ABSTRACT
Plant-specific TCP transcription factors play an essential role in plant growth and development. They can regulate leaf curvature, flower symmetry and the synthesis of secondary metabolites. The flavonoids in Ginkgo biloba leaf are one of the main medicinally bioactivate compounds, which have pharmacological and beneficial health effects for humans. In this study, a total of 13 TCP genes were identified in G. biloba, and 5 of them belonged to PCF subclades (GbTCP03, GbTCP07, GbTCP05, GbTCP13, GbTCP02) while others belonged to CIN (GbTCP01, GbTCP04, GbTCP06, GbTCP08, GbTCP09, GbTCP10, GbTCP11, GbTCP12) subclades according to phylogenetic analysis. Numerous cis-acting elements related to various biotic and abiotic signals were predicted on the promoters by cis-element analysis, suggesting that the expression of GbTCPs might be co-regulated by multiple signals. Transcript abundance analysis exhibited that most of GbTCPs responded to multiple phytohormones. Among them, the relative expression levels of GbTCP06, GbTCP11, and GbTCP13 were found to be significantly influenced by exogenous ABA, SA and MeJA application. In addition, a total of 126 miRNAs were predicted to target 9 TCPs (including GbTCP01, GbTCP02, GbTCP04, GbTCP05, GbTCP06, GbTCP08, GbTCP11, GbTCP12, GbTCP13). The correlation analysis between the expression level of GbTCPs and the flavonoid contents showed that GbTCP03, GbTCP04, GbTCP07 might involve in flavonoid biosynthesis in G. biloba. In short, this study mainly provided a theoretical foundation for better understanding the potential function of TCPs in G. biloba.
Introduction
TCP (Teosinte branched1, Cycloidea, Proliferating cell factor 1 and 2) family proteins are specific transcription factors (TFs) that play crucial roles in many developmental processes in plants, including lateral branch elongation, leaf development, hormone biosynthesis, stress response, and circadian rhythm.Citation1, Citation2–5 TCP TFs are named after the following regulators: TB1, which regulates the development of axillary meristem in Zea mays;Citation6 CYC, which controls asymmetry in Antirrhinum majus;Citation7 and PCF1/2, which affect cell proliferation and differentiation in Oryza sativa.Citation8 All TCP proteins contain one highly conservative non-canonical helix-loop-helix bHLH structure that is involved in DNA binding and protein-protein interactions.Citation9 According to differences in structural domains, TCPs can be divided into class I (PCF subclades) and class II (CYC/TB1 and CIN subclades) subfamilies. Compared with class II TCPs in class I have four amino acid deletions and a single TCP domain.Citation8,Citation10 PCF reportedly promoted plant proliferation and growth of plants,Citation11 whereas the members belonging to the CYC clade participate in plant inflorescence development, the TB1 clade are key transposons that regulate apical dominance in maize.Citation12,Citation13 Previous studies on snapdragon fund that the CIN gene could affect leaf shape development by regulating the activity of leaf marginal cells leading to leaf curl and promoting the differentiation of epidermal cells.Citation14,Citation15
Although TCP genes were identified in various plants including grapes, potatoes, soybeans and sorghum,Citation16–19 the functions of TCP genes were mainly systematically investigated in A. thaliana. Among them, AtTCP07 was reported to target the CYCLIND1-1 site and activate its transcription, which affected the development of leaf blades and hypocotyls.Citation20 Overexpression AtTCP08 affected the flowering process by improving the expression of gene FLC.Citation21 Overexpression of AtTCP4 was found to suppress the production of trichome branches by directly activating the hairless inflorescence stem gene GLABROUS INFLORESCENCE STEMS.Citation22 Additional, Yu et al found AtTCP5 inhibited the development of leaf margin serrations by activating the expression of SAW1.Citation23
miRNA is a kind of noncoding RNA with regulatory functions in eukaryotes. According to reports, they were involved in the regulation of gene expression by targeting specific TFs.Citation24 miRNA396 was reported to target the GRF and bHLH genes to affect the leaf development in Arabidopsis.Citation25 Besides, some miRNAs were also funded to targeted TCPs. For instance, TCP2, TCP3, TCP4, TCP10, TCP24 were reportedly regulated by miR319 in Arabidopsis.Citation26 miR164 was reported to target the AtTCP3 to impact the Leaf shape development.Citation27 miR319 promote the regeneration of new buds by targeting AtTCP3 and AtTCP4 to mediate auxin synthesis.Citation28 Additional, Li et al research found that miR319 targeted the expression of TCP and affected the flower development in maize.Citation29 In short, miRNA regulated the expression of genes by sequence complementarity, but the potential regulatory mechanism needs further study.Citation30
Ginkgo biloba L. is a deciduous tree and is one of the oldest extant plant species considered a “living fossil.”31 G. biloba leaf extract, of which the main active ingredients belonged to flavonoids, was proven to have antioxidant, anti-cancer, anti-inflammatory, and other medicinal activities, and could be used to treat cardiovascular diseases.Citation31–34 Flavonoid biosynthesis in G. biloba was initiated by the precursor substance phenylpropanoid.Citation35 The content reportedly can be regulated by moderating the expression of synthesis-related structural genes, such as CHI, CHS, and F3H.Citation36 Some TFs also allegedly affected flavonoid biosynthesis. For instance, MYB4 inhibited flavonoid biosynthesis by suppressing the expression of the Arogenate Dehydratase 6 gene.Citation37 While TCPs were also found to involve in the regulation of flavonoid biosynthesis. AtTCP15 inhibited the expression of anthocyanin biosynthesis genes to suppress the synthesis of anthocyanins under strong light. AtTCP3 was reported to interact with R2R3-MYBs to increase flavonoid accumulation.Citation38,Citation39 Besides, CsTCPs were also reported to regulate the transcription of anthocyanin synthase and anthocyanidin reductase thus influence the biosynthesis of catechin.Citation40 However, there is no study on the functional analysis of TCPs regulating flavonoid biosynthesis in G. biloba.
