ABSTRACT
Quantitative reverse transcription PCR (qRT-PCR) analysis and ProACO2::GUS expression showed that ACO2 was highly expressed in the shoots of Arabidopsis seedlings under light conditions. Exogenously applied aminocyclopropane-1-carboxylic acid (ACC) enhanced the expression of ACO2, whereas Co2+ ions suppressed its expression. In comparison with wild-type seedlings, the ACO2 knockdown mutant aco2-1 produced less ethylene, which resulted in the inhibited growth of Arabidopsis seedlings. Exogenously applied brassinolide reduced the expression of ACO2. ACO2 expression was increased in det2, a brassinosteroid (BR)-deficient mutant; however, it was decreased in bes1-D, a brassinosteroid insensitive 1-EMS-suppressor 1 (BES1)-dominant mutant. In the putative promoter region of ACO2, 11 E-box sequences for BES1 binding but not BR regulatory element sequences for brassinazole-resistant 1 (BZR1) binding were found. Chromatin immunoprecipitation assay showed that BES1 could directly bind to the E-boxes located in the putative promoter region of ACO4. Less ethylene was produced in bes1-D seedlings compared with wild-type seedlings, suggesting that the direct binding of BES1 to the ACO2 promoter may negatively regulate ACO2 expression to control the endogenous level of ethylene in Arabidopsis seedlings.
Ethylene is a gaseous hormonal regulator of various developmental and physiological processes in the life cycle of plants.Citation1-3 Therefore, it is crucial that plants can precisely control their ethylene levels in space and time. In plants, ethylene is biosynthesized from S-adenosyl-L-methionine (SAM), with 1-aminocyclopropane-1-carboxylic acid (ACC) as an intermediate.Citation4,Citation5 ACC synthases (ACSs) and ACC oxidases (ACOs) are known to catalyze the conversion of SAM to ACC and ACC to ethylene, respectively.Citation6-8 Initially, the conversion of SAM to ACC catalyzed by ACSs was regarded as rate-limiting, directing many studies to unravel the regulation of ACS activity and stability.Citation9-11 Extensive evidence gathered over the years suggests that the conversion of ACC to ethylene by ACOs is another important regulatory reaction in ethylene biosynthesis during certain processes in plants.Citation12 Nevertheless, compared with ACSs, the biochemical and physiological functions of ACOs in plants remain largely unknown.
Brassinosteroids (BRs) are steroidal plant hormones that regulate a wide range of growth and developmental processes in plants.Citation13-18 Recent studies have demonstrated that BRs interact with other plant hormones such as abscisic acid, gibberellins, auxin, cytokinin, and ethylene to control plant growth and development.Citation19 In Arabidopsis thaliana, exogenously applied BRs can increase ethylene production and endogenous levels of ACC, suggesting that the interaction of BRs and ethylene is mediated by the ethylene biosynthetic enzymes ACSs and ACOs.Citation20 Indeed, the expression and stability of several ACSs (ACS4, ACS5, ACS6, and ACO9) can be enhanced by applying BRs, which increase ethylene production in the plant.Citation21-23 Bingsheng et al. reported that BR signaling transcription factors such as brassinosteroid insensitive 1-EMS-suppressor 1 (BES1) and brassinazole-resistant 1 (BZR1) directly interacted with the promoters of ACS7, ACS9, and ACS11 to regulate their expression, suggesting that a native regulation mechanism for ethylene production by BR signaling via ACSs is present in A. thaliana.Citation24 Recently, we demonstrated that the expression of two functional ACOs in Arabidopsis (ACO1 and ACO4) is upregulated or downregulated by the direct binding of BES1 or BZR1 to cis-regulatory elements (E-box or BR regulatory element (BRRE)) in the intergenic regions of the genes.Citation25 Following their binding, the endogenous level of ethylene is controlled, indicating BR-induced ethylene production via ACO1 and ACO4 in Arabidopsis. Besides ACO1 and ACO4, ACO2 is also considered as a functional ACO gene in Arabidopsis,Citation26 suggesting that BR-induced ethylene production via ACOs may also be mediated by ACO2 in plants. To expand the knowledge on BR-induced ethylene production via ACOs, the molecular mechanism of the regulation of ACO2 by BR signaling was examined in this study.
