ABSTRACT
Exogenous application of methyl jasmonate (MeJA) has been extensively used to study jasmonate-dependent signaling events triggered by biotic stresses. MeJA application leads to complex jasmonate-dependent physiological responses, including changes in stomatal openness and induction of emissions of a multitude of volatile compounds. Whether the alterations in stomatal conductance and emissions of MeJA-induced volatiles are quantitatively associated with MeJA dose, and whether the induced volatile emissions are regulated by modifications in stomatal conductance had been poorly known until recently. Our latest studies highlighted a biphasic kinetics of jasmonate-dependent volatile emissions induced by MeJA treatment in the model species cucumber (Cucumis sativus), indicating induction of an immediate stress response and subsequent gene-expression level response. Both the immediate and delayed responses were MeJA dose-dependent. The studies further demonstrated that stomata modulated the kinetics of emissions of water-soluble volatiles in a MeJA dose-dependent manner. These studies contribute to understanding of plant short- and long-term responses to different biotic stress severities as simulated by treatments with a range of MeJA doses corresponding to mild to acute stress.
Methyl jasmonate as the model to simulate biotic stress
The role of endogenous jasmonic acid (JA) in regulating plant growth and immunizing the plant after abiotic and biotic stresses has been elucidated in numerous studies.Citation1–3 Moreover, it is also widely recognized that the volatile compound methyl jasmonate (MeJA), the methyl ester of JA, could serve as a systemic signal triggering the jasmonate-dependent defense pathways in undamaged parts of the attacked plant or in neighboring non-attacked plants.Citation4–6 Among these defenses, MeJA activates the jasmonate-dependent volatile biosynthesis pathways, leading to induction of volatile organic compound emissions.Citation7–11 Multiple studies have documented elicitation of volatile emissions by exogenous MeJA treatment, including the emissions of volatile products of the lipoxygenase (LOX) pathway (also called green leaf volatiles), and volatile terpenoids and benzenoids, blend of which plays a key role in attracting the enemies of herbivores to the herbivore-infested plants, but also elicits additional defense reactions leading to modified plant nonvolatile metabolome.Citation12,Citation13
Although the severity of biotic stress is predicted to be quantitatively related to the stress-induced volatile emissions, Citation12,Citation14 it is difficult to characterize the relationships between the volatile emissions and damage severity caused by real biotic stresses due to their complex spread in space and time.Citation12,Citation14 For instance, in the case of chewing herbivores, the timing of damage and degree of damage depends on herbivore feeding behaviors as well as size and number of feeding herbivores.Citation8 Exogenous application of biological elicitors such as MeJA that can be applied using precise doses is an alternative approach to simulate the impact of a biotic stress and study the quantitative relationships between the “biotic stress dose” and plant volatile response.Citation15,Citation16
Quantitative responses between MeJA dose and kinetics and amount of volatile emissions
Hence, we designed a series of studies to explore the MeJA-dependent modifications in the kinetics of volatile emission, quantitative scaling of emissions with applied MeJA dose and to improve the overall understanding of the biological significance of MeJA as the chemical signal. We used cucumber (Cucumis sativus L.) as the model plant in these experiments as it is a fast-growing agriculturally important species that is expected to be highly sensitive to different stresses. In addition, the constitutive volatile emissions of cucumber are much lower than stress-induced emissions,Citation17 facilitating the study of induced emissions. Elicitation of volatile emissions was measured in real time using a highly sensitive proton-transfer-reaction time-of-flight mass-spectrometer (PTR-TOF-MS) and the identity of different volatiles was confirmed with gas-chromatography mass-spectrometry (GC-MS).Citation17 MeJA at different concentrations in 5% ethanol was applied on 3–4-week-old, 20–30 cm tall cucumber plants with 4–5 mature leaves cultivated in controlled-conditions (light intensity: 300–400 μmol m−2 s−1, photoperiod: 12/12 h, air temperature: 24°C at day and 20°C at night, air humidity: 60–70%) by spraying the plant leaves uniformly once in the beginning of the experiment.Citation17 Six different concentrations of MeJA were used (0.2, 2, 5, 10, 20, and 50 mM) to obtain the stress severities ranging from mild to severe (leading to hypersensitive response) and 5% ethanol was used as the control.Citation18 Given that only a part of MeJA enters the leaves, relatively high MeJA doses were selected for “severe” stress.Citation17 Unexpectedly, we observed a biphasic kinetics of jasmonate-dependent induction of stress volatile and stress-induced volatile emissions.Citation17 The instant burst of emissions, between 0 and 1.5 h after application of MeJA, was dominated by methanol and LOX pathway volatiles (green leaf volatiles).Citation17 This initial early stress response reflected demethylation of pectins in cell walls and release of polyunsaturated fatty acids from membranes and activation of LOX pathway, responses that are ubiquitous to both abiotic and biotic stresses.Citation18–21 However, since the initial rapid emissions of methanol and LOX volatiles, a second rise of LOX compound emissions occurred in 15–25 h since the MeJA treatment.Citation17 This second rise of LOX volatile emissions corresponded to endogenous activation of JA pathway, and was further associated with elicitation of volatile terpene emissions (including limonene, linalool, and β-farnesene) as the result of activation of expression of corresponding terpene synthases.
In these experiments, the kinetics and magnitude of volatile emission responses were strictly dose-dependent, implying faster and stronger elicitation of both the initial stress response and the secondary elicitation of volatiles. In addition, the higher doses of MeJA led to the hypersensitive reactions and programmed cell death resembling the necrotic lesions formed by insect and pathogen elicitors, and emphasizing the importance of biotic stress severity in determining the downstream events of biological impacts.
Rapid MeJA responses as modulated by stomata
It is well known that stomata play a vital role in controlling the gas exchange between the leaf intercellular air space and ambient air. Moreover, the guard cells respond to complex environmental signals by stomatal opening or closing to regulate water loss and entry of CO2 into the leaf.Citation22,Citation23 MeJA enters plant leaves via stomata as typical for entry of different pathogens.Citation24–26 It is well documented that MeJA can elicit stomatal closure in a wide range of plant speciesCitation27–32 similarly to abscisic acid,Citation33 although the short-term and long-term response kinetics through the initial stress response (up to a few hours) to the endogenous JA response (6–48 h) have not been studied. MeJA-driven stomatal closure can impact both the gaseous MeJA entry into the leaf and emission of volatiles out of the leaf. The rate of change in stomatal conductance (Gs) and the extent of stomatal closure can modify how a given MeJA treatment alters the plant physiological response.Citation34–38
A certain decrease of Gs reduces the entry of all volatiles in a similar manner, and the degree of reduction only depends on the diffusivity of the specific compound. In contrast, the effect of stomatal closure on volatile emission differs qualitatively for different plant volatiles.Citation24,Citation39 In particular, once stomata close, volatile gas-phase concentration starts to build up and ultimately the increased diffusion gradient, ΔC compensates for the initial decrease of the emission rate, I, that had resulted from the reduction in Gs (I = GsΔC). However, the time required for the increase in the diffusion gradient depends on compound partitioning between gas and liquid phases. For water-soluble compounds, the rise of ΔC is slower than for non-water-soluble compounds because a given liquid-phase pool size supports a much lower gas-phase volatile concentration. Accordingly, large increases in the aqueous-phase concentration are necessary to support increases in gas-phase concentration sufficient to reestablish the steady-state emission flux prior to the reduction in Gs.Citation24–26 This implies that the key difference in stomatal control of different compounds is the time needed to achieve a new steady state following a perturbation in stomatal conductance or compound production rate, ultimately determining whether or not the emissions of volatiles can be affected by stomata.Citation24–26 Thus, the rate of emission of water-soluble compounds depends both on their rate of production and Gs.Citation24–26 Among plant volatiles, LOX volatiles and especially methanol are water-soluble, and thus, emission rates of these volatiles can be temporarily controlled by Gs until the liquid-phase concentration rises high enough to support a gas-phase concentration that overrides the reduced conductance.Citation40 However, the way stomatal conductance alters the quantitative and qualitative responses of volatile emissions to MeJA has not been studied until recently.
