ABSTRACT
Atractylodes lancea is a type of typical traditional Chinese medicinal (TCM) herb that is economically important in China. The traditional planting method of A. lancea is to plant in situ continuously for many years, which often leads to impediments for its growth and development and soil-borne diseases. The root-associated microbiome is believed to play an important role in plant resistance and the quality of products from the plant. This study aims to reveal detailed changes in the populations of rhizosphere microorganisms, and providing theoretical guidance for the prevention and control of soil-borne diseases in A. lancea. A high-throughput sequencing approach was utilized to illustrate changes in the microbial community from different planting years. Results and conclusions: The results show that the diversity and composition of the root-associated microbiome was significantly impacted by the consecutive monoculture of A. lancea. At the level of the comparisons of the phyla, Bacteroidetes, Proteobacteria, Ascomycota, and Basidiomycota declined significantly. In contrast, the relative abundance of Actinobacteria, Acidobacteria, and Mortierellomycota distinctly increased. Comparisons at the genus level indicated that Sphingomonas, Flavobacterium, Pseudomonas, Pedobacter, and Tausonia decreased significantly, whereas Mortierella, Cylindrocarpon, Dactylonectria, and Mucor distinctly increased. In conclusion, this study helps to develop an understanding of the impediments involved in the consecutive monoculture of A. lancea.
1. Introduction
Atractylodes lancea is a type of traditional medicinal plant and is the primary ingredient of many popular Chinese medicines, and can dispel wind and drive out the cold, invigorate the dryness and dampness of the spleen, and brighten eyes, which are used to treat rheumatic diseases, digestive disorders, night blindness, and influenza.1 It is a perennial plant that is usually continuously cultivated for three or more years for production. However, the replantation of A. lancea usually fails due to the disease and insect pests that are always influenced by continuous planting and can cause the plant to grow poorly or even die. Citation1–2 Consecutive monoculture leads to a substantial decline in both the yield and quality of A. lancea () and severe soil-borne disease. Citation3 The diversity and composition of the soil microbial communities are critical to maintain soil health and quality, which are significantly affected by both the length of monoculture and the plant growth stage. Citation4 Numerous medicinal plants are prone to develop serious disorders from consecutive monoculture, approximately 70% of the medicinal species whose tuberous roots are used in medicine have various degrees of problems from consecutive monoculture, including Pseudostellaria heterophylla and Rehmannia glutinosa. Citation5,Citation6 The rhizosphere contains abundant microorganisms, which has been reported to be the second genome of the plant, and are crucial for plant health and performance. Citation7–9 Previous research on the problems of consecutive monoculture has primarily focused on the deficiency of soil nutrients and the autotoxicity of allelochemicals in root exudates, Citation10 and there are increasing numbers of studies on the problems in the growth of Chinese medicinal materials. Yang et al. Citation11 found that the bacterial and fungal populations varied dramatically in rhizosphere soil, and the microbial balance was severely altered during the growth and consecutive monoculture of Rehmannia glutinosa. Nguyen et al. Citation12 found that the bacterial richness in the soil decreased with the years of cultivation of Panax ginseng. Li et al. Citation13 found that the long-term consecutive monoculture of black pepper leads to variations in the soil microbial community membership and structure that inhibited the growth of the plant. Although some reports demonstrated that the plant-microbe community interactions play crucial roles in crop health, Citation8,Citation14 little is known about the changes in the microbial community structure and abundance in the rhizosphere of A. lancea when grown under a monoculture regime.
Therefore, it is essential to study the relationship between A. lancea and the rhizosphere microbiome. In our study, we examined the diversity and composition of bacterial and fungal communities under continuous cropping of A. lancea, which should provide a new understanding of the manner in which the root microbiome can enhance the production and sustainability of A. lancea.
2. Material and methods
2.1. Soil sampling
The experiment was conducted at Feng City, Shaanxi Province, China (106°50ʹ21.42”E, 34°11ʹ48.43”N). Rhizosphere soil samples of A. lancea from different growth stages included five treatments, one-year cultivation (FH1), two-year consecutive monoculture (FH2), three-year consecutive monoculture (FH3), control with no A. lancea cultivation (FHCK1), and one year after the post-harvest soil samples (FHCK2), respectively. Each sample was collected using the five-point sampling method and three biological replicates were sampled for each treatment. All the samples were taken by carefully removing the plants from the soil around the sampling locations, and the rhizosphere soil adhering to the roots was separated by hand and enclosed within sterile gloves. We collected samples that passed through a 2-mm mesh and stored them at −80°C until they were subjected to further processing.
