ABSTRACT
Nitrate signaling integrates and coordinates the expression of a wide range of genes, metabolic pathways and ultimately, plant growth and development. Calcium signaling is proved to be involved in the primary nitrate response pathway. However, it is much less understood how calcium signaling mediates nitrate sensing and responses from the extracellular space to cytoplasm, then to the nucleus. In this review, we describe how transceptor-channel complex (cyclic nucleotide-gated channel protein 15 interacting with nitrate transceptor, CNGC15-NRT1.1), calcineurin B-like proteins (CBLs, CBL1, CBL9), CBL-interacting protein kinases (CIPKs), phospholipase C (PLC) and calcium-dependent protein kinases (CDPKs, also CPKs), acting as key players, complete a potential backbone of the nitrate-signaling pathway, from the plasma membrane to the nucleus. NRT1.1 together with CBL1/9-CIPK23 and CBL-CIPK8 links the NO3− signaling to cytoplasmic and nuclear regulators and triggers downstream NO3− responses. PLCs and inositol 1, 4, 5-triphosphate (IP3) connect NO3− signaling and cytoplasmic Ca2+ signature. CPK10/30/32 fill the gap between NRT1.1 and NIN-like protein (NLP) transcription factors. The arabidopsis nitrate regulated1 (ANR1) is induced from the endosome by the Ca2+–CPKs–NLPs signaling pathway activated by the unphosphorylated form of NRT1.1 (NRT1.1 T101A) at high nitrate condition. Understanding how calcium signaling interconnects the upstream nitrate sensor complex with downstream multiple sensors of the nitrate-signaling pathway is key to completing the nutrient–growth regulatory networks.
1. Introduction
Nitrate (NO3–) in the soil is not only an essential source of nitrogen for plants, but also functions as a signaling molecule, modulating gene expression and a multitude of physiological and developmental processes, ranging from the control of plant growth, root development, leaf development, seed dormancy, and flowering time as well as the transport and assimilation of NO3.−Citation1–7 Plant adaptation to NO3 – availability is accompanied by a massive and rapid reprogramming of the transcriptome, defined this multifaceted molecular phenomena as the primary nitrate response (PNR). The NO3 – sensing machinery consists of a dual-affinity NO3− transporter and sensor (defines this protein as a “transceptor”), named NRT1.1,Citation6,Citation8,Citation9 nitrate-responsive transcripts, protein phosphatase 2 C (PP2C) and transcription factors.Citation10–18 Despite the progress represented by the identification of the elements of the NO3− -sensing machinery, the molecular regulatory mechanism involving the primary nitrate signaling upstream and the multiple factors and sensors downstream remain to be ascertained. Calcium functions as a key second messenger in diverse physiological and developmental pathways, being associated with stress response, self-incompatibility, nodulation, and the regulation of ion transport.Citation19–30 Ca2+ is also involved in NO3 – signaling, which was first described 20 years ago in maize and barley.Citation31,Citation32 Riveras et al.Citation33 revealed a transient cytoplasmic Ca2+ increase induced by NO3− in Arabidopsis, which was inhibited by the Ca2+ channel blocker lanthanum chloride (LaCl3). Furthermore, this cytoplasmic Ca2+ increase was abolished in the NRT1.1 mutants chl1-5 and chl1-9, pointing to a functional transceptor being indispensable for this response. In addition, it has been shown that inositol 1, 4, 5-triphosphate (IP3) concentrations increased in response to NO3 – treatments, indicating that PLC activity also takes part in this process.Citation33 Notably, the calcium-dependent kinases calcineurin B-like protein (CBL)-interacting protein kinases (CIPKs) and calcium-dependent protein kinases (CPKs) are the master molecular actors regulating the NO3–-signaling pathway. The primary nitrate response pathway has a primary backbone linking the nitrate sensor NRT1.1, CPK calcium-dependent kinases and NLP7-induced gene expression except for the mechanism underlying Ca2+ influx. Ca2+ enters the cytoplasm through Ca2+ channels, which are located in cell membranes and organelles, such as vacuoles and endoplasmic reticulum. Cyclic nucleotide gated channel (CNGCS), denoted as Ca2+ -permeable channels, have been extensively researched in a variety of Ca2+-signaling processes in plants.Citation34–36 In this review, we discuss how CNGC15-NRT1.1, CBLs, CIPKs, PLCs and CPKs preliminarily link the upstream and downstream NO3 – sensing signaling pathway, and we highlight the importance of molecular and genetic analyses in the identification of novel features of calcium in the NO3 – signaling pathway.
2. CNGC15-NRT1.1 couples NO3− sensing to Ca2+ signaling
Cyclic nucleotide gated channel (CNGCS) is a class of nonselective cationic channels with permeability to monovalent and divalent cations, consisting of 20 members in Arabidopsis, which is one of the most important proteins in plants mediate Ca2+-signaling processes.Citation34–36 The membrane-located nitrate transceptor NRT1.1 has been identified as a central component for primary nitrate responses in Arabidopsis.Citation37,Citation38 Interestingly, Wang et al.Citation39 discovered a nutrient transceptor-channel complex named CNGC15-NRT1.1 as a molecular switch controls the channel activity and Ca2+ influx in a nitrate-dependent manner. CNGC15 mutants exhibited the characteristic loss of NO3− -induced Ca2+ signature, which is similar to that in NRT1.1-deficient mutants chl1-5. CNGC15 and NRT1.1 formed a plasma membrane Ca2+-permeable channel, but the channel activity of the heterocomplex was inhibited. After addition of NO3−, the interaction between CNGC15-NRT1.1 was weakened, and the Ca2+ channel activity of CNGC15-NRT1.1 complex was restored, thus forming the CNGC15-NRT1.1 complex which could be dynamically gated by NO3−. This novel “transceptor-channel” or “transporter-calcium channel” gated by small nutrient molecules further improves the nitrate nutrient signal transduction pathway and nitrate triggered calcium signaling transduction pathway in plants.