Quantitative reverse transcription PCR (qRT-PCR) analysis revealed that the expression of ACO2 was dominant in the shoots rather than the roots of light-grown Arabidopsis seedlings (). Transgenic Arabidopsis seedlings expressing the GUS gene driven by the ACO2 promoter (1820 bp) showed strong GUS activity in the vascular streams from the cotyledon to the elongation zone in the roots via the cotyledon, hypocotyl, hypocotyl-root junction, and root maturation zone (). No GUS activity was found in the tips of the primary and lateral roots (). The spatiotemporal expression of ACO2 suggested that ACO2 seems to exert its physiological activity under light conditions in A. thaliana from the cotyledon to the root apical meristematic zone via the vascular system. Exogenously applied ACC activated the expression of ACO2, whereas Co2+ ions inhibited its expression in Arabidopsis seedlings (). Additionally, compared with wild-type seedlings, aco2-1 seedlings (ACO2 knockdown mutant; a T-DNA mutant line (SALK_044146, Supplementary Figure S1) obtained from the Arabidopsis Biological Resource Center produced less ethylene in the roots and shoots (), which retarded the growth of aco2-1 under light conditions (). These findings indicated that ACO2 may play a role in controlling ethylene production for the growth and development of A. thaliana.
Figure 1. Expression and physiological functions of ACO2. (a) ACO2 expression in 5 DAG seedlings grown under light and dark conditions. (b) Comparison of ACO2 expression in the shoots and roots of seedlings. (c-i) Spatiotemporal expression pattern of ACO2 in ProACO2::GUS transgenic plants under light conditions. Images show the whole seedling (c), cotyledon (d and e), junction of the shoots and roots (f), and roots (g-i). (j-l) Effect of ACC (1 µM) and CoCl2 (1 µM) on the expression of ACO2 in 5 DAG seedlings under light conditions. Semi-qRT-PCR was performed with total RNA. UBQ5 was used to normalize the expression level. Two biological replicates along with three technical replicates were performed for the quantification of ACO2 expression level. The asterisks indicate significant differences between control and chemical-treated samples at P <.001 according to t-test. (m) Measurement of ethylene production in wild-type and aco2-1 (ACO2 knockdown mutant) seedlings. Values are expressed as the mean of three biological replicates ± S.E. (n) Growth of wild-type and aco2-1 seedlings under light conditions. The scale bar in N represents 1 cm
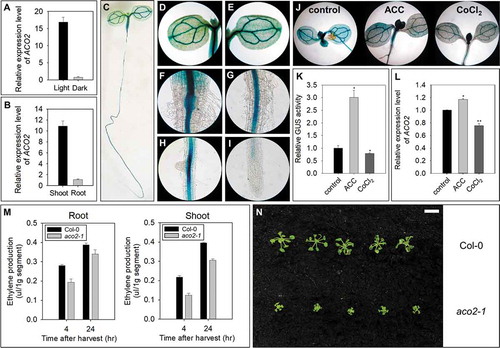
ProACO2::GUS (Supplementary Figure S2) expression and qRT-PCR analysis showed that exogenously applied brassinolide (BL, 10−7 M) reduced the expression of ACO2 (). In a BR-deficient mutant (de-etiolated2, det2), the expression of ACO2 was increased compared with that in wild-type seedlings (). The enhanced ACO2 expression in det2 was decreased with the application of BL, suggesting that BR may negatively regulate the expression of ACO2 in Arabidopsis. In bes1-D (a BES1-dominant mutant), ACO2 expression was reduced compared with that in wild-type seedlings; however, no significant change in ACO2 expression was detected in bzr1-1D (a BZR1-dominant mutant), indicating that BR-suppressed ACO2 expression may be mainly mediated by the BES1 transcription factor via the BR signaling pathway () in Arabidopsis.