2.2. DNA extraction and PCR amplification
A TIANamp Soil DNA Kit (Tiangen Biochemical Technology Co., Ltd., Beijing, China) was used to extract the total DNA of soil samples according to the manufacturer’s instructions. Three repetitions were conducted for every treatment. The DNA concentration and purity were monitored on 1.0% agarose gels. The DNA was diluted to 1 ng/µl using sterile water. The total DNA of the repeated samples was stored at −20°C for later use. PCR was then performed using specific barcode primers, and the reactions were conducted using Phusion® High-Fidelity PCR Master Mix (New England Biolabs, Ipswich, MA, USA). The 30 µL reaction system consisted of the following: 15 μl of PCR Mix (2× Phusion® High-Fidelity PCR Master Mix with GC Buffer), 2 μl of each primer (2 μM), 10 μl of gDNA (1 ng/μl), and 3 μl of ddH2O. Thermal cycling consisted of an initial denaturation at 98°C for 1 min, followed by 30 cycles of denaturation at 98°C for 10 s, annealing at 50°C for 30 s, and elongation at 72°C for 30 s with a final treatment of 72°C for 5 min. The bacterial 16S rRNA primer sequences used were 16SV4: 515 F-806 R, and the fungal 18S rRNA primer sequences used were ITS1: ITS1F-ITS2 and ITS2: ITS2-3 F-ITS2-4 R.
2.3. PCR purification and high-throughput sequencing
The PCR products were monitored on a 2.0% agarose gel, and the concentration of DNA was determined using a Nanodrop 2000 C spectrophotometer (ThermoFisher Scientific, USA). A 2.0% agarose gel and 1× TAE were used to purify the PCR products, and the target bands were cut and recovered for further analysis. The PCR products were mixed at an equal ratio and purified with a GeneJET Gel Extraction Kit (ThermoFisher Scientific).
An Ion Plus Fragment Library Kit 48 Reactions Preparation Kit (ThermoFisher Scientific) was used to construct cDNA libraries of the microbial communities in the soil samples. The library was constructed after the samples had passed Qubit quantification and library detection and was sequenced on an Ion S5TMXL System (ThermoFisher Scientific) by the Novogene Company (Beijing, China).
2.4. Bioinformatics and statistical analysis
To improve the reliability of the data processing, sequence reads were assigned to each sample based on their unique barcode and truncated by removing the barcode and primer sequences. Paired-end reads from the original DNA fragments were merged using FLASH. Through quality filtering and chimera removal, the retained sequences called effective tags were used to perform OTU cluster and species annotation. The effective tags were performed to cluster using UPARSE software (UPARSE v7.0.1001, http://drive5.com/uparse/). Sequences with ≥97% similarity were assigned to the same OTUs. According to its algorithm principle, the sequence with the highest frequency in OTUs was screened as the representative sequence of each OTU for further annotation, which was based on a Mothur algorithm and SSUrRNA database of SILVA132 (http://www.arb-silva.de/) to annotate the taxonomic information (with the threshold set to 0.8₋1). The abundances of OTUs were normalized using a standard of sequence number corresponding to the sample with the least sequences. Moreover, the community composition of each treatment was analyzed from the five classification levels of kingdom, phylum, class, order, family, genus, and species. Subsequent analysis of alpha diversity and beta diversity were all performed basing on this output normalized data. Alpha diversity was applied to analyze the species complexity using six indices, observed-species, community richness indices (Chao1 and ACE) and diversity indices (Shannon’s and Simpson’s). Beta diversity analyses were used to evaluate the differences in the complexity of the species between treatments, including principal coordinate analysis (PCoA) and unweighted pair-group method with arithmetic means (UPGMA) clustering.
3. Results
3.1. Alpha diversity indices of the microbial community
A rarefaction curves analysis showed that the sequences from 15 soil samples tended to plateau at 35,000 and 50,000 sequences for bacteria ()) and fungi ()), respectively. Venn diagrams were created by using OTUs and showed the similarity of microbial communities in all five treatments. A total of 6,516 and 4,691 OTUs for bacteria ()) and fungi ()), respectively, were obtained in the rhizosphere soil samples, and they enabled assignment to the phylum, class, order, family, genus, and species levels, respectively. The results showed 48 phyla, 516 genera, and 403 species of bacteria, and 49 phyla, 485 genera, and 544 species of fungi were identified, respectively (Table S1).
Figure 2. Rarefaction curves of microbial communities based on observed operational taxonomic units (OTUs) at the 97% similarity cutoff level for individual samples. FH1, FH2, FH3, FHCK1, and FHCK2 represent the rhizosphere soil from the one-year cultured, two-year consecutively monocultured, three-year consecutively monocultured, control with no Atractylodes lancea cultivation, and one year after the harvest of the rhizosphere soil plots, respectively. a – Bacteria; b – Fungi
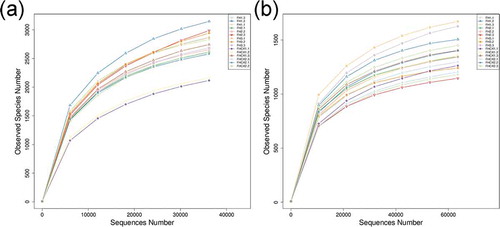
Figure 3. Venn diagrams created using OTUs and showing the number of OTUs unique to a single treatment or shared by two, three, four, or all five treatments. Numbers indicate the number of OTUs. FH1, FH2, FH3, FHCK1, and FHCK2 represent the rhizosphere soil from the one-year cultured, two-year consecutively monocultured, three-year consecutively monocultured, control with no Atractylodes lancea cultivation, and one year after the harvest of the rhizosphere soil plots, respectively. a – Bacteria; b – Fungi
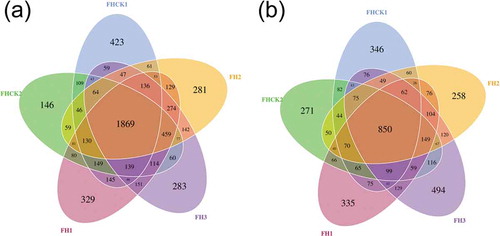
The microbial community richness and diversity indices are listed in . The FHCK1 had the lowest Shannon and Chao1 indices of 8.83 and 3,996 of the bacterial community, respectively, followed by the FH1 and FHCK2. In contrast, FH3 had the high Shannon and Chao1 indices of 9.72 and 4,676 of the bacterial community, respectively. Although the Shannon and Chao1 indices did not reveal a significant difference between adjacent years, it is known that the soil bacterial richness and diversity increased with the planting duration in the rhizosphere. Citation15,Citation16 However, the Shannon and Chao1 indices in all the treatments indicated a lower diversity and richness for the fungal compared with the bacterial communities. The Shannon and Chao1 indices also revealed a similar trend as the bacteria in rhizosphere soils as the planting duration increased, in particular, when the FH3 was compared with that of the FHCK1.