In this study, 13 novel TCP genes were identified in G. biloba. Systematic analysis and prediction on the structures and functions of TCPs were performed, including the physicochemical properties, gene structures, motif compositions, evolutionary relationships, cis-acting elements, correlation with, and miRNAs analysis. Quantitative real-time polymerase chain reaction (qRT-PCR) was conducted to detect the response of GbTCPs to different hormone treatments. The correlation analysis of gene expression and flavonoid content found that GbTCP03, GbTCP04, GbTCP07 might be correlated with flavonoid synthesis. This study will provide the classification and potential functions of GbTCPs and pave the way for the verification of TCPs involved in flavonoid regulation.
Materials and methods
Identification and physicochemical properties of TCP proteins from G. biloba
The whole protein sequence of G. biloba was downloaded from the online website GIGADB (http://gigadb.org/dataset/100613).Citation41 The transcriptome sequence database was obtained from NCBI under the Bioproject accession number PRJNA485059.Citation34 The TCP protein of A. thaliana was downloaded from the Plant Transcription Factor Database v5.0 (http://planttfdb.cbi.pku.edu.cn/) and used as a source for a local BLAST search against the G. biloba protein database. The Hidden Markov model file of TCP domain (PF03634) was downloaded from the Pfam database (https://pfam.xfam.org) and utilized as a resource to screen proteins that contain the corresponding domain in G. biloba. The conserved structures of the screened TCP proteins were characterized through the website CDD-Search (https://www.ncbi.nlm.nih.gov/Structure/cdd/wrpsb.cgi) and SMART (https://smart.embl-heidelberg.de/). The physicochemical properties of these TCP proteins, such as molecular weight (MW), isoelectric point (pI), and total protein length, were analyzed through the website EXPASy (https://web.expasy.org/protparam/).
Chromosome location and subcellular localization prediction
The distribution of GbTCPs on the 12 chromosomes of G. biloba was mapped by TBtools.Citation42 The TCP proteins were uploaded to WOLF PSORT (http://www.genscript.com/psort.html) to perform subcellular localization prediction.Citation43
Multiple alignments, gene structure, and conserved motif analysis of G. biloba
Multiple alignments of the TCP proteins were performed using Clustalx 2.1 with default parameters values. The gene structures of TCPs were predicted by GSDS (https://gsds.cbi.pku.edu.cn/index.php).Citation44 The conserved motifs of the GbTCP proteins were analyzed by the online tool MEME5.3.2 (http://meme-suite.org/tools/meme) and visualized by TBtools.
Cis-elements of analysis TCP promoters
The 2,000 bp sequence upstream of the start codon of the GbTCPs was screened from the genomic DNA database of G. biloba, and the cis-acting elements were predicted using PlantCARE (http://bioinformatics.psb.ugent.be/webtools/plantcare/html/).Citation45
Phylogenetic analysis
The evolutionary relationship of GbTCPs and other plants was clarified by obtaining the sequence of 33 AtTCPs and 23 OsTCPs from the Plant Transcription Factor Database v5.0. Multiple sequence alignments were conducted by ClustalX 2.1. An unrooted phylogenetic analysis was conducted via the neighbor-joining method by MEGA-X V.7, and bootstrap values were calculated with 1,000 replicates.Citation46
Prediction of TCP target miRNA
Based on the transcription database of G. biloba,Citation47 miRNAs targets GbTCP were screened using one plugin of TBtools with gene id as the index. The screened miRNAs were then predicted using the Omicshare platform (https://www.omicshare.com).
Correlation analysis between GbTCPs expression level and flavonoids content
All gene expression information was achieved from our previous transcriptome data of G. biloba (root, stem, immature leaf, mature leaf, microstrobilus, ovulate strobilus, immature fruit, and mature fruit) with three biological replicates for each organ.Citation34 The expression levels of the GbTCP genes were calculated by the value of FPKM.Citation48 Correlation between GbTCPs and flavonoid content was analyzed using OriginPro 2021 software with a significant correlation indicated by < 0.05.Citation49
Plant material and hormone treatments
The seedlings of G. biloba (one-year-old) were grown in the greenhouse of Yangtze University (30.35°N, 112.14°E) at 25°C with 14 h of light. The leaves were sprayed with 0.1 mmol·L−Citation1 methyl jasmonate (MeJA), 0.1 mmol·L−Citation1 abscisic acid (ABA), and 10 mmol·L−Citation1 salicylic acid (SA).Citation50 Leaves treated with deionized water served as the control. The experiment was repeated three times, and each treatment consisted of 10 seedlings with three biological repetitions. The leaves were immediately frozen in liquid nitrogen and stored in an ultralow temperature freezer (Haier, China) for further analysis.