Figure 2. Downregulation of ACO2 by BR signaling via BES1 in Arabidopsis seedlings. (a and b) Effect of exogenously applied BL on the expression of ACO2. (c) Comparison of ACO2 expression in wild-type and det2 seedlings. (d) Relative expression of ACO2 in bes1-D and bzr1-1D compared with its expression in wild-type seedlings (En-2 and Col-0 for bes1-D and bzr1-1D, respectively). qRT-PCR was performed with total RNA. UBQ5 was used to normalize the expression level. Two biological replicates along with three technical replicates were performed for the quantification of ACO2 expression level. The asterisks indicate significant differences between control and chemical-treated samples at P <.001 according to t-test. (e) Analysis of cis-elements located in the putative promoter region of ACO2. The boxes indicate E-box sequences in the promotor region, and the location of each E-box is marked with a negative number. (f) ChIP assay of the in vivo association of BES1 or BZR1 with ACO2 promoter sequences. ChIP assay was performed with anti-YFP antibody and chromatin isolated from 35S:YFP-BES1; BES1-YFP (red), 35S:YFP-BZR1 (blue), and non-transgenic wild-type; Col-0 (green) A. thaliana seedlings. Fold enrichment was quantified by qPCR using the primers of flanking regions including cis-regulatory elements: E-boxes or BRRE. SAUR-AC and DWF4 represent the positive control for the binding of BES1-YFP and BZR1-YFP, respectively. CNX5 represents the negative control of the binding of BES1-YFP and BZR1-YFP. Error bars indicate the S.D. of eight replicates (two biological replicates with four technical replicates)
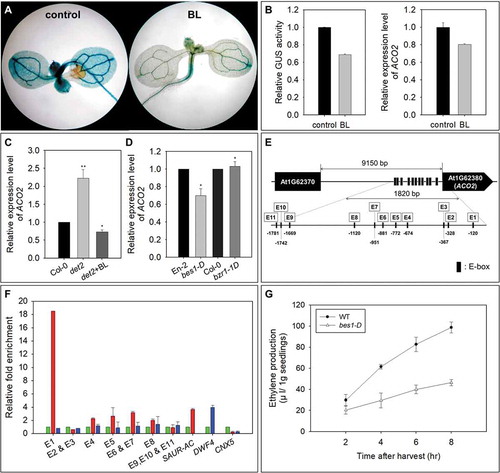
Figure 3. A proposed scheme for the regulation of ACO1, ACO2, and ACO4 expression by BR signaling in the seedlings of Arabidopsis thaliana.
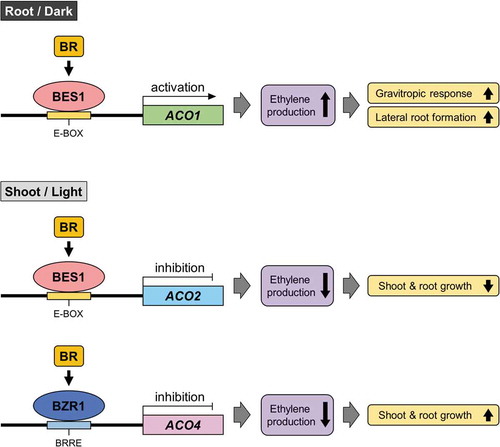
In the putative promoter region (a 1820 bp 5ʹ-intergenic region from the start codon of ACO2 to the 3ʹ-untranslated region of the adjacent gene) of ACO2, 11 E-box motifs (CANNTG) but not BRRE (CGTGTG) motifs were present (). To investigate the interaction between the ACO2 promoter and the BES1 and/or BZR1 protein, MBP-BES1 and MBP-BZR1 were obtained from E. coli using a construct in which MBP was fused to the N-terminal of full-length BES1 and BZR1, and chromatin immunoprecipitation (ChIP) assay was performed. As shown in , there was no meaningful binding of MBP-BZR1 to any E-box motifs. The MBP-BES1 protein was strongly bound to E-box1 and slightly bound to E-box4, E-box5, E-box6/E-box7, and E-box8. The MBP-BES1 protein binding activity to E-box1 was approximately 8 times higher than that to E-box4, E-box5, E-box6/E-box7, and E-box8, suggesting that BES1-regulated ACO2 expression may be mainly mediated by the interaction of BES1 and E-box1. In bes1-D, ethylene production was significantly reduced compared with that in wild-type seedlings (). Therefore, the BES1-mediated downregulation of ACO2 expression may play a role in the maintenance of the homeostatic level of endogenous ethylene for the growth and development of Arabidopsis seedlings.