Table 1. Bacterial and fungal calculations of the observed species, richness, and diversity in different soil treatments
3.2. Beta diversity indices microbial community
In this study, principal coordinate analysis (PCoA) at the phylum level showed distinct differences in the microbial community structure among bacteria and fungi in the different treatments. The first two principal components (PC1 and PC2) of PCoA in the bacteria accounted for 48.38% and 12.78% of the total variation, respectively ()), but accounted for 30.48% and 14.47% of the total variation in the fungi, respectively ()). Furthermore, a PCoA analysis showed that the fungal community was more dispersed than the bacterial community with different planting years, and it could be clearly observed that the bacterial and fungal structures changed significantly after the planting of A. lancea compared with the FHCK1. The FHCK1 was quite different from those of the FH1, FH2, FH3, and FHCK2 (), the results of UPGMA cluster analysis of the bacterial community ()) also reflect this phenomenon well. However, the fungi community obvious differences in those from bacteria ().
Figure 4. Principal coordinate analysis (PCoA) (a, c) and hierarchical clustering (b, d) of microbial communities based on an unweighted pair-group method with arithmetic mean (UPGMA) clustering analyses at the phylum level for five different soil treatments. FH1, FH2, FH3, FHCK1, and FHCK2 represent the rhizosphere soil from the one-year cultured, two-year consecutively monocultured, three-year consecutively monocultured, control with no Atractylodes lancea cultivation, and one year after the harvest of the rhizosphere soil plots, respectively. a, b – Bacteria; c, d – Fungi
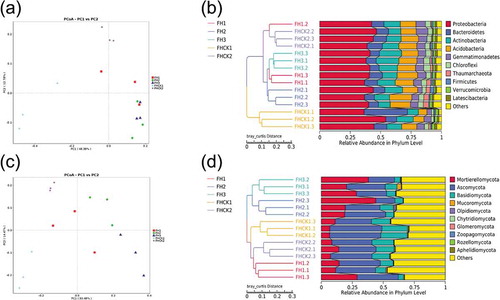
3.3. Shifts in the soil microbial community structure under consecutive monoculture
The successive cropping of A. lancea caused highly significant changes in the microbial composition and structure in the rhizosphere. The results of the relative abundance comparison at the phylum level ()) showed that Proteobacteria, Bacteroidetes, Actinobacteria, Acidobacteria, and Gemmatimonadetes were the dominant bacteria and accounted for 45.15%, 18.32%, 11.66%, 9.37%, and 5.36% of the bacterial microbial community, respectively. Nevertheless, after three years of continuous planting, the abundance of the dominant bacteria obviously changed and accounted for 40.25%, 7.83%, 17.35%, 14.35%, and 6.26% of the totals, respectively (). Although both Proteobacteria and Bacteroidetes were the dominant microbiome, their abundance decreased by 4.9% and 10.49%, respectively, after the planting of A. lancea, in contrast to the abundance of Actinobacteria and Acidobacteria, which increased by 5.69% and 4.98%, respectively. The fungi showed similar changes when compared with the bacteria ()). Ascomycota, Basidiomycota, and Mortierellomycota were the dominant microbiome, accounting for 36.40%, 18.94%, and 8.29%, respectively, and the abundance of the fungal community also changed significantly. After three years of planting, the abundances of the dominant microbiome changed to 24.79%, 6.81%, and 28.39%, respectively (). In addition, Ascomycota and Basidiomycota were the dominant microbiome and decreased by 11.61% and 12.13%, respectively, with the cultivation of A. lancea. However, the abundances of Mortierellomycota and Mucoromycota increased by 20.1% and 0.66%, respectively. Furthermore, it was notable that Bacteroidetes, Actinobacteria, Ascomycota, and Mortierellomycota showed a consistent trend with their abundance increasing or decreasing with the planting time.