To further test the hypothesis of the role of stomatal closure in regulating the volatile emissions by MeJA treatment, we investigated the rapid response of stomatal conductance, net assimilation rate (A), and LOX volatile and methanol emissions to varying MeJA concentrations (0.2–50 mM) in cucumber leaves.Citation41 Volatile emissions were again continuously monitored with a PTR-TOF-MS. The study demonstrated that the exposure to MeJA does not just result in stomatal closure, but actually leads to multiphasic stomatal responses, starting with initial opening of stomata, between 0 and 5 min. followed by MeJA concentration-dependent reduction in Gs between 5 min to 1 h.Citation41 At the same time, A initially decreased, followed by recovery for lower MeJA concentrations and time-dependent decline for higher MeJA concentrations.Citation41 These patterns can be explained by an initial osmotic shock after MeJA entry into the leaves and concomitant water efflux from the mesophyll and epidermal cells and hydropassive stomatal opening and reduction in A, followed by loss of turgor of guard cells and stomatal closure. In these experiments, the initial increase of Gs and reduction of A were both dependent on the applied MeJA concentration in a dose-dependent manner.Citation41 MeJA as relatively a water-insoluble molecule does not necessarily induce osmotic shock, but once taken up, MeJA can be demethylated again to more water-soluble methanol and JA.Citation42
In addition to changes in Gs and A, methanol and LOX emissions were elicited in MeJA concentration-dependent manner, whereas the peak methanol emissions (15–20 min after MeJA application) preceded the LOX emissions (20–60 min after application), and monoterpene emissions remained at a very low level (). In the long term (2–40 h), a subsequent LOX emission peak was induced and monoterpene emissions were triggered in MeJA-dose-dependent manner, whereas the methanol emissions gradually ceased ( as in our previous experiments.Citation17 The time-dependent changes in LOX volatile and methanol emissions broadly supported the postulated impacts of MeJA on changes in cellular oxidative status and propagation of lesions. Both the integrated emissions and maximum emission rates of LOX compounds and methanol scaled positively with the concentration of MeJA, indicating that greater MeJA concentrations were associated with more severe oxidative stress.
Figure 1. Generalized responses of emissions of methanol (a, b), lipoxygenase pathway (LOX) volatile compounds (also called green leaf volatiles; c, d) and terpenoids (e, f) to exposure to mild (5 mM) and acute (20 mM) doses of methyl jasmonate (MeJA) in short term (0–80 min; a, c, e) and long term (0–40 h; b, d, f) in leaves of cucumber (Cucumis sativus). The emissions of MeJA-induced volatiles were monitored continuously with a proton-transfer reaction time-of-flight mass spectrometer (PTR-TOF-MS).Citation17
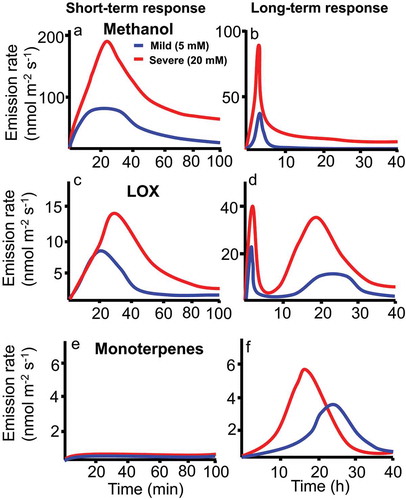
MeJA treatment concentration also importantly altered the short-term emission kinetics. The peak methanol emissions occurred earlier in treatments with higher MeJA concentration (), while the opposite was observed for LOX emissions (). This difference reflected the partial coincidence of the rise of methanol release with MeJA-dependent stomatal opening between 10 and 15 min after the MeJA exposure. As the result, methanol release was initially not limited by stomata, and the methanol release kinetics mirrored the rate of methanol formation. Nevertheless, stomatal closure at 20 min after MeJA application altered methanol emission kinetics by lowering the peak release rate and extending the emission period of methanol release after cessation of its synthesis (). In the case of LOX volatiles, during the first transient enhancement of Gs for 5–10 min after MeJA application, LOX emissions were only slightly induced. The major LOX volatile emissions occurred when stomata already started to close. Thus, increasingly stronger closure of stomata in leaves exposed to greater MeJA concentration led to a progressive delay of peak LOX emission rate.