3. CBL-CIPKs in NO3− signaling
Calcineurin-B like proteins (CBL) is a group of calcium sensor proteins that interact with CIPK kinases (CBL-interacting protein kinases), which phosphorylate their downstream target proteins.Citation40,Citation41 In Arabidopsis, 10 calcineurin B-like proteins (CBLs), with 26 CIPKs have been discovered so far.Citation41–43 CBL7 was reported to regulate the low-concentration nitrate response process, which was mainly expressed in the roots of seedlings and induced by nitrate starvation. In the absence of nitrate, the loss of CBL7 inhibited root growth and decreased the expression of NRT2.4/2.5.Citation44 In addition, CBL1 and ABI2, a member of the protein phosphatase 2 C family, were found to regulate nitrate transport, sensing, and signaling pathways.Citation10,Citation45 In particular, the NO3 – signaling needs to be transmitted to the nucleus and magnified through cytoplasmic regulators when NO3 – is sensed by NRT1.1.Citation46 The CBL-CIPK system may play a key role in the NO3–-signaling pathway, linking up the NO3− signal with cytoplasmic and nuclear regulators and triggering downstream NO3− responses.
CBL1 and CBL9 interact with CIPK23 and recruit the kinase to the plasma membrane where the substrate(s) of CIPK23 may reside, regulating the nitrate transporter NPF6.3/NRT1.1 via phosphorylation to modulate nitrate uptake.Citation43 NPF6.3/NRT1.1has been characterized as a dual-affinity NO3 – transporter, with T101-phosphorylated NRT1.1 acting as a high-affinity NO3 – transporter, but as a low-affinity NO3− transporter when it is dephosphorylated.Citation37 NRT1.1 is a transceptor for NO3–, the activity of which regulates a complicated network of multiple kinases and phosphatases affecting PNR.Citation8,Citation10 The calcium-dependent protein kinase CIPK23 was found to phosphorylate T101 of NRT1.1 when NO3 – concentrations were lowCitation8,Citation47 (). This suggested that NRT1.1 may trigger the changes in NO3–-induced calcium increases by regulating a dual-affinity binding and a phosphorylation switch in response to a range of NO3 – concentrations ().
Figure 1. Calcium signaling networks mediating nitrate signaling pathway. NO3 – is sensed by CHL1/NRT1.1/NPF6.3 under both high and low concentrations. CIPK8 (a CBL-interacting protein kinase, interacting with CBL) regulates NRT1.1 in low-affinity responses, whereas CIPK23 (a CBL-interacting protein kinase, interacting with CBL1/9) phosphorylates NRT1.1 under high-affinity responses. CNGC15-NRT1.1 as a molecular switch controls the channel activity and Ca2+ influx. NRT1.1 and PLC activity co-regulate the increase in cytoplasmic Ca2+ concentration in response to NO3–. When Ca2+ accumulates in the nucleus, NLP7 is phosphorylated by CPKs and is retained in the nucleus to regulate PNR. Ca2+–CPKs–ANR1 signaling pathway is activated by unphosphorylated form of NRT1.1 (NRT1.1T101A) at high nitrate (HN) condition
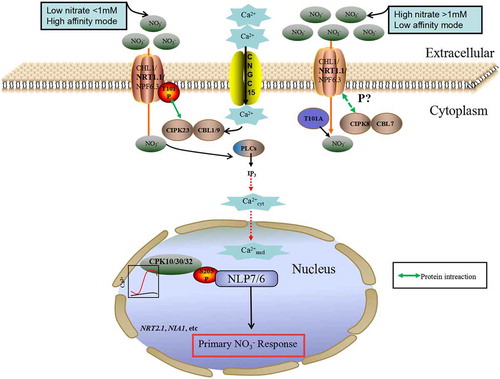
Another CBL-CIPK gene that was elucidated to to regulate NO3 – signaling is CIPK8, especially in low-affinity responses.Citation48 Hu et al.Citation48 identified that the expression of AtCIPK8 was strongly and rapidly induced by NO3 – (). Thus, this work described a potential link between calcium signaling and NO3 – signaling. In addition, they confirmed that CIPK8 positively mediated the NO3 – responses of PNR marker genes and modulated primary root growth using cipk8 knockout mutants.Citation48 Although a role for AtCIPK8 in regulating NO3 – sensing and signaling has been implicated, many outstanding questions remain to be answered, such as whether NO3− can trigger Ca2+-CIPK signaling, how CIPK8 perceives cytoplasmic calcium increases, and the direct target(s) of CIPK8 remains to be revealed in the NO3− signaling network.