ACO2 has been reported to be involved in seed germination, where it participates in ethylene production to control endosperm cap weakening and endosperm rupture.Citation27-29 In comparison with wild-type Arabidopsis, aco2-1 showed reduced seed germination (Supplementary Figure S3), demonstrating that ACO2 may play a role in the germination of Arabidopsis seeds. ACO2 is known to be highly expressed in the phloem, especially in companion cells.Citation29 As shown in , GUS activity was strongly detected in the vascular system, particularly in the phloem of ProACO2::GUS transgenic plants. However, GUS activity in companion cells was not detected by microscopic observation in the transgenic plants. ACO2 is also known to be upregulated during flower development.Citation29 In bes1-D, the expression of ACO2 was enhanced compared with that in wild-type seedlings (Supplementary Figure S4), suggesting that the downregulation of ACO2 by BES1 may be important in the vegetative growth but not reproductive growth of A. thaliana.
Our previous and present studies revealed that ACO1 was predominantly expressed in the root tips under dark conditions in Arabidopsis seedlings.Citation9 In contrast, ACO2 and ACO4 were strongly expressed in the shoots under light conditions.Citation30 The dark-to-light transition suppressed ACO1 expression but enhanced ACO2 and ACO4 expression, indicating that the expression of ACO1, ACO2, and ACO4 was tissue-specific and light-regulated in Arabidopsis. Exogenously applied BRs increased ACO1 expression but decreased ACO2 and ACO4 expression. In a BR-deficient mutant (det2), the expression of ACO1 was upregulated, whereas that of ACO2 and ACO4 was downregulated. The expression of ACO1, ACO2, and ACO4 was altered in BR-dominant mutants (bes1-D and bzr1-1D) compared with wild-type seedlings, suggesting that the BR-induced regulation of the expression of the three functional genes in Arabidopsis may be mediated by BES1 and/or BZR1. Promoter analysis for ACO1, ACO2, and ACO4 revealed that 4 E-boxes in ACO1, 11 E-boxes in ACO2, and 4 E-boxes and a BRRE in ACO4 may function as cis-regulatory elements for the binding of BES1 and/or BZR1 in the intergenic region of the genes. Electrophoretic mobility shift assay and/or ChIP assay showed the binding of only BES1 to E-boxes in the promoter region of ACO1 and ACO2 and the binding of only BZR1 to the BRRE in the ACO4 promoter. These findings indicated that the expression of ACO1 (upregulated), ACO2 (downregulated), and ACO4 (downregulated) may be differentially regulated by different BR transcription factors (i.e., BES1 for ACO1 and ACO2 and BZR1 for ACO4) in A. thaliana (). How Arabidopsis achieves this fine regulation and how BES1 and BZR1 coordinately function with each other to control ethylene production remain to be elucidated. Considering that the expression of ACO1, ACO2, and ACO4 is light-regulated, light may be an upstream factor for the fine regulation of ACO expression by BRs in A. thaliana.
Experimental section
Plant materials and growth conditions
A. thaliana Columbia-0 (Col-0) was used as the wild-type ecotype background of all mutants and transgenic plants such as aco2-1 obtained from SALK (SALK_044146), BES1-YFP, BZR1-YFP, and ProACO2::GUS in this study. Seeds were surface-sterilized for 5 min with EtOH-H2O (70:30 v/v), rinsed in distilled H2O, and stratified at 4°C for 2 days. The seeds were planted on 0.5× Murashige and Skoog (MS) medium containing 1% (w/v) sucrose and 0.8% agar medium (Phytagel; Sigma, St. Louis, MO, USA). Plants were grown under light conditions at 22°C for 16 h and dark conditions at 20°C for 8 h in a growth chamber (Vision Scientific, Seoul, Korea).