Conclusions and outlook
These experiments demonstrate that stomata play a pivotal role in controlling the kinetics of volatile emission triggered by the MeJA treatment. The MeJA dose-dependent decrease of Gs implies a reduction of plant sensitivity to airborne MeJA for longer MeJA exposure times (). Once MeJA has entered the leaves, an oxidative burst at the immediate sites of MeJA impact is induced, typically in several minutes after the treatmentCitation17,Citation43,Citation44. This is followed by methanol release and activation of lipoxygenases and elicitation of the first burst of LOX emissions (). Because MeJA also alters Gs, the kinetics of water-soluble stress volatiles are also altered, whereas the effects of Gs depend on when the compound is released, early when stomata open (methanol), or later when stomata start to close (LOX volatiles). These differences have major implications for stress propagation and signaling ().
Figure 2. A model of MeJA dose-dependent responses of leaf photosynthetic traits, signaling molecules and stress-induced volatile emissions in short and long term. In short term, volatiles are released as the result of activation of enzymes that are constitutively active in the leaves and their substrate becoming available, whereas the longer-term elicitation of volatiles is associated with activation of expression of genes responsible for synthesis of volatiles (LOX volatiles, monoterpenes, benzenoids) and generation of an endogenous oxidative burst (LOX volatiles). Gaseous MeJA is taken up into the leaf internal air space through the stomata, permeates further through cell walls and plasmalemma, reaching ultimately the symplastic leaf compartments. This activates an oxidative burst in several minutes after the treatment at the immediate locations of MeJA impact. As the result of constitutive activity of lipoxygenases, free fatty acids released from plant membranes are rapidly converted to volatile lipoxygenase pathway products (LOX volatiles), resulting in the first burst of LOX emissions. Simultaneously with the release of free fatty acids or somewhat earlier, constitutive pectin methylesterases are activated, resulting in major emissions of methanol. In addition, exposure to MeJA leads to concentration-dependent reduction in net assimilation rate (a) and stomatal conductance (Gs). A secondary oxidative burst is elicited 6–48 h after MeJA treatment. This corresponds to a further rise of LOX volatile emissions and elicitation of gene expression level responses as indicated by de novo emissions of terpenoids and benzenoids. Our experiments demonstrated that changes in all studied traits (photosynthetic traits and volatile emissions) were MeJA dose-dependent both in short and long term
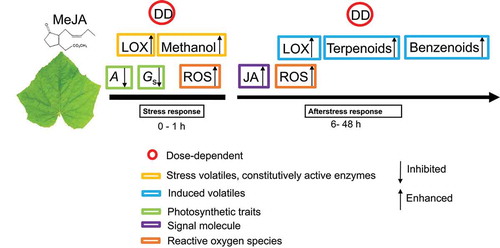
The longer-term sequence of events probably involves an endogenous elicitation of jasmonate production, and generation of a secondary oxidative burst, and interplay with jasmonate repressor proteins (JAZ),Citation45–48 whereas the plant afterstress responses depend on the initial stress severity (). Mild and moderate stress caused by MeJA treatment (5, 10, and 20 mM) is expected to lead to elicitation of gene expression-level defenses including enhanced expression of terpenoid synthase genesCitation49,Citation50 and lipoxygenase pathway genes,Citation51–53 resulting in volatile terpene and LOX emissions that are sustained for a time period of 6–48 h. These results collectively demonstrate that for quantitative understanding of plant responses to airborne elicitors such as MeJA, it is important to consider both the elicitor dose as well as the plant stomatal responses and concomitant modifications in the endogenous plant responses.Citation54 Future studies are needed to characterize the endogenous JA levels as affected by MeJA exposure dose and resulting gene expression patterns.