Table 1. CNGC, CBLs, CIPKs, CPKs, NLP7 and ANR1 involved in nitrate signaling
4. PLCs in NO3 – signaling
PLCs are key enzymes in phosphatidyl inositol turnover, accompanied by multiple second messengers.Citation49 Based on differences in substrate specificities, PLCs can be divided into phosphoinositide-specific phospholipase C (PI-PLC) and nonspecific phospholipase C in plants.Citation50 IP3 and diacylglycerol (DAG) are the two second intracellular messengers generated from phosphatidylinositol 4,5-bisphosphate (PIP2) hydrolyzed by PI-PLCs.Citation50,Citation51 IP3 induces Ca2+ released from internal stores (). The released Ca2+ may be acting as a second messenger in the NO3− -signaling network. Interestingly, it has been shown that IP3 and cytoplasmic Ca2+ levels increased in response to NO3 – treatment, but this response was blocked by the PLC inhibitor U73122.Citation33 This result suggested that PLC is required for NO3–-dependent increases in cytoplasmic Ca2+ levels and IP3. Furthermore, it has been shown that an increase in IP3 concentration in Arabidopsis roots in response to NO3 – treatments also requires NRT1.1 for activation of a PLC activity.Citation33 In addition, DAG can not only activate protein kinase C to transduce the signal to effector molecules but can also generate diacylglycerolpyrophosphate, which serves as a reasonable substrate for phosphatidic acid (PA) under the action of phospholipase D. Nevertheless, it is currently largely unknown whether PA affects cytoplasmic Ca2+ concentration in plants.
5. CPKs in NO3− signaling
5.1. CPK10/30/32 in NO3− signaling
Ca2+-dependent protein kinases (CDPKs, in Arabidopsis designated as CPKs), a family of Ser/Thr kinases, contains a calcium binding site (EF hand) in their C-terminal region. Binding of Ca2+ to the EF hand stimulates a conformational change, thus allowing autophosphorylation of the kinase.Citation42,Citation52 There are 34 CPK genes in the Arabidopsis genome, and more than half of them have been functionally characterized to modulate varying signaling pathways.Citation41,Citation53–55 CPKs are the long-anticipated molecular link between the major players in the NO3− signaling pathway, the NO3− transceptor NRT1.1 located in the plasma membrane and the NIN-like protein (NLP) transcription factors located in the cell nucleus.Citation46,Citation56 NLPs have been identified as key transcription factors of primary nitrate responses in Arabidopsis. NO3− can not only induce calcium increase in the nucleus but also trigger Ca2+-CPK signalingCitation56(). Then Liu et al.Citation56 identified subgroup III CPKs acting as master regulators mediating primary NO3− signaling. The development of an integrated seeding and cell-based analysis method, as well as a versatile single-cell analysis system, has contributed to the report of unique Ca2+ signaling triggered by nitrate so as to identify initially the mechanism of nitrate signaling transduction. The researchers then demonstrated that CPKs are the master regulators mediating primary NO3− signaling by using an ultrasensitive calcium biosensor, a sensitized and targeted functional genomic screen, and a chemical switch (a unique mutant system) successfully overcome the embryo lethality of cpk10 cpk30 double mutant. However, it remains unknown how plants transmit the nitrate signaling downstream via calcium-dependent protein kinases–CPK10, CPK30 and CPK32 after sense this signaling. The study reported that nitrate can not only induce Ca2+ accumulation in the nucleus, but also can induce CPKs translocation in the nucleus. Furthermore, CPK10 CPK30 and CPK32 phosphorylated the key transcription factor of PNR named NLP7 in the nucleus in response to nitrate. Phosphorylation proteomic analysis showed that the phosphorylation site was Ser205 in NLP7, and NLP7 (Ser205A) mutant lacking the CPK10, CPK30 and CPK32 phosphorylation site was not present in the nucleus and could not complement the root phenotype of the mutant nlp7, indicating that Ca2+ acted as a second messenger in response to nitrate and then modulate CPK activity so as to control the entry of NLP7 into the nucleus to interact with NLP7. This findings connected a new Ca2+–CPK–NLP signaling cascade to comprehensive nitrate responses and revealed a previously unrecognized function of Ca2+-sensor CPKs as master regulators that modulating the primary nitrate-signaling.Citation56However, it remains incompletely unclear how and why CPKs are shuttled from the cytoplasm into the nucleus in response to NO3–; whether plants can perceive cytoplasmic Ca2+ waves as opposed to nuclear ones; and which compartment can provide a link between cytosolic and nuclear calcium concentration.