Expression of GbTCPs gene under different hormone stress
Total RNA was extracted using the Plant RNA extraction kit (TaKaRa, China) following the provided protocol. RNA was quantified and qualified using the Nanodrop One spectrophotometer (Thermo Fisher Scientific, USA). The qualified RNA was reverse-transcribed into cDNA by using HiScript II 1st Strand cDNA synthesis kit (Vazyme, China). The relative quantitative primers (Supplemental Table S1) were designed using the online tool PrimerQuest (https://sg.idtdna.com/). For qRT-PCR, glyceraldehyde-3-phosphate dehydrogenase was used as the reference gene.Citation51 The qRT-PCR reaction was performed using ChamQ Universal SYBR qPCR Master Mix (Vazyme, China) according to the instruction. Each sample was biologically and technically repeated three times. Statistical analysis was performed using SPSS 23,Citation52 and the relative expression levels of GbTCPs were calculated via the 2−ΔΔCt method.Citation53
Results
Identification, chromosome distribution, and subcellular localization of GbTCPs
A total of 13 GbTCPs were identified from the G. biloba genome database by using the TCP sequence of A. thaliana by local BLAST and HMMER. The GbTCP members were named according to their order and locations on the chromosome, that is GbTCP01-GbTCP13 (Supplemental Table S2). As shown in , the length of the GbTCP proteins ranged from 202 aa to 716 aa, with an average length of 524 aa. The MW of these GbTCPs ranged from 22.18 kDa to 79.20 kDa. The pI of the predicted GbTCPs was between 5.84 and 9.31. Prediction results of subcellular localization by using WOLF PSORT indicated that all of the 13 GbTCP proteins were localized in the nucleus. Moreover, these GbTCPs were unevenly distributed on 6 chromosomes (): 5 GbTCPs were distributed on chromosome 12, 3 GbTCPs were distributed on chromosome 10, 2 GbTCPs were distributed on chromosome 1 and only 1 GbTCP was distributed in chromosomes 7, 9 and 11.
Table 1. The information of GbTCP proteins in G. biloba.
Multiple alignments of GbTCPs
The sequence logo () and multiple alignments () results indicated that all of the GbTCPs contained a noncanonical helix-loop-helix bHLH structure consisting of 59 amino acid residues, and they are highly conservative in basic and two helix regions. The basic region consists of 15 or 19 amino acids and most of which were alkaline amino acids, such as arginine (R), histidine (H), and lysine (K). All Class I type TCPs have four amino acid deletions in the basic region compared to the Class II type TCPs. Both Class I and Class II proteins have repeated tryptophan (W) and leucine (L) events in the helix II region and are highly conservative in helix I, alanine (A), and leucine (L). Totally, the proteins in both classes differed in overall amino acid composition in helix I, helix II, and loop regions.
Figure 2. Multiple sequence alignment of GbTCPs. (a) The sequence logo was constructed by WebLogo, the height of each letter representing the corresponding amino acid the frequency of occurrence. (b) Multiple sequence alignment was performed with Clustalx 2.1. The red part denotes highly conserved basic amino acids, the blue part indicates hydrophobic amino acids, the purple part indicates acidic amino acids and the green part represents basic amino acids.
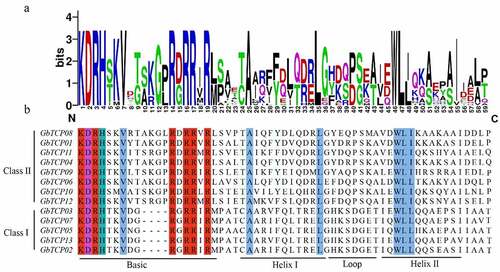
Phylogenetic analysis of GbTCP family
To clarify the evolutionary relationship of TCPs in G. biloba, A. thaliana, and O. sativa, an unrooted phylogenetic was constructed based on multiple sequence alignment of 13 G. biloba, 33 A. thaliana, and 23 O. sativa TCPs (Supplemental Table S3). As shown in , all TCPs were divided into PCF, CYC/TB1, and CIN subclades, according to the differences conserved domains. Of which 5 G. biloba, 15 A. thaliana, and 10 O. sativa TCPs were classified into PCF subclade, accounting for 43.47% of the total TCPs; 8 TCPs of G. biloba, 13 TCPs of A. thaliana, and 10 TCPs of O. sativa were classified into CIN subclade, accounting for 44.92% of the total TCPs; additionally, 0, 5, and 3 TCPs of G. biloba, A. thaliana, and O. sativa were clustered into the CYC/TB1 subclade respectively, accounting for 11.59% of the total TCPs.