Disclosure statement
The authors declare no competing interests.
Additional information
Funding
References
- Ali MS, Baek KH. Jasmonic acid signaling pathway in response to abiotic stresses in plants. Int J Mol Sci. 2020;21:1. doi:10.3390/ijms21020621.
- Jang G, Yoon Y, Choi YD. Crosstalk with jasmonic acid integrates multiple responses in plant development. Int J Mol Sci. 2020;21:305. doi:10.3390/ijms21010305.
- Yang J, Duan G, Li C, Liu L, Han G, Zhang Y, Wang C. The crosstalks between jasmonic acid and other plant hormone signaling highlight the involvement of jasmonic acid as a core component in plant response to biotic and abiotic stresses. Front Plant Sci. 2019;10:1349. doi:10.3389/fpls.2019.01349.
- Heil M, Ton J. Long-distance signalling in plant defence. Trends Plant Sci. 2008;13:264–6. doi:10.1016/j.tplants.2008.03.005.
- Kanagendran A, Chatterjee P, Liu B, Sa T, Pazouki L, Niinemets Ü. Foliage inoculation by Burkholderia vietnamiensis CBMB40 antagonizes methyl jasmonate-mediated stress in Eucalyptus grandis. J Plant Physiol. 2019;242:153032. doi:10.1016/j.jplph.2019.153032.
- Tamogami S, Rakwal R, Agrawal GK. Interplant communication: airborne methyl jasmonate is essentially converted into JA and JA-Ile activating jasmonate signaling pathway and VOCs emission. Biochem Bioph Res Co. 2008;376:723–727. doi:10.1016/j.bbrc.2008.09.069.
- Copolovici L, Kännaste A, Remmel T, Niinemets Ü. Volatile organic compound emissions from Alnus glutinosa under interacting drought and herbivory stresses. Environ Exp Bot. 2014;100:55–63. doi:10.1016/j.envexpbot.2013.12.011.
- Copolovici L, Kännaste A, Remmel T, Vislap V, Niinemets Ü. Volatile emissions from Alnus glutinosa induced by herbivory are quantitatively related to the extent of damage. J Chem Ecol. 2011;37:18–28. doi:10.1007/s10886-010-9897-9.
- Frost CJ, Mescher MC, Dervinis C, et al. Priming defense genes and metabolites in hybrid poplar by the green leaf volatile cis-3-hexenyl acetate. New Phytol. 2008;180:722–734. doi:10.1111/j.1469-8137.2008.02599.x.
- Mäntylä E, Blande JD, Klemola T. Does application of methyl jasmonate to birch mimic herbivory and attract insectivorous birds in nature? Arthropod-Plant Inte. 2014;8:143–153. doi:10.1007/s11829-014-9296-1.
- Semiz G, Blande JD, Heijari J, Işık K, Niinemets Ü, Holopainen JK. Manipulation of VOC emissions with methyl jasmonate and carrageenan in the evergreen conifer Pinus sylvestris and evergreen broadleaf Quercus ilex. Plant Biology. 2012;14:57–65. doi:10.1111/j.1438-8677.2011.00485.x.
- Niinemets Ü, Kännaste A, Copolovici L. Quantitative patterns between plant volatile emissions induced by biotic stresses and the degree of damage. Front Plant Sci. 2013;4:262. doi:10.3389/fpls.2013.00262.