5.2 Ca2+–CPKs–ARABIDOPSIS NITRATE REGULATED1 (ANR1)
ANR1, as a member of the plant MIKC-type MADS box family of transcription factors, was first found to play roles in linking external NO3− to lateral root (LR) development.Citation57 Gan et al.Citation58 illustrated that ANR1 positively regulated LR development and early seedling growth, in response to NO3− regulation in Arabidopsis thaliana. Most recently, Zhang et al. reveals that Ca2+–CPKs–ANR1 signaling pathway stimulated from the endosome, in conjunction with auxin transporting, plays an essential role in lateral root growth, which is regulated by the phosphorylated form of NRT1.1.Citation59 The NRT1.1 T101A nonphosphorylatable mutants exhibited a transient increase of Ca2+ signaling in cytoplasm in both high nitrate (HN) and low nitrate (LN) conditions. But the NRT1.1 T101D phosphomimetic mutants showed a reduction. This is an interesting point and future efforts are needed to investigate the detailed mechanism. In addition, the ANR1 expression in T101A line was higher than that of T101D line under HN condition. Therefore, the Ca2+–CPKs–NLPs signaling pathway could be activated from the endosome by the nonphosphorylated state of NRT1.1 together with elevating the expression of ANR1. Despite this progress, how the phosphorylated and dephosphorylated variants of NRT1.1 at T101 in LN condition affect Ca2+ signaling through the ANR1 deserves for further study. It will be interesting to explore the detailed mechanism of how ANR1 links cytoplasmic Ca2+ to PNR in the nucleus to better understand how calcium signaling interconnects the upstream nitrate sensor complex with downstream multiple sensors of the nitrate-signaling pathway.
6. Future research directions
This review describes how calcium signaling is involved in the NO3 – -sensing and -signaling pathways so as to establish the potential backbone of the NO3–-signaling pathway, from the plasma membrane to the nucleus. A single nitrate ‘transceptor’ NRT1.1 mediates both high-affinity and low-affinity nitrate uptake up to its phosphorylated status at threonine residue 101, regulated by CIPK23. The protein kinase CIPK8 is responsible for NO3 – sensing by regulating the low-affinity phase of the PNR.Citation48 Future research should focus on how CIPK8 participates in the NO3–-sensing pathway, such as the molecular mechanism elucidating the identity of the elements upstream and downstream of the sensors, to fill the gap in the NO3–-sensing network. In addition, the FIP1 (factor interacting with poly (A) polymerase) gene negatively regulated the expression of CIPK8 and CIPK23 when NO3− was present.Citation60 It will be interesting to elucidate how FIP1 regulates co-ordination of the calcium signal with the NO3 – signal, which will complement the NO3–-signaling pathway connection with CIPKs. Nevertheless, it is also emerging that we need to decipher the mechanistic details of how precisely CBL-CIPK complexes are formed and how they recognize their targets in much more resolution in order to achieve a more comprehensive understanding of plant nutrition regulation.
NRT1.1 and PLC activity co-regulate the increase in cytoplasmic Ca2+ concentration in response to NO3–. In this process, PLC hydrolyzes PIP2 into IP3 and DAG, with the increased IP3 concentration correlating positively with an increase in cytoplasmic Ca2+ concentration. Future research should focus on determining whether IP3 directly triggers the increase in cytoplasmic Ca2+ concentration or operates through its phosphorylated product IP6 in NO3–-mediated Ca2+ release in the NO3 – PLC-Ca2+ pathway.
CPKs bridge the gap between calcium increases triggered by NO3 – and NLP7/6, which are recognized to be pivotal transcription factors of PNR.Citation46,Citation56 This is the first time that a branch of the NO3–-signaling pathway has been revealed in its entirety. This finding prompts important questions such as whether CPKs can also phosphorylate NLP7 in the cytoplasm to regulate its nuclear import and whether NRT1.1 is also regulated by calcium through CIPK or CBL. Although phosphoproteomic analysis has shown that serine 205 of NLP7 is phosphorylated in the presence of NO3 – in the nucleus,Citation56 the mechanism behind the change in nuclear calcium concentrations remain to be elucidated. Future work should pay great attention to identifying the receptors, channels and other regulators playing roles in creating complex Ca2+ signatures in response to NO3–, other nutrients, microRNAs, long non-coding RNAs, circular RNAs, peptides, hormones and environmental cues; this research will be accelerated by new advances in the development of ultrasensitive Ca2+ biosensors.
Despite the critical importance of the calcium signals being transmitted downstream by CPKs in plants, the biochemical pathways that underpin the fundamental cellular program connecting [Ca2+]cyt to [Ca2+]nucl remain largely unknown. The changes in intracellular calcium concentration are not a synchronized process, either temporally or spatially.Citation61 Under different conditions, calcium concentrations in different organelles and other sub-cellular compartments vary, and these different calcium signatures participate in different cellular events. It is well known that the nuclear pore complex (NPC) forms a large channel for the free diffusion of ions and macromolecules.Citation62 Calcium could diffuse into and out of the nucleoplasm through the NPC, and changes in the nuclear calcium concentration would be dependent on the cytoplasmic calcium concentration. Researchers in this field urgently require Ca2+-signaling researchers to provide a better understanding of the changes in calcium concentration in the cytoplasm and the nucleus in order to fill the NO3 – gap in the NO3–-signaling pathway so as to refine our understanding of Ca2+-signaling processes in plants.