Table 2. Abundance of the primary bacteria and fungi at the phyla or genera level in the treatment of different growth years
Figure 5. Relative abundances of the top 10 microbial phylum levels in five different soil treatments. FH1, FH2, FH3, FHCK1, and FHCK2 represent the rhizosphere soil from the one-year cultured, two-year consecutively monocultured, three-year consecutively monocultured, control with no Atractylodes lancea cultivation, and one year after the harvest of the rhizosphere soil plots, respectively. a – Bacteria; b – Fungi
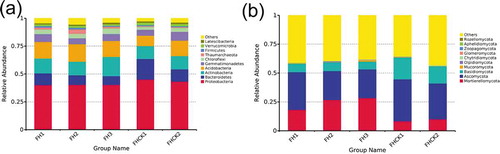
At the genus level, 35 of the most abundant genera in five different treatments were analyzed by a heatmap. The abundance of bacterial communities showed that Sphingomonas, Flavobacterium, Pedobacter, and Pseudomonas had higher abundances, and the dominant bacteria in the FHCK1 accounted for 7.72%, 6.04%, 3.82%, and 3.46%, respectively. However, the dominant microbiome had undergone obvious changes with the cultivation of A. lancea, and Sphingomonas, Acidobacteria, and Pseudomonas had become the predominant microbiome in the FHCK3, which accounted for 3.52%, 3.30%, and 3.06% of the total number of bacteria, respectively ()). Furthermore, some predominant genera consistently changed. In particular, the relative abundance of Sphingomonas gradually decreased with cropping years. In addition, the relative abundances of Flavobacterium, Pseudomonas, Pedobacter, and Lelliottia were significantly reduced, but the relative abundances of Blastococcus and Acidobacteria behaved in an opposite manner. Compared with the bacterial community, the fungal community had similar changes at the genus level ()). Plectosphaerella, Fusarium, Alternaria, and Solicoccozyma were the predominant microbiome, which accounted for 7.40%, 6.59%, 3.36%, and 3.19% of the total in the FHCK1, respectively, but compared with cropping for three years, Mortierella, Solicoccozyma, Fusarium, and Chaetomium had become the predominant microbiome, which accounted for 21.69%, 4.32%, 4.08%, and 2.26% of the total, respectively. Moreover, the relative abundances of Cylindrocarpon, Dactylonectria, Acremonium, and Mucor increased with the cropping years ().
Figure 6. Heatmap analysis of the top 35 most abundant genera in five different treatments. FH1, FH2, FH3, FHCK1, and FHCK2 represent the rhizosphere soil from the one-year cultured, two-year consecutively monocultured, three-year consecutively monocultured, control with no Atractylodes lancea cultivation, and one year after the harvest of the rhizosphere soil plots, respectively. a – Bacteria; b – Fungi
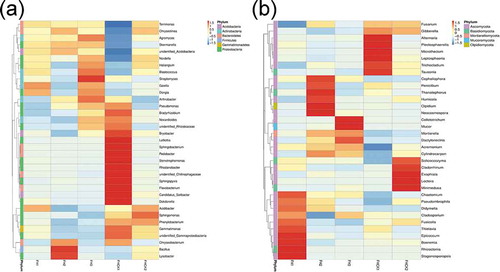
4. Discussion
Consecutive monoculture problems are a common phenomenon in nature, and the rhizosphere of plants contains a large number of microbial communities, which play an important role in the growth and development of plants and has been designated the second genome of plants. Citation17,Citation18 This phenomenon is particularly common in the cultivation of Chinese medicinal materials because of the limitation of the growth years. Citation4,Citation5,Citation12 High-throughput sequencing is an effective method to study the structure and composition of plant rhizosphere microbial community, which is widely applied to the interaction between a variety of medicinal plants and microorganisms. Citation2,Citation11 However, it is the first time to use this method to analyze the changes of the rhizosphere microbial communities of A. lancea in different planting years. Through the analysis of sequencing results, it was found that there were a large number of rhizosphere microbial (Table S1), and the relationship between plants and microorganisms was complex. Citation19,Citation20 Moreover, the result is also supported by Venn Diagrams ().
Xiong et al. Citation21 found that soil microbial richness and diversity index including the Chao1, ACE and Shannon index increased significantly after monoculture of vanilla (Vanilla planifolia). This change of microbial community was also confirmed in the continuous cropping soil of peaches. Citation22 Our results showed the similar changes (), the Chao1, ACE and Shannon indices of the microbial community in other treatments were higher than those in the FHCK1, which indicated that the cultivation of A. lancea can promote the richness of rhizosphere microorganisms. However, this growth trend weakens in the bacteria community with the cultivation years, while that of fungi increased. Interestingly, Dong et al. Citation2 studied the continuous cropping of American ginseng also revealed that a decline in bacterial diversity and an increase in fungal diversity. Previous studies have revealed that plants can shape their rhizospheric microbiomes through root exudates. The total flavones, total phenolics, and total saponins released from Solidago canadensis accumulate in soil, and these secondary metabolites negatively correlated with disease severity of tomato, suggesting that these secondary metabolites contribute to the allelopathic effects of S. canadensis on the soilborne pathogen. Citation23 Haichar et al. Citation24 utilized stable isotope probing (SIP) to determine the bacterial communities that were assimilating each carbon source in the rhizosphere of wheat, maize, rape, and barrel clover (Medicago truncatula) and found that the root exudates significantly shaped the rhizosphere bacterial community structure. We speculated root exudates may be related to the continuous cropping of A. lancea. The above studies also provide insights for us to further study whether the rhizosphere exudates of A. lancea rhizome participate in the composition of rhizosphere microbial community. Although the richness of bacteria and fungi has changed, fungi seemed to suggest more complex changes ()). Li et al. Citation25 suggest that the accumulations of fungal pathogen loads at the expense of plant-beneficial fungi in the soil appear likely explanations for yield declines as a consequence of consecutive peanut cultivation, which indicated that the fungal community was closely related to the cultivation of A. lancea. In addition, the consecutive monoculture of Rehmannia glutinosa revealed that the fungal communities in both rhizosphere and bulk soils was restructured, which was correlated with the time of continuous cropping. Citation26 We speculated that compared with the diversity of the bacteria, short-term planting has less impact on the fungal diversity. Therefore, after A. lancea was harvested, the fungal community of the FHCK2 was the same as that of FH1.