- Yamashita F, Rodrigues AL, Rodrigues TM, - Palermo FH, Baluška F, de Almeida LFR. Potential plant-plant communication induced by infochemical methyl Jasmonate in Sorghum (Sorghum bicolor). Plants. 2021;10:485. doi:10.3390/plants10030485.
- Copolovici L, Niinemets Ü. Environmental impacts on plant volatile emission. In: Blande J, Glinwood R, editors. Deciphering chemical language of plant communication. Berlin, Germany: Springer International Publishing; 2016. p. 35–59.
- Shi J, Ma C, Qi D, Lv H, Yang T, Peng Q, Chen Z, Lin Z. Transcriptional responses and flavor volatiles biosynthesis in methyl jasmonate-treated tea leaves. BMC Plant Biol. 2015;15:233. doi:10.1186/s12870-015-0609-z.
- Suh HW, Hyun SH, Kim SH, Lee S-Y, Choi H-K. Metabolic profiling and enhanced production of phytosterols by elicitation with methyl jasmonate and silver nitrate in whole plant cultures of Lemna paucicostata. Process Biochem. 2013;48:1581–1586. doi:10.1016/j.procbio.2013.06.032.
- Jiang YF, Ye JY, Li S, Niinemets Ü. Methyl jasmonate-induced emission of biogenic volatiles is biphasic in cucumber (Cucumis sativus): a high-resolution analysis of dose dependence. J Exp Bot. 2017;68(16):4679–4694. doi:10.1093/jxb/erx244.
- Wang P, Song CP. Guard cell signaling for hydrogen peroxide and abscisic acid. New Phytol. 2008;178:703–718. doi:10.1111/j.1469-8137.2008.02431.x.
- Casson S, Gray JE. Influence of environmental factors on stomatal development. New Phytol. 2008;178:9–23. doi:10.1111/j.1469-8137.2007.02351.x.
- Loreto F, Barta C, Brilli F, Nogues I. On the induction of volatile organic compound emissions by plants as consequence of wounding or fluctuations of light and temperature. Plant Cell Environ. 2006;29(9):1820–1828. doi:10.1111/j.1365-3040.2006.01561.x.
- Li S, Harley PC, Niinemets Ü. Ozone-induced foliar damage and release of stress volatiles is highly dependent on stomatal openness and priming by low-level ozone exposure in Phaseolus vulgaris. Plant Cell and Environ. 2017;40:1984–2003. doi:10.1111/pce.13003.
- Micheli F. Pectin methylesterases: cell wall enzymes with important roles in plant physiology. Trends Plant Sci. 2001;6:414–419. doi:10.1016/S1360-1385(01)02045-3.
- Beauchamp J, Wisthaler A, Hansel A, Kleist E, Miebach M, NiinemetsÜ, Schurr U, Wildt J. Ozone induced emissions of biogenic VOC from tobacco: relations between ozone uptake and emission of LOX products. Plant Cell Environ. 2005;28:1334–1343. doi:10.1111/j.1365-3040.2005.01383.x.
- Niinemets Ü, Loreto F, Reichstein M. Physiological and physicochemical controls on foliar volatile organic compound emissions. Trends Plant Sci. 2004;9:180–186. doi:10.1016/j.tplants.2004.02.006.
- Niinemets Ü, Reichstein M, Staudt M. Stomatal constraints may affect emission of oxygenated monoterpenoids from the foliage of Pinus pinea. Plant Physiol. 2002;130:1371–1385. doi:10.1104/pp.009670.
- Widhalm JR, Jaini R, Morgan JA, Dudareva N. Rethinking how volatiles are released from plant cells. Trends Plant Sci. 2015;20(9):545–550. doi:10.1016/j.tplants.2015.06.009.
- Irving HR, Gehring CA, Parish RW. Changes in cytosolic pH and calcium of guard cells precede stomatal movements. Proc Natl Acad Sci USA. 1992;89:1790–1794. doi:10.1073/pnas.89.5.1790.