NO3 – signaling is closely associated with hormonal signaling in order to adjust plant growth and development in response to nutrient availability.Citation63–65 It is noteworthy that CPK10 and CPK30 were demonstrated to be related to abscisic acid (ABA) signaling 20 years ago.Citation66 This exciting link opens up promising avenues of research on the co-control of the NO3–- and ABA-signaling pathways. This information can provide us with a greater understanding of the intimate connections between NO3− -signaling and hormonal signaling pathways. NRT1.1 has been demonstrated to play diverse roles in plants, not only in nitrate uptake and transport, and nitrate signal transduction, but also in auxin transport and mediating root developmentCitation67(Wang et al 2020). AtNRT1.1 has been verified to transport auxin and regulate nitrate-modulated lateral root development (Krouk et al., 2010).Citation68 In addition, the phosphorylated form of NRT1.1 (named as NRT1.1T101D) accelerates auxin flux and inhibits lateral root elongation under low nitrate conditionsCitation38 (Bouguyon et al., 2015), however, the unphosphorylated form of NRT1.1 (NRT1.1 T101A) suppresses auxin transport and triggers Ca2+–CPKs–ANR1 signaling pathway to modulate LR developmentCitation59(Zhang et al. 2019). Further work demonstrated that nitrate can increase the transcription and mRNA accumulation of AtNRT1.1 in the lateral root primordium, but inhibits the accumulation of AtNRT1.1 protein in the lateral root primordium, with a concomitant increase of auxin accumulation to enhance lateral root elongation, indicating that nitrate can modulate AtNRT1.1 at the post-transcriptional level in a tissue-specific mannerCitation9(Bouguyon et al., 2016). Recent work has uncovered that AtNRT1.1 displays auxin biosynthesis activity as well as auxin transport activity to modulate root branching when nitrate is available. AtNRT1.1 negatively regulates the expression of TAR2(one auxin biosynthetic gene) and LAX3 (auxin influx carrier), which inhibits auxin biosynthesis and represses the lateral root primordia development. However, this phenomenon is suppressed at high nitrate availabilityCitation6(Maghiaoui 2020). These results suggest a vital role of AtNRT1.1 in regulating root system architecture in response to nutrient availability. Future efforts are needed to investigate the integrated roles of NRT1.1 providing an comprehensive overview of the functions of NRT1.1 in plants.
Calcium signaling triggered by NO3 – at the transcriptional and post-transcriptional levels is a recent research avenue, as the components of calcium- and NO3–-signaling pathways (such as receptors, kinases, and transcription factors) were not identified until the current decade. Further transporters and signaling components still await discovery and their roles in functional and regulatory mechanisms remain to be elucidated. Ultimately, a greater understanding of the landscape of NO3–-sensing and -signaling pathways and how calcium-signaling networks connect with target biological processes is key to devising new biotechnological strategies to increase nitrogen-use efficiency in crops for sustainable agriculture.
Abbreviations
Acknowledgments
We thank TopEdit (www.topeditsci.com) for its linguistic assistance during the preparation of this manuscript.
Disclosure Statement
No potential conflicts of interest were disclosed.
Additional information
Funding
References
- Wang R, Tischner R, Gutiérrez RA, Hoffman M, Xing X, Chen M, Coruzzi G, Crawford NM. Genomic analysis of the nitrate response using a nitrate reductase-null mutant of Arabidopsis. Plant Physiol. 2018;136:1–7. doi:10.1104/pp.104.044610.
- O’Brien JA, Vega A, Bouguyon E, Krouk G, Gojon A, Coruzzi G, Gutiérrez RA. Nitrate transport, sensing and responses in plants. Mol Plant. 2016;9:837–856. doi:10.1016/j.molp.2016.05.004.
- Undurraga SF, Ibarra-Henriquez C, Fredes I, Alvarez JM, Gutierrez RA. Nitrate signaling and early responses in Arabidopsis roots. J Exp Bot. 2017;68:2541–2551. doi:10.1093/jxb/erx041.
- Fredes I, Moreno S, Díaz FP, Gutiérrez RA. Nitrate signaling and the control of Arabidopsis growth and development. Curr Opin Plant Biol. 2018;47:112–118. doi:10.1016/j.pbi.2018.10.004.
- Ye JY, Tian WH, Zhou M, Zhu QY, Jin CW. Improved plant nitrate status involves in flowering induction by extended photoperiod. Front Plant Sci. 2021:12. doi:10.3389/fpls.2021.629857.
- Maghiaoui A, Bouguyon E, Cuesta C, Perrine-Walker F, Alcon C, Krouk G, Benková E, Nacry P, Gojon A, Bach L. The Arabidopsis NRT1.1 transceptor coordinately controls auxin biosynthesis and transport to regulate root branching in response to nitrate. J Exp Bot. 2020;71(15):4480–4494. doi:10.1093/jxb/eraa242.
- Sakuraba Y, Chaganzhana, Mabuchi A, Iba K, Yanagisawa S. Enhanced NRT1.1/NPF6.3 expression in shoots improves growth under nitrogen deficiency stress in arabidopsis. Commun Biol. 2021;4(1). doi:10.1038/s42003-021-01775-1.
- Ho CH, Lin SH, Hu HC, Tsay YF. CHL1 functions as a nitrate sensor in plants. Cell. 2009;138:1184–1194. doi:10.1016/j.cell.2009.07.004.
- Bouguyon E, Perrine-Walker F, Pervent M, Rochette J, Cuesta C, Benkova E, Martinière A, Bach L, Krouk G, Gojon A, et al. Nitrate controls root development through post-transcriptional regulation of the NRT1.1/NPF6.3 transporter/sensor. Plant Physiol. 2016;172:1237–1248. doi:10.1104/pp.16.01047.
