ABSTRACT
Rice tillering is an important characteristic that responds to both GA (gibberellin) and nitrogen-based fertilizers. How plants balance these two responses? A newly identified NGR5 (NITROGEN-MEDIATED TILLER GROWTH RESPONSE 5) protein reveals its important role in controlling the balance between GA-regulated dwarfism and nitrogen-regulated tillering. NGR5 directly interacts with PRC2 (Polycomb Repressive Complex 2) to form a repressive complex at the shoot branching inhibitory genes in nitrogen-dependent way, thereby repressing branching inhibitors and promoting tillering in response to nitrogen fertilizers. The GA receptor GID1 (GIBBERELLIN INSENSITIVE DWARF1) targets NGR5 for proteolysis by the 26S proteasome. The rice DELLA proteins of GA signaling way competitively inhibit GID1-NGR5 interaction, thereby protecting NGR5 from degradation and enhancing nitrogen-induced tiller number.
The green revolution of the 1960s boosted the productivity of staple crops in great part due to cultivation of semi-dwarf varieties. The green revolution dramatically increases worldwide grain yields of cereal crops, such as rice, maize, millet, and wheat. It was known that the dwarf trait is largely attributed to the reduction of GA activity in cultivated varieties.Citation1–4 For instance, rice varieties have mutations at SD1 (Semi-Dwarf 1) locus causing loss of gibberellin 20-oxidase (GA20ox) function in the GA synthesis pathway, and eventually resulting in a semi-dwarf growth phenotype.Citation5,Citation6 The shorter plants become mechanically more stable to support photosynthetic accumulation and translocate photosynthetic assimilates more efficiently to grains.
Although the semi-dwarf varieties exhibit suitable height and strong lodging resistance which is attractive for agriculture, these varieties show reduced sensitivity to nitrogen, with decreased nitrogen absorption and utilization efficiency, thus relying heavily on nitrogen fertilizer that can potentially lead to environmental pollution. Understanding the relationship between semi-dwarf phenotypes and nitrogen response is of great important for sustainable agriculture and food security. Tillering in rice is a nitrogen-dependent process. The number of cereal tillers or shoot branches that carry grains is a major attribute to the high-yield properties of green revolution varieties. A recent study by Wu et al. provides new insights of how gibberellin signaling and nitrogen fertilizers regulate rice tillering.Citation1 The study’s most striking findings shed light on the molecular mechanisms of GA and nitrogen-regulated rice tillering through the function of NGR5 and open the door of opportunities of increasing rice tillering numbers with reduced nitrogen fertilizer levels by genetic manipulation of these mechanisms to sustain further agricultural development.
Previously, Li et al. reported that the absorption efficiency of ammonium in the O. indica rice variety NJ6 (Nanjing6) was about three times lower than that of NJ6-sd1 (a NJ6 isogenic line containing the sd1 allele).Citation7 By positional mapping, a rice growth regulator GRF4 (GROWTH REGULATING FACTOR 4) was identified. GRF4 gene expression level is higher in sd1 variety and can improve the nitrogen use efficiency.Citation7 Li et al. revealed that the transcription factor GRF4 positively regulates the expression of various nitrogen-metabolism genes and, on the other hand, physically interacts with a growth inhibitor of the GA signaling pathway, thus integrating GA-facilitated plant growth and GRF4-mediated metabolic regulation.Citation7 Now, Wu et al. found that NJ6 showed an increase in the number of tillers and grains with the increase of nitrogen supply. The dwarf variety NJ6-sd1 showed similar phenotype except that it was more obvious than NJ6. Moreover, this phenotype was suppressed by GA treatment or by overexpression of the receptor of GA signaling pathway, GID1 (GIBBERELLIN INSENSITIVE DWARF1). This research further implicates the balance between plant growth (such as tillering number and grain yield) and nitrogen use efficiency can be tipped by modulating the GA signaling pathway.