- Gehring CA, Irving HR, McConchie R. Jasmonates induce intracellular alkalinization and closure of Paphiopedilum guard cells. Ann Bot. 1997;80:485–489. doi:10.1006/anbo.1997.0471.
- Suhita D, Raghavendra AS, Kwak JM, Vavasseur A. Cytoplasmic alkalization precedes reactive oxygen species production during methyl jasmonate- and abscisic acid-induced stomatal closure. Plant Physiol. 2004;134(4):1536–1545. doi:10.1104/pp.103.032250.
- Munemasa S, Oda K, Watanabe-Sugimoto M. The coronatine-insensitive mutation reveals the hormonal signaling interaction between abscisic acid and methyl jasmonate in Arabidopsis guard cells: specific impairment of ion channel activation and second messenger production. Plant Physiol. 2007;143:1398–1407. doi:10.1104/pp.106.091298.
- Akter N, Okuma E, Sobahan MA, Uraji M, Munemasa S, Nakamura Y, Mori IC, Murata Y. Negative regulation of methyl jasmonate-induced stomatal closure by glutathione in Arabidopsis. J Plant Growth Regul. 2013;32(1):208–215. doi:10.1007/s00344-012-9291-7.
- Shahzad R, Waqas M, Khan AL, Hamayun M, Kang S-M, Lee I-J. Foliar application of methyl jasmonate induced physio-hormonal changes in Pisum sativum under diverse temperature regimes. Plant Physiol Biochem. 2015;96:406–416. doi:10.1016/j.plaphy.2015.08.020.
- Islam MM, Hossain MA, Jannat R, Munemasa S, Nakamura Y, Mori IC, Murata Y. Cytosolic alkalization and cytosolic calcium oscillation in Arabidopsis guard cells response to ABA and MeJA. Plant Cell Physiol. 2010;51(10):1721–1730. doi:10.1093/pcp/pcq131.
- Islam MM, Munemasa S, Hossain MA, Nakamura Y, Mori IC, Murata Y. Roles of AtTPC1, vacuolar two pore channel 1, in Arabidopsis stomatal closure. Plant Cell Physiol. 2010;51(2):302–311. doi:10.1093/pcp/pcq001.
- Hossain MA, Munemasa S, Uraji M, Nakamura Y, Mori IC, Murata Y. Involvement of endogenous abscisic acid in methyl jasmonate-induced stomatal closure in Arabidopsis. Plant Physiol. 2011;156(1):430–438. doi:10.1104/pp.111.172254.
- Yan S, McLamore ES, Dong S. The role of plasma membrane H+-ATPase in jasmonate-induced ion fluxes and stomatal closure in Arabidopsis thaliana. Plant J. 2015;83:638–649. doi:10.1111/tpj.12915.
- Jung S. Effect of chlorophyll reduction in Arabidopsis thaliana by methyl jasmonate or norflurazon on antioxidant systems. J Plant Physiol. Biochem 2004;42:231–255.
- Attaran E, Major I, Cruz J, Rosa BA, Koo AJK, Chen J, Kramer DM, He SY, Howe GA. Temporal dynamics of growth and photosynthesis suppression in response to jasmonate signaling. Plant Physiol. 2014;165(3):1302–1314. doi:10.1104/pp.114.239004.
- Rodriguez-Saona C, Polashock J, Malo E. Jasmonate-mediated induced volatiles in the American cranberry, Vaccinium macrocarpon: from gene expression to organismal interactions. Front Plant Sci. 2013;4:1–17. doi:10.3389/fpls.2013.00115.
- Kanagendran A, Pazouki L, Li S, Liu B, Kännaste A, Niinemets Ü. Ozone-triggered surface uptake and stress volatile emissions in Nicotiana tabacum ‘Wisconsin’. J Exp Bot. 2017;69:681–697. doi:10.1093/jxb/erx431.
- Jiang Y, Ye J, Rasulov B. Role of stomatal conductance in modifying the dose-response of stress volatile emissions in methyl jasmonate treated leaves of cucumber (Cucumis sativa). Int J Mol Sci. 2020;21:1018. doi:10.3390/ijms21031018.