- Leran S, Edel KH, Pervent M, Hashimoto K, Corratgé-Faillie C, Offenborn JN, Tillard P, Gojon A, Kudla J, Lacombe B. Nitrate sensing and uptake in Arabidopsis are enhanced by ABI2, a phosphatase inactivated by the stress hormone abscisic acid. Sci Signal. 2015;8:ra43. doi:10.1126/scisignal.aaa4829.
- Vidal EA, Álvarez JM, Moyano TC, Gutiérrez RA. Transcriptional networks in the nitrate response of Arabidopsis thaliana. Curr Opin Plant Biol. 2015;27:125–132. doi:10.1016/j.pbi.2015.06.010.
- Konishi M, Yanagisawa S. Arabidopsis NIN-like transcription factors have a central role in nitrate signaling. Nat Commun. 2013;4:1617–1625. doi:10.1038/ncomms2621.
- Marchive C, Roudier F, Castaings L, Bréhaut V, Blondet E, Colot V, Meyer C, Krapp A. Nuclear retention of the transcription factor NLP7 orchestrates the early response to nitrate in plants. Nat Commun. 2013;4:1713–1721. doi:10.1038/ncomms2650.
- Guan P, Ripoll JJ, Wang R, Vuong L, Bailey-Steinitz LJ, Ye D, Crawford NM. Interacting TCP and NLP transcription factors control plant responses to nitrate availability. Proc Natl Acad Sci USA. 2017;114:2419–2424. doi:10.1073/pnas.1615676114.
- Zhong L, Chen D, Min D, Li W, Xu Z, Zhou Y, Li L, Chen M, Ma Y. AtTGA4, a bZIP transcription factor, confers drought resistance by enhancing nitrate transport and assimilation in Arabidopsis thaliana. Biochem Biophys Res Commun. 2015;457:433–439. doi:10.1016/j.bbrc.2015.01.009.
- Canales J, Contreras-López O, Álvarez JM, Gutiérrez RA. Nitrate induction of root hair density is mediated by TGA1/TGA4 and CPC transcription factors in Arabidopsis thaliana. Plant J. 2017;92:305–316. doi:10.1111/tpj.13656.
- Guan P, Wang R, Nacry P, Breton G, Kay SA, Pruneda-Paz JL, Davani A, Crawford NM. Nitrate foraging by Arabidopsis roots is mediated by the transcription factor TCP20 through the systemic signaling pathway. Proc Natl Acad Sci USA. 2014;111:15267–15272. doi:10.1073/pnas.1411375111.
- Alvarez JM, Riveras E, Vidal EA, Gras DE, Contreras-López O, Tamayo KP, Aceituno F, Gómez I, Ruffel S, Lejay L, et al. Systems approach identifies TGA1 and TGA4 transcription factors as important regulatory components of the nitrate response of Arabidopsis thaliana roots. Plant J. 2014;80:1–13. doi:10.1111/tpj.12618.
- Hepler PK. Calcium: a central regulator of plant growth and development. Plant Cell. 2005;17:2142–2155. doi:10.1105/tpc.105.032508.
- Clapham DE. Calcium signaling. Cell. 2007;131:1047–1058. doi:10.1016/j.cell.2007.11.028.
- Batistič O, Kudla J. Analysis of calcium signaling pathways in plants. Biochim Biophys Acta. 2012;1820:1283–1293. doi:10.1016/j.bbagen.2011.10.012.
- Iwano M, Ito K, Fujii S, Kakita M, Asano-Shimosato H, Igarashi M, Kaothien-Nakayama P, Entani T, Kanatani A, Takehisa M, et al. Calcium signaling mediates self-incompatibility response in the Brassicaceae. Nat Plants. 2015;1:15128. doi:10.1038/nplants.2015.128.
- Denninger P, Bleckmann A, Lausser A, Vogler F, Ott T, Ehrhardt DW. Male-female communication triggers calcium signatures during fertilization in Arabidopsis. Nat Commun. 2014;5:4645. doi:10.1038/ncomms5645.
- Charpentier M, Sun J, Vaz Martins T, Radhakrishnan GV, Findlay K, Soumpourou E, Thouin J, Very AA, Sanders D, Morris RJ, et al. Nuclear localized cyclic nucleotide-gated channels mediate symbiotic calcium oscillations. Science. 2016;352:1102–1105. doi:10.1126/science.aae0109.
- Chaves-Sanjuan A, Sanchez-Barrena MJ, Gonzalez-Rubio JM, Moreno M, Ragel P, Jimenez M, Pardo JM, Martinez-Ripoll M, Quintero FJ, Albert A. Structural basis of the regulatory mechanism of the plant CIPK family of protein kinases controlling ion homeostasis and abiotic stress. Proc Natl Acad Sci USA. 2014;111:4532–4541. doi:10.1073/pnas.1407610111.
- Kudla J, Becker D, Grill E, Hedrich R, Hippler M, Kummer U, Parniske M, Romeis T, Schumacher K. Advances and current challenges in calcium signaling. New Phytol. 2018;218:414–431. doi:10.1111/nph.14966.
- Choi WG, Hilleary R, Swanson SJ, Kim SH, Gilroy S. Rapid, long-distance electrical and calcium signaling in plants. Annu Rev Plant Biol. 2016;67:287–307. doi:10.1146/annurev-arplant-043015-112130.