Figure 3. The phylogenetic analysis of TCPs among G. biloba, A. thaliana, and O. sativa. The unrooted phylogenetic tree was constructed by the neighbor-joining (NJ) method with 1000 bootstrap replicates. The blue circle indicates the O. sativa TCP family, the red pentagram indicates the G. biloba TCP family, and the purple triangle indicates the A. thaliana TCP family. The blue pink and lycopene ring indicate PCF, CYC/TB1, and CIN subclades, respectively.
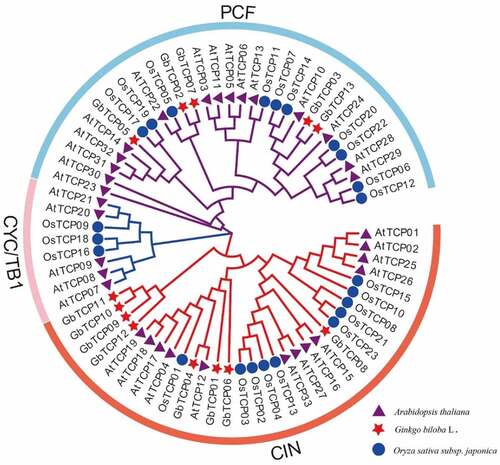
Gene structural and conserved motif analysis of TCPs in G. biloba
Exon-intron regions of GbTCPs were analyzed using the online tool GSDS to gain insights into the evolution of their gene structures (). The gene in Class I has only one exon, five genes in Class II have 1–5 exons, and GbTCP09 and GbTCP12 contain five exons and four introns. These results suggested that the exon-intron distribution of the GbTCPs was similar in the same classification. Twenty putative conservative motifs of GbTCPs were identified and named motifs 1–20 using the MEME program (), of which motifs 1 and 2 were identified in all of the GbTCPs. The number of motifs on each protein ranged from 5 to 11, of which GbTCP03 contains the maximum number of 11 motifs, GbTCP04, GbTCP05, GbTCP06, and GbTCP09 have the least number of motifs with 5. Additionally, motifs 4 and 9 existed in almost all Class I type GbTCPs except for GbTCP05, whereas motif 3 and 7 existed in most Class II type GbTCPs.
Figure 4. The conserved motifs and gene structure identified in GbTCP. (a) The classify and gene structure of GbTCPs. Exons are labeled using the orange box, introns are labeled using black lines. (b) Conserved motifs analysis of GbTCPs. Each color represents a motif and the number represents the classify of the motif in the protein sequence.
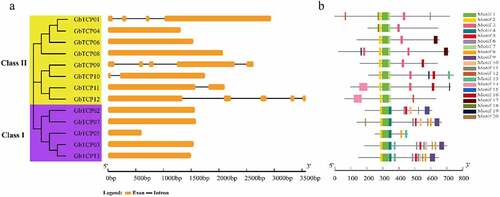
Cis-acting elements in the promoters of TCPs in G. biloba
The promoter sequence of 2,000 bp upstream of the coding region of GbTCPs was extracted for the prediction of cis-acting elements. As shown in Supplemental Figure S1 and Table S4, a total of 20 type cis-acting elements were discovered in the promoter regions of GbTCPs. These cis-acting elements were predicted to be related to transcription, various hormonal and stress responses, and development. In particular, the cis-acting elements related to plant growth and development, such as circadian rhythm, seed and endosperm-specific expression, and developmental regulatory were found in the promoter of GbTCP02, GbTCP05, GbTCP06, GbTCP07, GbTCP08, and GbTCP12. The cis-acting elements in response to hormones, such as ABA, SA, MeJA, auxin (AUX), and gibberellic acid (GA) were identified in GbTCP01 ~ GbTCP13. Abiotic stress elements responding to drought induction, anaerobic induction, and low temperature were identified in GbTCP01, GbTCP02, GbTCP06, GbTCP07, and GbTCP12. The Zein metabolism regulation element was identified in GbTCP01, GbTCP02, GbTCP03, GbTCP05, GbTCP07, GbTCP09, and GbTCP10. Furthermore, MYBHVL binding sites were identified in the promoter regions of GbTCP01 and GbTCP10.
Expression patterns of GbTCPs under different exogenous hormone treatments
The effects of exogenous hormone treatment on the gene expression levels of GbTCPs in leaves were evaluated using qRT-PCR. Mostly GbTCPs were expressed under various hormone treatments, while the expression of GbTCP09, GbTCP10, and GbTCP12 was not detected in all the hormone-treated samples. The available data were analyzed and shown in . Under 0.1 mmol·L−Citation1 ABA treatment, most of the GbTCPs showed a decreasing trend and then gradually increased, but a few of them displayed a continuously decreasing trend. Among them, the expression levels of GbTCP06, GbTCP11, and GbTCP13 at 3 h were higher than those at 0 h. Whereas the expression levels of GbTCP02, GbTCP05, and GbTCP07 at 3 h were lower than those at 0 h. Under 10 mmol·L−Citation1 SA treatment, the expression level of GbTCPs showed a downward trend at 0–12 h but slightly increased at 48 h. After being treated with 0.1 mmol·L−Citation1 MeJA, the expression levels of GbTCP01, GbTCP04, and GbTCP08 decreased initially and subsequently reached the peak at 48 h. Besides, the relative expression levels of GbTCP03, GbTCP05, GbTCP06, and GbTCP13 decreased 3 h after MeJA treatment and overall displayed a W-shaped trend. Interestingly, the expression level of GbTCP07 was substantially induced at 3 h, which was different from other GbTCPs.