To better understand the molecular mechanism of GA-regulated plant growth and nitrogen use efficiency, Wu et al. screened an EMS-mutagenized population and initially found that in ngr5 the number of tillers was reduced and insensitive to nitrogen treatment.Citation1 NGR5 is an AP2 (APETALA2) transcription factor, therefore the tillering phenotype is likely to be related to regulating certain sets of genetic expression. RNA-sequencing revealed that the up-regulated genes in ngr5 mutant, such as D14 (Dwarf14) and SPL14 (squamosa promoter binding protein-like–14), were inhibitors of tillering. D14 encodes an α/β hydrolase that hydrolyses the plant branching hormone strigolactone. D14 defines a non-canonical receptor be involved in the perception of the plant branching hormone, strigolactone.Citation8–10 SPL14 encodes an SBP-domain transcription factor of which the downstream targets are still elusive.Citation11,Citation12 Transgenic rice constitutively expressing SPL14 have fewer tillers. Expression of D14 and SPL14 genes decreased with increasing nitrogen treatment.Citation1 ChIP-qPCR analysis further showed that NGR5 can directly bind to the promoter region of D14 and SPL14, and the binding strength increased with increasing nitrogen concentration. There was correlation between up-regulated genes in the ngr5 mutant and the H3K27me3 (histone H3 lysine 27 trimethylation) pattern, which were known to be repressed by histone modification,Citation13–15 suggesting that NGR5 may be involved in PRC2-mediated epigenetic repression. Indeed, NGR5 interacted with PRC2 complex member LC2 (leaf inclination 2). The NGR5 phenotype depends on LC2 because the lc2 mutant has the same phenotype as ngr5, but the lc2 phenotype cannot be restored by overexpression of NGR5.Citation1 Methylation of D14 and SPL14 was increased with nitrogen concentration resulting decreased expression, but there was no difference observed in the methylation status in lc2 and ngr5 background.Citation1,Citation14,Citation16 NGR5 links epigenetic modification of histone acetylation to the phytohormone GA signaling pathway.
GA was found to play an important role in regulating NGR5 mediated tillering. There were many genes co-regulated by NGR5 and GA as revealed by RNA-seq and ChIP analysis, such as D14 and SPL14. Furthermore, GA3 treatment reduced the methylation level of D14 and SPL14 resulting in increased expression. GID1 is the receptor of GA responsible for recruiting DELLAs to enable its polyubiquitination and degradation through 26S proteasome by interacting with GID2 F-box component of the SCFGID2 E3 ubiquitin ligase.Citation17–20 GID1 mutant varieties NJ6-gid1-10 have more tillers than that of NJ6, and its overexpression lines show a decrease in tiller number. Surprisingly, GA treatment could not inhibit the tillers in GID1 mutants or overexpression lines, and failed to inhibit NJ6-sd1-ngr5 tillers as well. Therefore, GA-treatment or GID1-mediated inhibition mimicks the phenotype of ngr5. The abundance of NGR5 increased in NJ6-sd1 and decreased after GA treatment. MG132 treatment inhibited the effect of GA on NGR5, indicating that it was 26S proteasome-mediated degradation. Moreover, this degradation is suppressed in NJ6-gid1-10, hence the ubiquitination and degradation of NGR5 must depend on GID1. Subsequent research found that NGR5-GID1 interacted, and the interaction intensity increased with the treatment of GA.Citation1 Therefore, NGR5 is another substrate for ubiquitination of GID1. Moreover, NGR5 interacts with GID2. NGR5 is ubiquitinated by GID2 in vitro where it is expressed as a substrate of GID2 complex.Citation1 GA-promoted NGR5 degradation was inhibited by MG132, gid1-c1 and gid2-c1 (two CRISPR-Cas9 mutants). Therefore, GA-mediated regulation of NGR5 is not due to the degradation of DELLAs, but depends on direct interaction with GID1, resulting in ubiquitination by the SCFGID2 E3 complex and degradation by 26S proteasome.Citation1