Total RNA isolation and qRT-PCR
Total RNA was extracted from the seedlings using Total RNA Prep Kit (BIOFACT, Seoul, Korea) containing 1% 2-mercaptoethanol in accordance with the manufacturer’s instructions. The extracted total RNA (5 µg) was reverse-transcribed by M-MLV Reverse Transcriptase (Promega, Madison, WI, USA). PCR was performed using iQ SYBR Green SuperMix and iCycler iQTM Real-time PCR Detection System (Bio-Rad). PCR conditions consisted of denaturation at 95°C for 3 min followed by 40 cycles of denaturation at 95°C for 15 s, annealing at 58°C for 15 s, and elongation at 72°C for 30 s. mRNA levels were quantified using the 2−ΔΔCT method (Livak and Schmittgen, 2001). The expression level of the target gene was normalized to the expression of the housekeeping gene PROTEIN PHOSPHATASE 2A (PP2A). Gene-specific primers are listed in Supplementary Table S1.
Transgenic plants harboring ProACO2::GUS constructs and histochemical analysis of GUS
Genomic DNA fragments of 1820 bp upstream from the translation start codon of ACO2 (At1g62380) were introduced in the pBI121 binary vector containing the GUS (UidA) reporter gene. Constructs were transformed into Col-0 of Arabidopsis using the floral dipping method. Transgenic plants were selected by kanamycin (50 mg/ml). For GUS histochemical analysis, transgenic Arabidopsis seedlings of proACO2::GUS were fixed with 90% (v/v) acetone at 4°C and incubated in a staining solution containing 2 mM 5-bromo-4-chloro-3-indolyl-β-D-GlcUA (X-glcA; Duchefa Biochemie) in 50 mM Na2HPO4 buffer (pH 7.2), 2 mM potassium ferrocyanide, 2 mM potassium ferricyanide, and 0.2% Triton X-100 at 37°C overnight after vacuum infiltration on ice for 20 min. The samples were washed with ethanol-distilled water (70:30 v/v) and observed using a dissecting microscope (Olympus SZ-PT) and a light microscope (Olympus CX21).Citation31
Chromatin immunoprecipitation (ChIP) assay
Wild-type Arabidopsis (Col-0) and transgenic plants expressing BES1-YFP and BZR1-YFP were used for ChIP assay as described by Saleh et al.Citation32 The chromatin-protein complex was isolated from the plant tissue by incubation in cross-linking extraction buffer (0.4 M sucrose, 10 mM Tris-HCl pH 8.0, 1 mM PMSF, 1 mM EDTA, and 1% formaldehyde) under vacuum. Tissues were ground to a fine powder with liquid nitrogen and resuspended in cold nuclei isolation buffer (0.25 M sucrose, 15 mM PIPES pH 6.8, 5 mM MgCl2, 60 mM KCl, 15 mM NaCl, 1 mM CaCl2, 0.9% Triton X-100, 2 µg ml−1 pepstatin A, and 2 µg ml−1 aprotinin). The homogenized slurry was filtered through several layers of Miracloth and centrifuged at 11,000 g for 20 min at 4°C. The supernatant was discarded, and the pellet was resuspended in cold nuclei lysis buffer (50 mM HEPES pH 7.5, 150 mM NaCl, 1 mM EDTA, 1% SDS, 0.1% sodium deoxycholate, 1% Triton X-100, 1 µg ml−1 pepstatin A, and 1 µg ml−1 aprotinin). The resuspended samples (DNA) were sheared into ~500 bp fragments by ultrasonication. ChIP-grade YFP antibody (Abcam, Cambridge, UK) was added to the samples and kept at 4°C for at least 5 h under gentle rotation. The immunocomplexes were precipitated with pre-equilibrated protein A agarose beads (Santa Cruz Biotechnology, Dallas, TX, USA). The eluted DNA was purified using a PCR purification kit (LaboPass, Seoul, Korea) and used as a template for qRT-PCR. Region-specific primer sequences used in ChIP-qPCR are listed in Supplementary Table S2.
Measurement of ethylene production
The seedlings of Arabidopsis were collected in 25 ml silicon-capped vials and mixed with 200 μL of buffer (100 mM MES pH 6.8, 1.5 mM chloramphenicol). The vials were incubated under dark conditions at 27 ± 1°C with gentle shaking (170 rpm). Air samples (1 ml) from these vials were withdrawn with a syringe and injected into a gas chromatograph (HP5890 Series II; Hewlett-Packard, Palo Alto, CA, USA) equipped with a column containing alumina and an 80/100 Porapak Q column. The operating conditions were set as follows: oven temperature, 120°C; injector temperature, 150°C; detector temperature.