Figure 5. Expression pattern of GbTCP genes under different hormone treatments which was included 10 mmol L−1 SA, 0.1 mmol L−1 ABA, 0.1 mmol L−1 MeJA. One-year-old G. biloba leaves were used as experimental materials and samples were collected at 0, 3, 6, 12, 24, and 48 h after different hormone treatments. Values represent means ± standard deviation of triplicates and the letters indicate significance were reckoned by Tukey and Duncan test (p< 0.05).
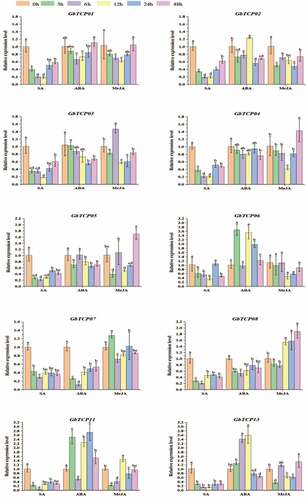
Tissue-specific expression pattern of GbTCPs and correlation analysis with flavonoids
Based on our previous transcriptome data, the FPKM data of 12 available GbTCPs was selected for tissue-specific expression analysis. As shown in , most of the GbTCPs were expressed in different tissues. GbTCP01 was mainly expressed in young leaves, mature leaves, microstrobilus, young fruits, and mature fruits, whereas GbTCP07 was expressed at different development stages and tissues of G. biloba, especially in stems and mature leaves. In addition, GbTCP08 was primarily expressed in roots and microstrobilus, and GbTCP10 and GbTCP12 were only expressed in the shoot. Curiously, the expression of GbTCP09 was not detected in all the examined tissues. The correlation analysis showed that the expression level of GbTCP03, GbTCP04, and GbTCP07 were positively correlated with flavonoid content ().
Figure 6. Correlation analysis of GbTCPs expression level and flavonoid content in different tissues of G.biloba. The R, S, IL, ML, M, OS, IF, MF represents (root, stem, immature leaf, mature leaf, microstrobilus, ovalate strobilus, immature fruit, mature fruit) respectively, the expression level of GbTCPs is indicated on the left and the flavonoids content are displayed on the right all experiments were repeated three times. Letter denote significant difference (P< 0.05).
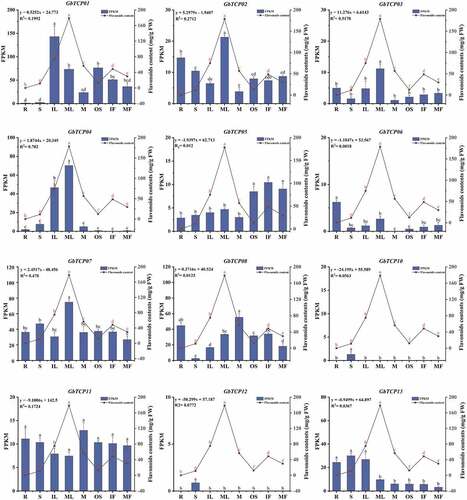
Predicted miRNA-based Network
A miRNA regulation network was constructed based on the miRNA data of five tissues (roots, stems, leaves, microstrobilus, and ovulate strobilus) obtained in our previous study.Citation47 As shown in , the miRNA-based network comprised 135 nodes (126 miRNAs and 9 TCP TFs) and 126 directed edges. Among which, 66 miRNAs were identified to target GbTCP12, accounting for 51.97% of the total miRNAs. Moreover, 29 miRNAs were identified to target GbTCP08, accounting for 22.83% of the total miRNAs. GbTCP13 was targeted by 12 miRNAs, whereas GbTCP02 and GbTCP04 were regulated by 3 miRNAs. GbTCP08 and GbTCP13 could be targeted and regulated by 8 common miRNAs (e.g., miR_2239, miR_2669, miR_2910, miR_2923, miR_3074, miR_3312, miR_3317, and miR_3441). Both GbTCP08 and GbTCP01 could be targeted by 5 miRNAs (e.g., miR_3288, miR_372, miR_1106, miR_1804, and miR_2294). Both GbTCP12 and GbTCP13 could be regulated by the same miRNA (e.g., miRNA_1249). Furthermore, 37 miRNAs among the 126 miRNAs were annotated with family names (Supplemental Table S5), such as miRNA319, miRNA159, miRNA2600, and miRNA2588.