In this study, we also found that the species abundance of the microbial community changed significantly at the phylum or genus level of classification (). At the phylum level, bacteria and fungi showed similar changes. Some dominant microbiome had highly significant decreases or increases (). The relationship between rhizosphere microorganisms and plants is complex, and microorganisms are classified as beneficial, deleterious, or neutral according to their effects on the host. Citation27 The additional analysis at the genus level indicated that Sphingomonas, Flavobacterium, Pedobacter, and Pseudomonas were the dominant bacterial microbiome ()), but they displayed a lower abundance in the other treatments than FHCK1. Studies have shown that isolated Flavobacterium can produce IAA, and IAA is known to stimulate responses, such as cell elongation, and long-term responses (e.g., cell division and differentiation) in plants. Citation28 In other research, the Flavobacterium was found to produce IAA, and the species are also considered to be plant growth promoting rhizobacteria. Citation29,Citation30 Previous studies have shown that the bacterial endophyte Pseudomonas fluorescens ALEB7B could release several nitrogenous volatiles and carbohydrates, which were preferentially used by the host plant to induce metabolic flux changes and significantly promote the growth of plants, production of volatile oils, and the accumulation of photosynthates in A. lancea. Citation31,Citation32 Xiong et al. Citation33 found that long-term consecutive monoculture of black pepper (Piper nigrum L.) led to a significant decline in soil bacterial abundance, especially in the Pseudomonas spp., this is consistent with our results. In addition, most of them showed a decrease in abundance, including Pedobacter, Bacillus, and Stenotrophomonas. Interestingly, the members of Bacillus have been reported to be used extensively as potential antagonistic agents against various plant pathogens by their ability to induce systemic resistance against pathogens. Citation34,Citation35 It was not difficult to find that the abundance of Bacillus decreased with the increase in the planting years. The reduction of these beneficial microorganisms in the rhizosphere soil may have removed the restrictions to the rapid growth of some harmful microorganisms, which subsequently led to the occurrence of some diseases and directly or indirectly affected the health of plants. Citation8 The research on this aspect merits further verification. In contrast, some microorganisms also showed signs of increase. Acetobacter, Arthrobacter, Blastococcus, and Streptomyces increased to different degrees with the cultivation of A. lancea (). Li et al. Citation36 showed that strain ZZ21 of Arthrobacter was a plant-beneficial form found in the rhizosphere. The production of the phytohormone indole-3-aectic acid (IAA) by ZZ21 has the ability to promote plant growth and remediate fluoranthene-contaminated soil. Further analysis found that many Streptomyces strains isolated from soils, rhizospheres, and plant internal tissue of a wide variety of plant species limited the growth of various bacterial and fungal plant pathogens and functioned as effective biocontrol agents. Citation37,Citation38 Several substances produced by beneficial microorganisms were related to pathogen control and the indirect promotion of growth in many plants, such as siderophores and antibiotics. Citation34 Previous studies have shown that only 1 ~ 2% of bacteria can promote plant growth, which demonstrates that plants respond to pathogen attack by recruiting specific beneficial microorganisms. Citation39 It was proposed that the major changes in the composition of the microbiome were the result of the plant-specific selection of microorganisms, while the inhibition was the result of changes in the activity of pathogenicity of existing microorganisms. Citation8 Therefore, the increase in the populations of Arthrobacter and Streptomyces might be the result of rhizosphere recruitment by A. lancea. Compared with the bacterial community, the fungal dominant microbiome did not exhibit regular changes at the genus level ()), Fusarium, Tausonia, and Alternaria exhibited obvious decreases in abundance. In previous studies, Fusarium and Alternaria were considered to be fungi that are associated with disease, which were closely related to the soil-borne disease caused by consecutive monoculture. Citation40 The soil-inhabiting fungus Fusarium oxysporum was ranked one of the top 10 fungal pathogens in molecular plant pathology. Citation41 However, our results showed a different change that we hypothesized to be related to the competition between rhizosphere microorganisms and the planting time in contrast to the opposite trend of Mortierella (). Similarly to the bacterial community, the abundances of the other fungal genera, particularly Cylindrocarpon, Dactylonectria, Acremonium, and Cladorrhinum, also exhibited obvious increases or decreases during different years of cultivation. It is notable that Cylindrocarpon is a deleterious fungus and was isolated from the rhizosphere of ginseng. Citation42 In addition, Carlucci et al. Citation43 showed that the Dactylonectria spp. had been associated with black foot disease of grapevine worldwide. Moreover, the analysis of the data showed that the abundance of fungi increased with the increase of planting time, and they might be potential pathogens. Therefore, we hypothesized that the consecutive monoculture obstacles of A. lancea are closely related to these deleterious fungi.