GA promotes plant growth and development by degrading repressors DELLA proteins and its semi-dwarfism is due to accumulation of DELLAs in the green revolution varieties of cereals.Citation21,Citation22 The effect of nitrogen-induced tillering was pronounced in semi-dwarf varieties, which raises the question of whether DELLAs play a role in tillering.Citation1,Citation7 NGR5 was found to directly interact with the rice DELLA protein SLR1 (SLENDER RICE1) and the interaction of LC2-NGR5 could not be interfered by SLR1. The interaction between GID1 and NGR5 is reduced in a DELLA protein accumulated line, Rht-B1b. Using FRET (Fluorescence Resonance Energy Transfer) method, it was found that the reduced GID1-NGR5 interaction in Rht-B1b reduced the degradation of NGR5 and SLR1.Citation1 Further investigation showed that NGR5 was more easily degraded than SLR1. Although GA-promoted degradation of NGR5 is DELLA-independent, the competitive binding of NGR5 and SLR1 to GID reduces the interaction of NGR5-GID1 and improves DELLA function. This competition inhibits GID1-NGR5 interactions in rice and wheat green varieties, thus stabilizing NGR5 by reducing GA-GID1-mediated degradation. In order to determine that DELLA promoted rice tillering was NGR5-dependent, 9311 NILs lines were constructed, including various fusions of SD1, GID1, and NGR5.Citation1,Citation7 When there was NGR5, the lines with the accumulation of SLR1 improved tillering, whereas when NGR5 was missing, there was no difference in tiller numbers under different nitrogen concentration.Citation1
Therefore, DELLA-mediated enhancement of nitrogen-induced tillering depends on the function of NGR5 in the green revolution varieties. Correspondingly, the increased DELLA function in sd1 in response to nitrogen that promotes tillering was caused by increased stability of NGR5. From these results summarized in , we could expect that increased NGR5 expression increases tillering and yield with reduced fertilizer input. Although still premature in promoting its application, Wu et al. study addressed a new important mechanism of modulating plant GA-regulated dwarfism and nitrogen-regulated tillering. Resetting this balance is expected to increase yields and reduce nitrogen fertilizer input.
Figure 1. Schematic representation of NGR5-regulated rice branch in response to gibberellin and nitrogen. The gibberellin receptor GID1 promotes NGR5 polyubiquitination and proteasome degradation to release expression of branching inhibitory genes. DELLA proteins of the GA signaling pathway competitively interact with GID1 to reduce the extent of NGR5-GID1 interaction, thus preclude NGR5 from GID1-mediated destruction. The nitrogen-inducible NGR5 recruits epigenetic repressor PRC2 to the branching inhibitory genes and subsequently repressing the expression of branching inhibitory genes leading to increase in tiller numbers
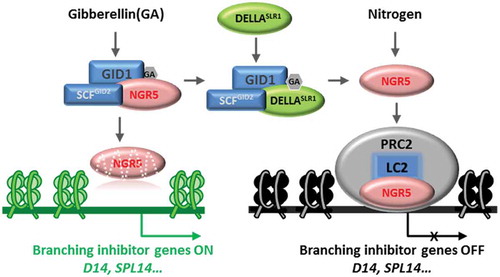
Disclosure statement
No potential conflict of interest was reported by the author(s).
Additional information
Funding
References
- Wu K, Wang S, Song W, Zhang J, Wang Y, Liu Q, Yu J, Ye Y, Li S, Chen J, et al. Enhanced sustainable green revolution yield via nitrogen-responsive chromatin modulation in rice. Science. 2020;367:1. doi:10.1126/science.aaz2046.
- Khush GS. Green revolution: preparing for the 21st century. Genome. 1999;42:646–3. doi:10.1139/g99-044.
- Evenson RE, Gollin D. Assessing the impact of the green revolution, 1960 to 2000. Science. 2003;300:758–762. doi:10.1126/science.1078710.
- Peng J, Richards DE, Hartley NM, Murphy GP, Devos KM, Flintham JE, Beales J, Fish LJ, Worland AJ, Pelica F, et al. “Green revolution” genes encode mutant gibberellin response modulators. Nature. 1999;400:256–261. doi:10.1038/22307.
- Sasaki A, Ashikari M, Ueguchi-Tanaka M, Itoh H, Nishimura A, Swapan D, Ishiyama K, Saito T, Kobayashi M, Khush GS, et al. Green revolution: a mutant gibberellin-synthesis gene in rice. Nature. 2002;416:701–702. doi:10.1038/416701a.
- Spielmeyer W, Ellis MH, Chandler PM. Semidwarf (sd-1), “green revolution” rice, contains a defective gibberellin 20-oxidase gene. Proc Natl Acad Sci USA. 2002;99:9043–9048. doi:10.1073/pnas.132266399.
- Li S, Tian Y, Wu K, Ye Y, Yu J, Zhang J, Liu Q, Hu M, Li H, Tong Y, et al. Modulating plant growth-metabolism coordination for sustainable agriculture. Nature. 2018;560:595–600. doi:10.1038/s41586-018-0415-5.