- Garrido I, Espinosa F, Córdoba-Pedregosa MC. Redox-related peroxidative responses evoked by methyl-jasmonate in axenically cultured aeroponic sunflower (Helianthus annuus L.) seedling roots. Protoplasma. 2003;221:79–91. doi:10.1007/s00709-002-0073-0.
- Zhao N, Lin H, Lan S, Jia Q, Chen X, Guo H, Chen F. VvMJE1 of the grapevine (Vitis vinifera) VvMES methylesterase family encodes for Methyl Jasmonate Esterase and has a role in stress response. Plant Physiol Bioch. 2016;102:125–132. doi:10.1016/j.plaphy.2016.02.027.
- Hazra S, Bhattacharyya D, Chattopadhyay S. Methyl jasmonate regulates podophyllotoxin accumulation in Podophyllum hexandrum by altering the ROS-responsive podophyllotoxin pathway gene expression additionally through the down regulation of few interfering miRNAs. Front Plant Sci. 2017;9:164.
- Küpper FC, Gaquerel E, Cosse A, Adas F, Peters AF, Müller DG, Kloareg B, Salaün J-P, Potin P. Free fatty acids and methyl jasmonate trigger defense reactions in Laminaria digitata. Plant Cell Physiol. 2009;50(4):789–800. doi:10.1093/pcp/pcp023.
- Chini A, Fonseca S, Fernández G, Adie B, Chico JM, Lorenzo O, García-Casado G, López-Vidriero I, Lozano FM, Ponce MR, et al. The JAZ family of repressors is the missing link in jasmonate signalling. Nature. 2007;448(7154):666–670. doi:10.1038/nature06006.
- Wasternack C, Song S. Jasmonates: biosynthesis, metabolism, and signaling by proteins activating and repressing transcription. J Exp Bot. 2017;68:1303–1321. doi:10.1093/jxb/erw443.
- Zhang L, Zhang F, Melotto M, Yao J, He SY. Jasmonate signaling and manipulation by pathogens and insects. J Exp Bot. 2017;68:1371–1385. doi:10.1093/jxb/erw478.
- Byun-mckay A, Godard KA, Toudefallah M, Martin DM, Alfaro R, King J, Bohlmann J, Plant AL. Wound-induced terpene synthase gene expression in Sitka spruce that exhibit resistance or susceptibility to attack by the white pine weevil. Plant Physiol. 2006;140:1009–1021. doi:10.1104/pp.105.071803.
- Martin D, Tholl D, Gershenzon J, Bohlmann J. Methyl jasmonate induces traumatic resin ducts, terpenoid resin biosynthesis, and terpenoid accumulation in developing xylem of Norway spruce stems. Plant Physiol. 2002;129(3):1003–1018. doi:10.1104/pp.011001.
- Bell E, Mullet JE. Lipoxygenase gene expression is modulated in plants by water deficit, wounding, and methyl jasmonate. Mol Gen Genet. 1991;230:456–462. doi:10.1007/BF00280303.
- Wasternack C, Parthier B. Jasmonate-signalled plant gene expression. Trends Plant Sci. 2007;2:302–307. doi:10.1016/S1360-1385(97)89952-9.
- Yang XY, Jiang WJ, Yu HJ. The expression profiling of the lipoxygenase (LOX) family genes during fruit development, abiotic stress and hormonal treatments in cucumber (Cucumis sativus L.). Int J Mol Sci. 2012;13:2481–2500. doi:10.3390/ijms13022481.
- Kunert M, Biedermann A, Koch T, Boland W. Ultrafast sampling and analysis of plant volatiles by a hand-held miniaturised GC with pre-concentration unit: kinetic and quantitative aspects of plant volatile production. J Sep Sci. 2002;25(10–11):677–684. doi:10.1002/1615-9314(20020701)25:10/11<677::AID-JSSC677>3.0.CO;2-5.