- Costa A, Kudla J. Colorful insights: advances in imaging drive novel breakthroughs in Ca2+ signaling. Mol Plant. 2015;8:352–355. doi:10.1016/j.molp.2014.11.020.
- Guo H, Feng P, Chi W, Sun X, Xu X, Li Y, Ren D, Lu C, David Rochaix J, Leister D, et al. Plastid-nucleus communication involves calcium-modulated MAPK signalling. Nat Commun. 2016;7:12173. doi:10.1038/ncomms12173.
- Edel KH, Kudla J. Increasing complexity and versatility: how the calcium signaling toolkit was shaped during plant land colonization. Cell Calcium. 2015;57:231–246. doi:10.1016/j.ceca.2014.10.013.
- Sakakibara H, Kobayashi K, Deji A, Sugiyama T. Partial characterization of the signaling pathway for the nitrate-dependent expression of genes for nitrogen-assimilatory enzymes using detached maize leaves. Plant Cell Physiol. 1997;38:837–843. doi:10.1093/oxfordjournals.pcp.a029242.
- Sueyoshi K, Mitsuyama T, Sugimoto T, Kleinhofs A, Warner RL, Oji Y. Effects of inhibitors for signaling components on the expression of the genes for nitrate reductase and nitrite reductase in excised barley leaves. Soil Sci Plant Nutr. 1999;45:1015–1019. doi:10.1080/00380768.1999.10414353.
- Riveras E, Alvarez JM, Vidal EA, Oses C, Vega A, Gutiérrez RA. The calcium ion is a second messenger in the nitrate signaling pathway of Arabidopsis. Plant Physiol. 2015;169:1397–1404. doi:10.1104/pp.15.00961.
- Gao QF, Gu LL, Wang HQ, Fei CF, Fang X, Hussain J, Sun SJ, Dong JY, Liu H, Wang YF. Cyclic nucleotide-gated channel 18 is an essential Ca2+ channel in pollen tube tips for pollen tube guidance to ovules in Arabidopsis. Proc Natl Acad Sci USA. 2016;113:3096–3101. doi:10.1073/pnas.1524629113.
- Zhang S, Pan Y, Tian W, Dong M, Zhu H, Luan S, Li L. Arabidopsis CNGC14 mediates calcium influx required for tip growth in root hairs. Mol Plant. 2017;10:1004–1006. doi:10.1016/j.molp.2017.02.007.
- Tian W, Hou C, Ren J, Wang C, Zhao F, Dahlbeck D, Hu S, Zhang L, Niu Q, Li L, et al. A calmodulin-gated calciumchannel links pathogen patterns to plant immunity. Nature. 2019;572:131–135. doi:10.1038/s41586-019-1413-y.
- Liu KH, Tsay YF. Switching between the two action modes of the dual-affinity nitrate transporter CHL1 by phosphorylation. EMBO J. 2003;22:1005–1013. doi:10.1093/emboj/cdg118.
- Bouguyon E, Brun F, Meynard D, Kubeš M, Pervent M, Leran S, Lacombe B, Krouk G, Guiderdoni E, Zažímalová E, et al. Multiple mechanisms of nitrate sensing by Arabidopsis nitrate transceptor NRT1.1. Nat Plants. 2015;1:15015. doi:10.1038/nplants.2015.15.
- Wang X, Feng C, Tian L, Hou C, Tian W, Hu B, Zhang Q, Ren ZJ, Niu Q, Song JL, et al. A transceptor-channel complex couples nitrate sensing to calcium signaling in Arabidopsis. Mol Plant. 2021;14:774–786. doi:10.1016/j.molp.2021.02.005.
- Mao J, Manik S, Shi S, Chao J, Jin Y, Wang Q, Liu H. Mechanisms and physiological roles of the cbl-cipk networking system in Arabidopsis thaliana. Genes. 2016;7(9):62. doi:10.3390/genes7090062.
- Saito S, Uozumi N. Calcium-regulated phosphorylation systems controlling uptake and balance of plant nutrients. Front Plant Sci. 2020:11. doi:10.3389/fpls.2020.00044.
- Hashimoto K, Kudla J. Calcium decoding mechanisms in plants. Biochimie. 2011;93:2054–2059. doi:10.1016/j.biochi.2011.05.019.
- Dong QY, Bai B, Almutairi BO, Kudla J. Emerging roles of the cbl-cipk calcium signaling network as key regulatory hub in plant nutrition. J Plant Physiol. 2021;257:153335. doi:10.1016/j.jplph.2020.153335.
- Ma Q, Tang RJ, Zheng XJ, Wang SM, Luan S. The calcium sensor CBL7 modulates plant responses to low nitrate in Arabidopsis. Biochem Biophys Res Commun. 2015;468:59–65. doi:10.1016/j.bbrc.2015.10.164.
- Yang J, Deng X, Wang X, Wang JZ, Du SY, Li YS. The calcium sensor oscbl1 modulates nitrate signaling to regulate seedling growth in rice. PLoS ONE. 2019;14(11):e0224962. doi:10.1371/journal.pone.0224962.
- Krouk G. Nitrate signaling: calcium bridges the nitrate gap. Nat Plants. 2017;3:17095. doi:10.1038/nplants.2017.95.