A growing number of evidences has shown that the change of microbial community structure and composition is an important reason to affecting the cultivation of medicinal plants. Citation44,Citation45 While the soil microbial community exerted a dynamic influence on the state of plant growth through its participation in the synthesis of secondary metabolites or pathogenic activity, it also reshaped by the plants. Citation46 Doornbos et al. Citation47 reported that plants can determine the composition of the root microbiome by their active secretion of root exudates and rhizodeposition. A loss of carbon-containing metabolites from the roots into the soil matrix as a result of rhizodeposition can specifically stimulate or repress members of the microbial community and further influence soil microbial communities. Citation48 The studies described above have fully illustrated the complexity of the relationship between plants and rhizosphere microorganisms. A growing number of studies have shown that the consecutive monoculture impediments might be associated with the succession of rhizosphere microbial community and the development of soil-borne diseases under monoculture. Citation4,Citation5,Citation49 Our results demonstrated that the consecutive monoculture of A. lancea altered the structure and composition of microbial communities in the rhizosphere. Furthermore, to our knowledge, this is the first report on changes of the microbial community in response to the consecutive culture of A. lancea. The reduction in the abundance of beneficial microbes such as Flavobacterium, Pseudomonas, Bacillus and the increment of deleterious microbes such as Fusarium, Alternaria, Arthrobacter, Dactylonectria will provide us with a way to break down the continuous cropping barrier. Wu et al. Citation50 found the application of bio-organic fertilizer MT could significantly increase the bacterial community diversity and restructure microbial community with relatively fewer pathogenic Fusarium oxysporum and more beneficial Burkholderia spp. The application of novel bio-organic fertilizer could effectively suppress Fusarium wilt by enriching the antagonistic bacteria and enhancing the bacterial diversity. Qiu et al. Citation51 indicated that a bio-organic fertilizer which was a combination of manure compost with antagonistic microorganisms (Bacillus sp., Paenibacillus sp., etc.). Therefore, based on our research, we can increase some beneficial microorganisms through some biological fertilizers or regulators, reduce deleterious microorganisms to artificially regulate the changes of the rhizosphere microorganisms of A. lancea. Our results provide a promising strategy for the control of consecutive monoculture barriers and increase both the quality and production of A. lancea culture.
In conclusion, the consecutive monoculture of A. lancea could alter the microbial communities in the rhizosphere. However, more research is needed to verify the relationship between the rhizosphere and the pathogenic and beneficial microorganisms and the mechanism of the change in microbial communities. The isolation of specific microorganisms (i.e., Flavobacterium spp. and Dactylonectria spp.) and their relationships with A. lancea may provide us with more evidence to explain the phenomenon described above.
Disclosure of Potential Conflicts of Interest
No potential conflicts of interest were disclosed.
Supplemental Material
Download MS Word (14.9 KB)Supplementary material
Supplemental data for this article can be accessed on the publisher’s website.
Additional information
Funding
References
- Wang HX , Liu CM , Liu Q , Gao K. Three types of sesquiterpenes from rhizomes of Atractylodes lancea . Phytochemistry. 2008;69:1–9.
- Dong L, Xu J, Zhang L, Yang J, Liao B, Li X, Chen S. High-throughput sequencing technology reveals that continuous cropping of American ginseng results in changes in the microbial community in arable soil. Chin Med-UK. 2017;12:18.
- Bennett AJ , Bending GD , Chandler D , Hilton S , Mills P . Meeting the demand for crop production: the challenge of yield decline in crops grown in short rotations. Biol Rev Camb Philos Soc. 2012;87:52–71.
- Wu L , Wu H , Chen J , Wang J , Lin W . Microbial community structure and its temporal changes in Rehmannia glutinosa rhizospheric soils monocultured for different years. Eur J Soil Biol. 2016;72:1–5.
- Zhao Y-P , Lin S , Chu L , Gao J , Azeem S , Lin W . Insight into structure dynamics of soil microbiota mediated by the richness of replanted Pseudostellaria heterophylla . Sci Rep-UK. 2016;6:26175.
- Wu L, Li Z, Li J, Khan MA, Huang W, Zhang Z, Lin W. Assessment of shifts in microbial community structure and catabolic diversity in response to Rehmannia glutinosa monoculture. Appl Soil Ecol. 2013;67:1-9.
- Mendes R, Kruijt M, de Bruijn I, Dekkers E, van der Voort M, Schneider JH, Piceno YM, DeSantis TZ, Andersen GL, Bakker PA, Raaijmakers JM. Deciphering the rhizosphere microbiome for disease-suppressive bacteria. Science. 2011;332:1097.
- Berendsen RL , Pieterse CMJ , Bakker PAHM . The rhizosphere microbiome and plant health. Trends Plant Sci. 2012;17:478–486.
- Zhang X, Liu X, Zhong C, Cao Z, Liu F, Chen L, Liu S, Hu Y. Soil microbial community response to pyrene at the presence of Scirpus triqueter. Eur J Soil Biol. 2012;50:44-50.