Discussion
Phylogenetic and structure of the TCP family in G. biloba
In recent years, the completion of whole-genome sequences provides us an opportunity to comparatively identify and analyze TCP genes in G. biloba. In the present study, 13 TCPs were identified from G. biloba genome, and these genes were unequally distributed in six chromosomes. Based on the results of multiple alignments, GbTCPs could be divided into Class I (PCF) and Class II (CIN) types. The number of genes distributed in Class II was found to be higher than that in Class I (). Class I genes are primarily involved in the regulation of cell proliferation and differentiation, whereas Class II subfamily genes, especially the CIN subclade, chiefly affect leaf shape development.Citation11,Citation14 Therefore, we speculated that those GbTCPs may play multiple roles in plant growth and development. Curiously, phylogenetic analysis indicated that the GbTCP genes do not have TB1/CYC subclades, which regulate the development of meristems and affect the symmetry of flowers.Citation6,Citation7 Besides, Liu et al confirmed that CYC is recent and originated from CIN in angiosperms while G. biloba belong to ancient gymnosperms.Citation54,Citation55 Therefore, we speculated that the difference in evolutionary time may cause this result. In addition, GbTCP09-12 was found to distribute in the same chromosome and adjacent to each other. Combining the cluster of those genes in the phylogenetic tree, it is referred that gene duplication or doubling may have occurred during the evolution. Additionally, 8 GbTCPs were classified into the CIN subclade, which is reportedly involved in the regulation of leaves, trichomes, and flowering time.Citation56 Subcellular localization predictions found that all of the GbTCPs was expressed in the nucleus (), these results are consistent with earlier reports in Fragaria vesca and Phyllostachys edulis.Citation57,Citation58 Analysis of tissue expression patterns showed that most of GbTCPs were highly expressed in mature leaves while weakly expressed in roots, young leaves, and stems. This result was consistent with the dynamic expression patterns of TCP genes reported in snapdragon.Citation14
Multi cis-acting elements exist in the promoter of GbTCPs
The analysis of cis-acting elements suggested that the expression of GbTCPs might be co-regulated by multiple signals. According to the prediction results, three types of elements were identified from the promoter regions of GbTCPs. The first type of cis-acting elements which included circadian rhythm, and endosperm-specific expression element. Circadian rhythm was an endogenous process that could regulate the stomatal opening, rate of photosynthesis, carbohydrate metabolism, and initiation of flowering.Citation59,Citation60 We speculated that these circadian rhythm response elements may serve multiple functions in G.biloba. The second type of cis-acting elements was predicted to be associated with phytohormones, such as ABA, SA, and MeJA. Besides, the third type of cis-acting elements was predicted to be linked to abiotic stress response, such as drought induction, anaerobic induction, and low temperature, consistent with previous findings in cassava.Citation61 Therefore, we speculated that these GbTCPs may play roles in resisting various stress.
Hormonal pathways associated with TCPs expression in G. biloba
Recently, several studies reported that the hormonal pathways are related to the expression of TCPs.Citation62,Citation63 AtTCP01 mediates AtDWF4 transcription and promotes brassinosteroid biosynthesis.Citation64 FvTCP9 affected ABA synthesis by regulating the expression levels of FaNCED1, FaPYR1, FaSnRK2, and FaABI5 to promoted fruit development.Citation57 Besides, it was reported that strigolactone affects leaf development by activating TCP1 expression in A. thaliana.Citation65 In this study, the expression of GbTCPs was differentially expressed in G. biloba leaves under ABA, SA, and MeJA treatment. After ABA treatment, the expression levels of most GbTCPs showed an upward trend. By comparison, after treatment with SA and MeJA, the expression levels of most GbTCPs showed a downward trend, which was similar to the reports by Feng et al.Citation18 Previous study showed that OsTCP19 can improve ABA signal transduction by promoting the expression of ABA INSENSITIVE4.Citation66 In the present study, the expression levels of GbTCP06, GbTCP11, and GbTCP13 exhibited an upward trend after ABA treatment, consistent with the study by Liu et al.Citation67 Some reports revealed that TCPs can interact with the SA biosynthetic enzyme gene and enhance its expression.Citation68 Moreover, SA treatment reportedly substantially increases the expression levels of several PeTCPs.Citation67 Curiously, in the present study, the relative expression levels of the GbTCPs showed a downward trend under SA treatment, which might indicate the existence of a negative feedback mechanism in G. biloba. In this study, the expression levels of GbTCP04, GbTCP05, and GbTCP08 showed an upward trend and reached a peak at 48 h under MeJA treatment. Hence, we speculated that TCPs may participate in the regulation of senescence in G. biloba by positively responding to MeJA induction.
Multi miRNAs targeted the GbTCPs in G. biloba
miRNAs are a class of non-coding RNAs present in plants and animals. They participate in the regulation of gene expression by targeting TFs. Most miRNAs, such as miR156-SPL and miR159-MYB, were reported as suppressors of TFs.Citation29,Citation69,Citation70 In recent years, about 8,400 miRNAs have been identified from 121 plants, of which 1,427 miRNAs have been identified in Arabidopsis.Citation71 These miRNAs play an important role in plant growth and development. For instance, miR159, miR319, miR390, and miR399 participate in the regulation of flowering time.Citation72 Furthermore, a minority of miRNA functional in G. biloba has been reported such as miR159 involved in regulating the development of morphophysiological dormancy,Citation73 miR164, miR172, and miR156 involved in regulating the senescence process.Citation74,Citation75 In the present study, 126 miRNAs were predicted to target 9 GbTCP genes, and 38 miRNAs were known for their function. 22 annotated miRNAs were predicted to regulate GbTCP01, and the majority of them were annotated as miRNA319 family. miRNA159 were predicted to target gene GbTCP08, while miRNA172 were predicted to target gene GbTCP02 (). The studies of Palatnik et al found that miRNA319 regulating floral development and leaf senescence by targeting TCP.Citation74 Besides, Gupta et al demonstrated that miR319 regulate the biosynthesis of isoflavones in soybean seeds by targeting GmTCP4.Citation76 Moreover, the upregulation of miR159 and miR319 suppress the expression of TCP genes and delayed the flowering in A. thaliana.Citation74 Therefore, we speculated that miRNA319 and miRNA159 in G. biloba may play similarity function by targeting GbTCPs. In addition, GbTCP13 and GbTCP08 can be targeted by eight identical miRNAs, and GbTCP08 and GbTCP01 can be targeted by five identical miRNAs. Therefore, we inferred that these miRNAs may play different roles in regulating multiple developmental processes in G. biloba.