- Vert G, Chory J. A toggle switch in plant nitrate uptake. Cell. 2009;138:1064–1066. doi:10.1016/j.cell.2009.09.005.
- Hu HC, Wang YY, Tsay YF. AtCIPK8, a CBL-interacting protein kinase, regulates the low-affinity phase of the primary nitrate response. Plant J. 2009;57:264–278. doi:10.1111/j.1365-313X.2008.03685.x.
- Tuteja N, Sopory SK. Plant signaling in stress: g-protein coupled receptors, heterotrimeric G-proteins and signal coupling via phospholipases. Plant Signal Behav. 2008:379–386. doi:10.4161/psb.3.2.5303.
- Rupwate SD, Rajasekharan R. Plant phosphoinositide-specific phospholipase C: an insight. Plant Signal Behav. 2012;7:1281–1283. doi:10.4161/psb.21436.
- Singh A, Bhatnagar N, Pandey A, Pandey GK. Plant phospholipase C family: regulation and functional role in lipid signaling. Cell Calcium. 2015;58:139–146. doi:10.1016/j.ceca.2015.04.003.
- Schulz P, Herde M, Romeis T. Calcium-dependent protein kinases: hubs in plant stress signaling and development. Plant Physiol. 2013;163(2):523–530. doi:10.1104/pp.113.222539.
- Kudla J, Batistic O, Hashimoto K. Calcium signals: the lead currency of plant information processing. Plant Cell. 2010;22:541–563. doi:10.1105/tpc.109.072686.
- Boudsocq M, Sheen J. CDPKs in immune and stress signaling. Trends Plant Sci. 2013;18(1):30–40. doi:10.1016/j.tplants.2012.08.008.
- Shi S, Li S, Muhammad A, Mao J, Xu D, Ullah Z, Liu GS, Wang Q, Liu HB. The Arabidopsis calcium-dependent protein kinases (CDPKs) and their roles in plant growth regulation and abiotic stress responses. Int J Mol Sci. 2018;19(7):1900. doi:10.3390/ijms19071900.
- Liu KH, Niu Y, Konishi M, Wu Y, Du H, Sun CH, Li L. Discovery of nitrate-cpk-nlp signalling in central nutrient-growth networks. Nature. 2017;545(7654):311–316. doi:10.1038/nature22077.
- Zhang H, Forde BG. An arabidopsis mads box gene that controls nutrient-induced changes in root architecture. Science. 1998;279(5349):407–409. doi:10.1126/science.279.5349.407.
- Gan Y, Bernreiter A, Filleur S, Abram B, Forde BG. Overexpressing the ANR1 MADS-Box gene in transgenic plants provides new insights into its role in the nitrate regulation of root development. Plant Cell Physiol. 2012;53(6):1003–1016. doi:10.1093/pcp/pcs050.
- Zhang X, Cui Y, Yu M, Su B, Gong W, Baluška F, Komis G, Šamaj J, Shan X, Lin J. Phosphorylation-mediated dynamics of nitrate transceptor NRT1.1 regulate auxin flux and nitrate signaling in lateral root growth. Plant Physiol. 2019;181:480–498. doi:10.1104/pp.19.00346.
- Wang C, Zhang W, Li Z, Li Z, Bi Y, Crawford NM, Wang Y. FIP1 plays an important role in nitrate signaling and regulates cipk8 and cipk23 expression in Arabidopsis. Front Plant Sci. 2018;9:593. doi:10.3389/fpls.2018.00593.
- Dodd AN, Kudla J, Sanders D. The language of calcium signaling. Annu Rev Plant Biol. 2010;61:593–620. doi:10.1146/annurev-arplant-070109-104628.
- Mossaid I, Fahrenkrog B. Complex commingling: nucleoporins and the spindle assembly checkpoint. Cells. 2015;4(4):706–725. doi:10.3390/cells4040706.
- Ristova D, Carré C, Pervent M, Medici A, Kim GJ, Scalia D, Ruffel S, Birnbaum KD, Lacombe B, Busch W, et al. Combinatorial interaction network of transcriptomic and phenotypic responses to nitrogen and hormones in the Arabidopsis thaliana root. Sci Sig. 2016;9:rs13. doi:10.1126/scisignal.aaf2768.
- Edel KH, Kudla. Integration of calcium and ABA signaling. Curr Opin in Plant Biol. 2016;33:83–91. doi:10.1016/j.pbi.2016.06.010.
- Efeyan A, Comb WC, Sabatini DM. Nutrient-sensing mechanisms and pathways. Nature. 2015;517:302. doi:10.1038/nature14190.
- Sheen J. Ca2+-dependent protein kinases and stress signal transduction in plants. Science. 1996;274:1900–1902. doi:10.1126/science.274.5294.1900.
- Wang W, Hu B, Li A, Chu C. NRT1.1s in plants: functions beyond nitrate transport. Front Plant Sci. 2020;71(15):4373–4379. doi:10.1093/jxb/erz554.
- Krouk G, Lacombe B, Bielach A, Perrine-Walker F, Malinska K, Mounier E, Hoyerova K, Tillard P, Leon S, Ljung K. Nitrate-regulated auxin transport by NRT1.1 defines a mechanism for nutrient sensing in plants. Dev Cell. 2010;18:927–937. doi:10.1016/j.devcel.2010.05.008.