Disclosure of potential conflicts of interest
No potential conflicts of interest were disclosed.
Supplemental Material
Download MS Word (22.4 KB)Supplementary material
Supplemental data for this article can be accessed on the publisher’s website.
Additional information
Funding
References
- Bleecker AB, Kende H. Ethylene: a gaseous signal molecule in plants. Annu Rev Cell Dev Biol. 2000;16:1–6. doi:10.1146/annurev.cellbio.16.1.1.
- Yin -C-C, Zhao H, Ma B, Chen S-Y, Zhang J-S. Diverse roles of ethylene in regulating agronomic traits in rice. Front Plant Sci. 2017;8:1676. doi:10.3389/fpls.2017.01676.
- Dubois M, Van den Broeck L, Inzé D. The pivotal role of ethylene in plant growth. Trends Plant Sci. 2018;23:311–323. doi:10.1016/j.tplants.2018.01.003.
- Yang SF, Hoffman NE. Ethylene biosynthesis and its regulation in higher plants. Annu Rev Plant Physiol. 1984;35:155–189. doi:10.1146/annurev.pp.35.060184.001103.
- Kende H. Ethylene biosynthesis. Annu Rev Plant Biol. 1993;44:283–307. doi:10.1146/annurev.pp.44.060193.001435.
- Sato T, Theologis A. Cloning the mRNA encoding 1-aminocyclopropane-1-carboxylate synthase, the key enzyme for ethylene biosynthesis in plants. Proc Nat Acad Sci. 1989;86:6621–6625. doi:10.1073/pnas.86.17.6621.
- Hamilton A, Bouzayen M, Grierson D. Identification of a tomato gene for the ethylene-forming enzyme by expression in yeast. Proc Nat Acad Sci. 1991;88:7434–7437. doi:10.1073/pnas.88.16.7434.
- Spanu P, Reinhardt D, Boller T. Analysis and cloning of the ethylene‐forming enzyme from tomato by functional expression of its mRNA in Xenopus laevis oocytes. Embo J. 1991;10:2007–2013.
- Park CH, Roh J, Youn J-H, Son S-H, Park JH, Kim SY, Kim T-W, Kim S-K. Arabidopsis ACC oxidase 1 coordinated by multiple signals mediates ethylene biosynthesis and is involved in root development. Mol Cells. 2018;41:923.
- Van de Poel B, Van Der Straeten D. 1-aminocyclopropane-1-carboxylic acid (ACC) in plants: more than just the precursor of ethylene! Front Plant Sci. 2014;5:640. doi:10.3389/fpls.2014.00640.
- Wang KL-C, Li H, Ecker JR. Ethylene biosynthesis and signaling networks. Plant Cell. 2002;14:S131–S51. doi:10.1105/tpc.001768.
- Adams D, Yang S. Ethylene biosynthesis: identification of 1-aminocyclopropane-1-carboxylic acid as an intermediate in the conversion of methionine to ethylene. Proc Nat Acad Sci. 1979;76:170–174. doi:10.1073/pnas.76.1.170.
- Sasse JM. Physiological actions of brassinosteroids: an update. J Plant Growth Regul. 2003;22:276–288. doi:10.1007/s00344-003-0062-3.
- Mandava NB. Plant growth-promoting brassinosteroids. Annu Rev Plant Physiol Plant Mol Biol. 1988;39:23–52. doi:10.1146/annurev.pp.39.060188.000323.
- Clouse S, Zurek D. Molecular analysis of brassinolide action in plant growth and development. American Chemical Society; 1991
- Zhu J-Y, Sae-Seaw J, Wang Z-Y. Brassinosteroid signalling. Development. 2013;140:1615–1620. doi:10.1242/dev.060590.
- Li J, Nagpal P, Vitart V, McMorris TC, Chory J. A role for brassinosteroids in light-dependent development of Arabidopsis. Science. 1996;272:398–401. doi:10.1126/science.272.5260.398.