- Huang LF, Song LX, Xia XJ, Mao WH, Shi K, Zhou YH, Yu JQ. Plant-soil feedbacks and soil sickness: from mechanisms to application in agriculture. J Chem Ecol. 2013;39:232-42.
- Yang Q , Wang R , Xu Y , Kang C , Miao Y , Li M . Dynamic change of the rhizosphere microbial community in response to growth stages of consecutively monocultured Rehmanniae glutinosa . Biologia. 2016;71:1320–1329.
- Nguyen NL, Kim YJ, Hoang VA, Subramaniyam S, Kang JP, Kang CH, Yang DC. Bacterial diversity and community structure in Korean ginseng field soil are shifted by cultivation time. PloS ONE. 2016;11:e0155055.
- Li Z , Zu C , Wang C , Yang J , Yu H , Wu H . Different responses of rhizosphere and non-rhizosphere soil microbial communities to consecutive Piper nigrum monoculture. Sci Rep-UK. 2016;6:35825.
- Jiang J, Song Z, Yang X, Mao Z, Nie X, Guo H, Peng X. Microbial community analysis of apple rhizosphere round Bohai Gulf. Sci Rep-UK. 2017;7:8918.
- Wu LK , Chen J , Xiao ZG , Zhu XC , Wang JY , Wu HM , Wu YH , Zhang ZY , Lin WX . Barcoded pyrosequencing reveals a shift in the bacterial community in the rhizosphere and rhizoplane of Rehmannia glutinosa under consecutive monoculture. Int J Mol Sci. 2018;19:850.
- Edwards J , Johnson C , Santos-Medellín C , Lurie E , Podishetty NK , Bhatnagar S , Eisen JA , Sundaresan V . Structure, variation, and assembly of the root-associated microbiomes of rice. Proc Natl Acad Sci USA. 2015;112:E911–E920.
- Berg G , Köberl M , Rybakova D , Müller H , Grosch R , Smalla K . Plant microbial diversity is suggested as the key to future biocontrol and health trends. FEMS Microbiol Ecol. 2017:93. doi:10.1093/femsec/fix050.
- Raaijmakers JM , Paulitz TC , Steinberg C , Alabouvette C , Moënne-Loccoz Y . The rhizosphere: a playground and battlefield for soilborne pathogens and beneficial microorganisms. Plant Soil. 2008;321:341–361.
- Bainard LD , Koch AM , Gordon AM , Klironomos JN . Growth response of crops to soil microbial communities from conventional monocropping and tree-based intercropping systems. Plant Soil. 2013;363:345–356.
- Buée M, Reich M, Murat C, Morin E, Nilsson RH, Uroz S, Martin F. 454 Pyrosequencing analyses of forest soils reveal an unexpectedly high fungal diversity. New Phytol. 2009;184:449-56.
- Xiong W, Zhao Q, Zhao J, Xun W, Li R, Zhang R, Wu H, Shen Q. Different continuous cropping spans significantly affect microbial community membership and structure in a vanilla-grown soil as revealed by deep pyrosequencing. Microbial Ecol. 2015;70; 209–218.
- Benizri E , Piutti S , Verger S , Pagès L , Vercambre G , Poessel JL . Replant diseases: bacterial community structure and diversity in peach rhizosphere as determined by metabolic and genetic fingerprinting. Soil Biol Biochem. 2005;37:1738–1746.
- Zhang S , Zhu W , Wang B , Tang J , Chen X . Secondary metabolites from the invasive Solidago canadensis L. accumulation in soil and contribution to inhibition of soil pathogen Pythium ultimum. Appl Soil Ecol. 2011;48:280–286.
- Haichar FeZ, Marol C, Berge O, Rangel-Castro JI, Prosser JI, Balesdent J, Heulin T, Achouak W. Plant host habitat and root exudates shape soil bacterial community structure. ISME J. 2008;2:1221-30.
- Li XG , Ding CF , Zhang TL , Wang XX . Fungal pathogen accumulation at the expense of plant-beneficial fungi as a consequence of consecutive peanut monoculturing. Soil Biol Biochem. 2014;72:11–18.
- Wu L , Chen J , Khan MU , Wang J , Wu H , Xiao Z , Zhang Z , Lin W . Rhizosphere fungal community dynamics associated with Rehmannia glutinosa replant disease in a consecutive monoculture regime. Phytopathology. 2018;108:1493–1500.
- Benizri E , Baudoin E , Guckert A . Root colonization by inoculated plant growth-promoting rhizobacteria. Biocontrol Sci Technol. 2001;11:557–574.
- Soltani AA , Khavazi K , Rahmani H , Omidvari M , Abbaszadeh Dahaji P , Hamed M . Plant growth promoting characteristics in some Flavobacterium spp. Isolated from soils of Iran. J Agric Sci Cambridge. 2010;2:106–115.
- Asghar HN , Zahir ZA , Arshad M . Screening rhizobacteria for improving the growth, yield, and oil content of canola (Brassica napus L.). Aust J Agric Res. 2004;55:187–194.