- Hamiaux C, Drummond RSM, Janssen BJ, Ledger SE, Cooney JM, Newcomb RD, Snowden KC. DAD2 is an α/β hydrolase likely to be involved in the perception of the plant branching hormone, strigolactone. Curr Biol. 2012;22:2032–2036. doi:10.1016/j.cub.2012.08.007.
- Nakamura H, Xue Y-L, Miyakawa T, Hou F, Qin H-M, Fukui K, Shi X, Ito E, Ito S, Park S-H, et al. Molecular mechanism of strigolactone perception by DWARF14. Nat Commun. 2013;4:2613. doi:10.1038/ncomms3613.
- Yao R, Ming Z, Yan L, Li S, Wang F, Ma S, Yu C, Yang M, Chen L, Chen L, et al. DWARF14 is a non-canonical hormone receptor for strigolactone. Nature. 2016;536:469–473. doi:10.1038/nature19073.
- Jiao Y, Wang Y, Xue D, Wang J, Yan M, Liu G, Dong G, Zeng D, Lu Z, Zhu X, et al. Regulation of OsSPL14 by OsmiR156 defines ideal plant architecture in rice. Nat Genet. 2010;42:541–544. doi:10.1038/ng.591.
- Miura K, Ikeda M, Matsubara A, Song X-J, Ito M, Asano K, Matsuoka M, Kitano H, Ashikari M. OsSPL14 promotes panicle branching and higher grain productivity in rice. Nat Genet. 2010;42:545–549. doi:10.1038/ng.592.
- Pien S, Grossniklaus U. Polycomb group and trithorax group proteins in Arabidopsis. Biochim Biophys Acta. 2007;1769:375–382. doi:10.1016/j.bbaexp.2007.01.010.
- Wang J, Hu J, Qian Q, Xue H-W. LC2 and OsVIL2 promote rice flowering by photoperoid-induced epigenetic silencing of OsLF. Mol Plant. 2013;6:514–527. doi:10.1093/mp/sss096.
- Laugesen A, Højfeldt JW, Helin K. Molecular mechanisms directing PRC2 recruitment and H3K27 methylation. Mol Cell. 2019;74:8–18. doi:10.1016/j.molcel.2019.03.011.
- Zhao S-Q, Hu J, Guo L-B, Qian Q, Xue H-W. Rice leaf inclination2, a VIN3-like protein, regulates leaf angle through modulating cell division of the collar. Cell Res. 2010;20:935–947. doi:10.1038/cr.2010.109.
- Gomi K, Sasaki A, Itoh H, Ueguchi-Tanaka M, Ashikari M, Kitano H, Matsuoka M. GID2, an F-box subunit of the SCF E3 complex, specifically interacts with phosphorylated SLR1 protein and regulates the gibberellin-dependent degradation of SLR1 in rice. Plant J. 2004;37:626–634. doi:10.1111/j.1365-313X.2003.01990.x.
- Ueguchi-Tanaka M, Ashikari M, Nakajima M, Itoh H, Katoh E, Kobayashi M, Chow T, Hsing YC, Kitano H, Yamaguchi I, et al. GIBBERELLIN INSENSITIVE DWARF1 encodes a soluble receptor for gibberellin. Nature. 2005;437:693–698. doi:10.1038/nature04028.
- Griffiths J, Murase K, Rieu I, Zentella R, Zhang Z-L, Powers SJ, Gong F, Phillips AL, Hedden P, Sun T, et al. Genetic characterization and functional analysis of the GID1 gibberellin receptors in Arabidopsis. Plant Cell. 2006;18:3399–3414. doi:10.1105/tpc.106.047415.
- Murase K, Hirano Y, Sun T, Gibberellin-induced HT. DELLA recognition by the gibberellin receptor GID1. Nature. 2008;456:459–463. doi:10.1038/nature07519.
- Hedden P. The genes of the green revolution. Trends Genet. 2003;19:5–9. doi:10.1016/S0168-9525(02)00009-4.
- Gao S, Chu C. Gibberellin metabolism and Signaling: targets for improving agronomic performance of crops. Plant Cell Physiol. 2020;61:1902–1911. doi:10.1093/pcp/pcaa104.