes encoding secreted proteins and receptors. Proc Natl Acad Sci U S A. 1996;93(14):7108–7113. doi:10.1073/pnas.93.14.7108.
- Gietz RD, Schiestl RH, Willems AR, Woods RA. Studies on the transformation of intact yeast cells by the LiAc/SS-DNA/PEG procedure. Yeast. 1995;11(4):355–360. doi:10.1002/yea.320110408.
- Zheng X, McLellan H, Fraiture M, Liu X, Boevink PC, Gilroy EM, Chen Y, Kandel K, Sessa G, Birch PRJ, et al. Functionally redundant RXLR effectors from phytophthora infestans act at different steps to suppress early flg22-triggered immunity. PLoS Pathog. 2014;10(4):e1004057. doi:10.1371/journal.ppat.1004057.
- Yang H, Yang S, Li Y, Hua J. The Arabidopsis BAP1 and BAP2 genes are general inhibitors of programmed cell death. Plant Physiol. 2007;145(1):135–146. doi:10.1104/pp.107.100800.
- Xu F, Li T, Xu P-B, Li L, Du -S-S, Lian H-L, Yang H-Q. DELLA proteins physically interact with CONSTANS to regulate flowering under long days in Arabidopsis. FEBS Lett. 2016;590(4):541–549. doi:10.1002/1873-3468.12076.
- Li X, Yin L, Ma L, Zhang Y, An Y, Lu J. Pathogenicity variation and population genetic structure of plasmopara viticola in China. J Phytopathol. 2016;164(11–12):863–873. doi:10.1111/jph.12505.
- Malacarne G, Vrhovsek U, Zulini L, Cestaro A, Stefanini M, Mattivi F, Delledonne M, Velasco R, Moser C. Resistance to Plasmopara viticolain a grapevine segregating population is associated with stilbenoid accumulation and with specific host transcriptional responses. BMC Plant Biol. 2011;11(1):114. doi:10.1186/1471-2229-11-114.
- Yang B, Wang Y, Guo B, Jing M, Zhou H, Li Y, Wang H, Huang J, Wang Y, Ye W, et al. The phytophthora sojae RXLR effector Avh238 destabilizes soybean Type2 GmACSs to suppress ethylene biosynthesis and promote infection. New Phytologist. 2019;222(1):425–437. doi:10.1111/nph.15581.
- Thordal-Christensen H, Zhang Z, Wei Y, Collinge DB. Subcellular localization of H2O2 in plants. H2O2 accumulation in papillae and hypersensitive response during the barley—powdery mildew interaction. Plant J. 1997;11(6):1187–1194. doi:10.1046/j.1365-313X.1997.11061187.x.
- Yu X, Tang J, Wang Q, Ye W, Tao K, Duan S, Lu C, Yang X, Dong S, Zheng X, et al. The RxLR effector Avh241 from Phytophthora sojae requires plasma membrane localization to induce plant cell death. New Phytologist. 2012;196(1):247–260. doi:10.1111/j.1469-8137.2012.04241.x.
- Zhang Y, Meng Q, Zhu H, Guo Y, Gao H, Luo Y, Lu J. Functional characterization of a LAHC sucrose transporter isolated from grape berries in yeast. Plant Growth Regul. 2007;54(71–79):71–79. doi:10.1007/s10725-007-9226-7.
- Mackey D, Belkhadir Y, Alonso JM, Ecker JR, Dangl JL. Arabidopsis RIN4 is a target of the type III virulence effector AvrRpt2 and Modulates RPS2-mediated resistance. Cell. 2003;112(3):379–389. doi:10.1016/S0092-8674(03)00040-0.
- Kanzaki H, Saitoh H, Takahashi Y, Berberich T, Ito A, Kamoun S, Terauchi R. NbLRK1, a lectin-like receptor kinase protein of Nicotiana benthamiana, interacts with Phytophthora infestans INF1 elicitin and mediates INF1-induced cell death. Planta. 2008;228(6):977–987. doi:10.1007/s00425-008-0797-y.
- Bonnet P, Bourdon E, Ponchet M, Blein JP, Ricci P. Acquired resistance triggered by elicitins in tobacco and other plants. Er J Plant Pathol. 1996;102(2):181–192. doi:10.1007/BF01877105.
- Kawamura Y, Hase S, Takenaka S, Kanayama Y, Yoshioka H, Kamoun S, Takahashi H. INF1 elicitin activates jasmonic acid‐ and ethylene‐mediated signalling pathways and induces resistance to bacterial wilt disease in tomato. J Phytopathol. 2010;157(5):287–297. doi:10.1111/j.1439-0434.2008.01489.x.
- Kanzaki H, Saitoh H, Ito A, Fujisawa S, Kamoun S, Katou S, Yoshioka H, Terauchi R. Cytosolic HSP90 and HSP70 are essential components of INF1-mediated hypersensitive response and non-host resistance to Pseudomonas cichorii in Nicotiana benthamiana. Mol Plant Pathol. 2003;4(383–391):383–391. doi:10.1046/j.1364-3703.2003.00186.x.