Candidate GbTCPs involved in flavonoids biosynthesis in G. biloba
Flavonoids are natural plant secondary products that conferred antioxidant ability through hydrophobicity and molecular structure, which defend plants against abiotic and biotic stress.Citation77 Besides, flavonoids were proven one of the main bioactive components of the G. biloba leaf extracts which have been used in treating cardiovascular diseases.Citation75,Citation78,Citation79 Previous studies demonstrated that multiple TFs regulated the activity of key enzymes in the phenylpropanoid biosynthetic pathway to affect flavonoid biosynthesis.Citation36For instance, GbMYBF2 and GbMYBR2 were reported to negatively regulate biosynthesis of flavonoid in G. biloba.Citation80,Citation81 GbbHLH, GbTrihelix, and three GbERFs contributed to flavonoid biosynthesis by regulating the expression of structural genes in the flavonoid biosynthesis pathway.Citation82 In recent years, some studies found that TCPs may also be involved in flavonoid biosynthesis. For instance, AtTCP15 affected anthocyanin accumulation by inhibiting the expression of anthocyanin biosynthesis genes,Citation38 and FvTCP9 promoted anthocyanin accumulation by affecting the expression of genes involved in the ABA signal transduction pathway.Citation57 Additionally, AtTCP3, in combination with the R2R3-MYB protein, promoted flavonoid biosynthesis.Citation39 The interaction of MdTCP46 and MdMYB enhanced the binding ability of MdMYB and the target genes, which affected anthocyanin accumulation in apple.Citation83 In the present study, the correlation analysis of gene expression and flavonoid content indicated that CIN-type GbTCP04, and PCF-type GbTCP03, and GbTCP07 were highly correlated with flavonoid synthesis (), suggesting GbTCP03, GbTCP04, and GbTCP07 may involve in flavonoid biosynthesis in G. bioba. This result was consistent with Yu et al.Citation40 In short, the above conclusions mainly based on data analysis, and further research is required to identify the regulatory mechanism of TCPs in G. biloba.
Conclusion
In this study, a total of 13 GbTCPs were identified from G. biloba. These GbTCPs were unequally distributed on six chromosomes. They were divided into Class I (PCF) and Class II (CIN) according to differences in their conserved domains. Genetic structure analysis showed that TCPs of the same subclade have similar exon and intron distributions. Subcellular localization predictions indicated that all 13 genes are expressed in the nucleus. Cis-acting element prediction indicated that the promoter region of most TFs contains various response elements, such as photoresponse, stress response, and hormone response. Results of exogenous phytohormone treatments showed that the expression pattern of the same gene varies among different phytohormone treatments. The correlation analysis showed that GbTCP03, GbTCP04, and GbTCP07 may be related to the synthesis of flavonoids. In addition, a total of 126 miRNAs were identified to target nine TCPs in G. biloba. Overall, our study provided actual data and a theoretical basis for further studies on the functions of GbTCPs in G. biloba.
Author contribution statement
FX and JBY provided the idea and conception of this study. LY performed the draft writing, database analysis, and chart drawing. XYY has supervised the conduct of the experiment. QWC is responsible for the article revision, software installation, and RNA Extractions. JBY contributes to the writing guidance and article revision. JRZ helped in the bioinformatics analysis. WWZ performed the article revision and the revision of the experiment progress. YLL helped with the example collection and analysis. All author has read and approved the manuscript and publish.
Availability of data and materials
The datasets used and analyzed in this study are available from the corresponding author upon reasonable request.
Supplemental Material
Download Zip (558.5 KB)Disclosure statement
The authors declare no competing interest.
Supplementary material
Supplemental data for this article can be accessed on the publisher’s website
Additional information
Funding
References
- Takeda T, Suwa Y, Suzuki M, Kitano H, Ueguchi-Tanaka M, Ashikari M, Matsuoka M, Ueguchi C. The OsTB1 gene negatively regulates lateral branching in rice. Plant J. 2003;33(3):513–13. doi:10.1046/j.1365-313x.2003.01648.x.
- Bresso EG, Chorostecki U, Rodriguez RE, Palatnik JF, Schommer C. Spatial control of gene expression by miR319-regulated TCP transcription factors in leaf development. Plant Physiol. 2018;176(2):1694–1708. doi:10.1104/pp.17.00823.