- Cattelan AJ , Hartel PG , Fuhrmann JJ . Screening for plant growth–promoting rhizobacteria to promote early soybean growth. Soil Sci Soc Am J. 1999;63:1670–1680.
- Zhou JY , Sun K , Chen F , Yuan J , Li X , Dai CC . Endophytic Pseudomonas induces metabolic flux changes that enhance medicinal sesquiterpenoid accumulation in Atractylodes lancea . Plant Physiol Bioch. 2018;130:473–481.
- Zhou JY , Li X , Zheng JY , Dai CC . Volatiles released by endophytic Pseudomonas fluorescens promoting the growth and volatile oil accumulation in Atractylodes lancea . Plant Physiol Bioch. 2016;101:132–140.
- Xiong W, Li Z, Liu H, Xue C, Zhang R, Wu H, Li R, Shen Q. The effect of long-term continuous cropping of black pepper on soil bacterial communities as determined by 454 pyrosequencing. PLoS ONE. 2015;10:e0136946.
- Beneduzi A , Ambrosini A , Passaglia LMP . Plant growth-promoting rhizobacteria (PGPR): their potential as antagonists and biocontrol agents. Genet Mol Biol. 2012;35:1044–1051.
- Olishevska S , Nickzad A , Déziel E . Bacillus and Paenibacillus secreted polyketides and peptides involved in controlling human and plant pathogens. Appl Microbiol Biot. 2019;103:1189–1215.
- Li M, Guo R, Yu F, Chen X, Zhao H, Li H, Wu J. Indole-3-acetic acid biosynthesis pathways in the plant-beneficial bacterium Arthrobacter pascens ZZ21. Int J Mol Sci. 2018;19:443.
- Viaene T , Langendries S , Beirinckx S , Maes M , Goormachtig S . Streptomyces as a plant’s best friend? FEMS Microbiol Ecol. 2016:92. doi:10.1093/femsec/fiw119.
- Lugtenberg B , Kamilova F . Plant-growth-promoting rhizobacteria. Annu Rev Microbiol. 2009;63:541–556.
- Rudrappa T , Czymmek KJ , Paré PW , Bais HP . Root-secreted malic acid recruits beneficial soil bacteria. Plant Physiol. 2008;148:1547.
- López-Díaz C, Rahjoo V, Sulyok M, Ghionna V, Martín-Vicente A, Capilla J, Di Pietro A. Fusaric acid contributes to virulence of Fusarium oxysporum on plant and mammalian hosts. Mol Plant Pathol. 2018;19:440-53.
- Dean R, Van Kan JA, Pretorius ZA, Hammond-Kosack KE, Di Pietro A, Spanu PD, Rudd JJ. The top 10 fungal pathogens in molecular plant pathology. Mol Plant Pathol. 2012;13:414-30.
- Farh ME-A, Kim YJ, Van An H, Sukweenadhi J, Singh P, Huq MA, Yang DC. Burkholderia ginsengiterrae sp. nov. and Burkholderia panaciterrae sp. nov., antagonistic bacteria against root rot pathogen Cylindrocarpon destructans, isolated from ginseng soil. Arch Microbiol. 2015;197:439-47.
- Carlucci A , Lops F , Mostert L , Halleen F , Raimondo ML . Occurrence fungi causing black foot on young grapevines and nursery rootstock plants in Italy. Phytopathol Mediterr. 2017;56:10–39.
- Wang W , Zhang D , Wen H , Wang Q , Peng C , Gao J . Soil fungal biodiversity and pathogen identification of rotten disease in Aconitum carmichaelii (Fuzi) roots. Plos One. 2018;13:e0205891.
- Wu H, Qin X, Wang J, Wu L, Chen J, Fan J, Zheng L, Tangtai H, Arafat Y, Lin W, et al. Rhizosphere responses to environmental conditions in Radix pseudostellariae under continuous monoculture regimes. Agr Ecosyst Environ. 2019;270-271:19-31.
- Venturi V , Keel C . Signaling in the rhizosphere. Trends Plant Sci. 2016;21:187–198.
- Doornbos RF , van Loon LC , Bakker PAHM . Impact of root exudates and plant defense signaling on bacterial communities in the rhizosphere. A review. Agron Sustain Dev. 2012;32:227–243.
- Paterson E , Gebbing T , Abel C , Sim A , Telfer G . Rhizodeposition shapes rhizosphere microbial community structure in organic soil. New Phytol. 2007;173:600–610.
- Janvier C , Villeneuve F , Alabouvette C , Edel-Hermann V , Mateille T , Steinberg C . Soil health through soil disease suppression: which strategy from descriptors to indicators? Soil Biol Biochem. 2007;39:1–23.
- Wu LK , Chen J , Wu HM , Qin XJ , Wang JY , Wu YH , Khan MU , Lin S , Xiao ZG , Luo XM , et al. Insights into the regulation of rhizosphere bacterial communities by application of bio-organic fertilizer in Pseudostellaria heterophylla monoculture regime. Front Microbiol. 2016;7:1788.
- Qiu MH, Zhang RF, Xue C, Zhang SS, Li SQ, Zhang N, Shen QR. Application of bio-organic fertilizer can control Fusarium wilt of cucumber plants by regulating microbial community of rhizosphere soil. Biol. Fert. Soils 2012;48:807–816.