- Mitchell J, Mandava N, Worley J, Plimmer J, Smith M. Brassins—a new family of plant hormones from rape pollen. Nature. 1970;225:1065–1066. doi:10.1038/2251065a0.
- Domagalska MA, Sarnowska E, Nagy F, Davis SJ. Genetic analyses of interactions among gibberellin, abscisic acid, and brassinosteroids in the control of flowering time in Arabidopsis thaliana. PloS One. 2010;5:e14012. doi:10.1371/journal.pone.0014012.
- Joo S, Seo YS, Kim SM, Hong DK, Park KY, Kim WT. Brassinosteroid induction of AtACS4 encoding an auxin‐responsive 1‐aminocyclopropane‐1‐carboxylate synthase 4 in Arabidopsis seedlings. Physiol Plant. 2006;126:592–604.
- Nemhauser JL, Hong F, Chory J. Different plant hormones regulate similar processes through largely nonoverlapping transcriptional responses. Cell. 2006;126:467–475. doi:10.1016/j.cell.2006.05.050.
- Arteca RN, Bachman JM. Light inhibition of brassinosteroid-induced ethylene production. J Plant Physiol. 1987;129:13–18. doi:10.1016/S0176-1617(87)80097-4.
- Hansen M, Chae HS, Kieber JJ. Regulation of ACS protein stability by cytokinin and brassinosteroid. Plant J. 2009;57:606–614. doi:10.1111/j.1365-313X.2008.03711.x.
- Lv B, Tian H, Zhang F, Liu J, Lu S, Bai M, Li C, Ding Z. Brassinosteroids regulate root growth by controlling reactive oxygen species homeostasis and dual effect on ethylene synthesis in Arabidopsis. PLoS Genet. 2018;14:e1007144. doi:10.1371/journal.pgen.1007144.
- Park C-H, Seo C, Park YJ, Youn J-H, Roh J, Moon J, Kim S-K. BES1 directly binds to the promoter of the ACC oxidase 1 gene to regulate gravitropic response in the roots of Arabidopsis thaliana. Plant Signal Behav. 2020;15:1690724. doi:10.1080/15592324.2019.1690724.
- Jiroutova P, Oklestkova J, Strnad M. Crosstalk between brassinosteroids and ethylene during plant growth and under abiotic stress conditions. Int J Mol Sci. 2018;19:3283. doi:10.3390/ijms19103283.
- Linkies A, Müller K, Morris K, Turečková V, Wenk M, Cadman CS, Corbineau F, Strnad M, Lynn JR, Finch-Savage WE, et al. Ethylene interacts with abscisic acid to regulate endosperm rupture during germination: a comparative approach using lepidium sativum and Arabidopsis thaliana. Plant Cell. 2009;21:3803–3822. doi:10.1105/tpc.109.070201.
- Linkies A, Leubner-Metzger G. Beyond gibberellins and abscisic acid: how ethylene and jasmonates control seed germination. Plant Cell Rep. 2012;31:253–270. doi:10.1007/s00299-011-1180-1.
- Houben M, Van de Poel B. 1-Aminocyclopropane-1-carboxylic acid oxidase (ACO): the enzyme that makes the plant hormone ethylene. Front Plant Sci. 2019;10:695. doi:10.3389/fpls.2019.00695.
- Moon J, Park YJ, Son S-H, Roh J, Youn JH, Kim SY, Kim S-K. Brassinosteroids signaling via BZR1 down-regulates expression of ACC oxidase 4 to control growth of Arabidopsis thaliana seedlings. Plant Signal Behav. 2020;15:1734333.
- Jefferson RA, Kavanagh TA, Bevan MW. GUS fusions: beta‐glucuronidase as a sensitive and versatile gene fusion marker in higher plants. Embo J. 1987;6:3901–3907. doi:10.1002/j.1460-2075.1987.tb02730.x.
- Saleh A, Alvarez-Venegas R, Avramova Z. An efficient chromatin immunoprecipitation (ChIP) protocol for studying histone modifications in Arabidopsis plants. Nat Protoc. 2008;3:1018. doi:10.1038/nprot.2008.66.