- Guo Z, Fujioka S, Blancaflor EB, Miao S, Gou X, Li J. TCP1 modulates brassinosteroid biosynthesis by regulating the expression of the key biosynthetic gene DWARF4 in Arabidopsis thaliana. Plant Cell. 2010;22(4):1161–1173. doi:10.1105/tpc.109.069203.
- Ding S, Cai Z, Du H, Wang H. Genome-wide analysis of TCP family genes in Zea mays L. identified a role for in drought tolerance. Int J Mol Sci. 2019;20(11):2762. doi:10.3390/ijms20112762.
- Giraud E, Ng S, Carrie C, Duncan O, Low J, Lee CP, Van Aken O, Millar AH, Murcha M, Whelan J. TCP transcription factors link the regulation of genes encoding mitochondrial proteins with the circadian clock in Arabidopsis thaliana. Plant Cell. 2010;22(12):3921–3934. doi:10.1105/tpc.110.074518.
- Doebley J, Stec A, Hubbard L. The evolution of apical dominance in maize. Nature. 1997;386(6624):485–488. doi:10.1038/386485a0.
- Luo D, Carpenter R, Copsey L, Vincent C, Clark J, Coen E. Control of organ asymmetry in flowers of antirrhinum. Cell. 1999;99(4):367–376. doi:10.1016/s0092-8674(00)81523-8.
- Kosugi S, Ohashi Y. DNA binding and dimerization specificity and potential targets for the TCP protein family. Plant J. 2002;30(3):337–348. doi:10.1046/j.1365-313x.2002.01294.x.
- Martín-Trillo M, Cubas P. TCP genes: a family snapshot ten years later. Trends Plant Sci. 2010;15(1):31–39. doi:10.1016/j.tplants.2009.11.003.
- Chai W, Jiang P, Huang G, Jiang H, Li X. Identification and expression profiling analysis of TCP family genes involved in growth and development in maize. Physiol Mol Biol Plants. 2017;23(4):779–791. doi:10.1007/s12298-017-0476-1.
- Kosugi S, Ohashi Y. PCF1 and PCF2 specifically bind to cis elements in the rice proliferating cell nuclear antigen gene. Plant Cell. 1997;9(9):1607–1619. doi:10.1105/tpc.9.9.1607.
- Tähtiharju S, Rijpkema AS, Vetterli A, Albert VA, Teeri TH, Elomaa P. Evolution and diversification of the CYC/TB1 gene family in asteraceae–a comparative study in gerbera (Mutisieae) and sunflower (Heliantheae). Mol Biol Evol. 2012;29(4):1155–1166. doi:10.1093/molbev/msr283.
- Studer A, Zhao Q, Ross-Ibarra J, Doebley J. Identification of a functional transposon insertion in the maize domestication gene tb1. Nat Genet. 2011;43(11):1160–1163. doi:10.1038/ng.942.
- Nath U, Crawford BCW, Carpenter R, Coen E. Genetic control of surface curvature. Science. 2003;299(5611):1404–1407. doi:10.1126/science.1079354.
- Crawford BCW, Nath U, Carpenter R, Coen ES. CINCINNATA controls both cell differentiation and growth in petal lobes and leaves of antirrhinum. Plant Physiol. 2004;135(1):244–253. doi:10.1104/pp.103.036368.
- Jiu S, Xu Y, Wang J, Wang L, Wang S, Ma C, Guan L, Abdullah M, Zhao M, Xu W, et al. Genome-wide identification, characterization, and transcript analysis of the TCP transcription factors in Vitis vinifera. Front Genet. 2019;10:1276. doi:10.3389/fgene.2019.01276.
- Bao S, Zhang Z, Lian Q, Sun Q, Zhang R. Evolution and expression of genes encoding TCP transcription factors in Solanum tuberosum reveal the involvement of StTCP23 in plant defence. BMC Genet. 2019;20(1):91. doi:10.1186/s12863-019-0793-1.
- Feng Z-J, Xu S-C, Liu N, Zhang G-W, Hu Q-Z, Gong Y-M. Soybean TCP transcription factors: Evolution, classification, protein interaction and stress and hormone responsiveness. Plant Physiol Biochem. 2018;127:129–142. doi:10.1016/j.plaphy.2018.03.020.
- Francis A, Dhaka N, Bakshi M, Jung K-H, Sharma MK, Sharma R. Comparative phylogenomic analysis provides insights into TCP gene functions in sorghum. Sci Rep. 2016;6(1):38488. doi:10.1038/srep38488.
- Zhang G, Zhao H, Zhang C, Li X, Lyu Y, Qi D, Cui Y, Hu L, Wang Z, Liang Z, et al. TCP7 functions redundantly with several Class I TCPs and regulates endoreplication in Arabidopsis. J Integr Plant Biol. 2019;61(11):1151–1170. doi:10.1111/jipb.12749.
- Wang X, Xu X, Mo X, Zhong L, Zhang J, Mo B, Kuai B. Overexpression of TCP8 delays Arabidopsis flowering through a FLOWERING LOCUS C-dependent pathway. BMC Plant Biol. 2019;19(1):534. doi:10.1186/s12870-019-2157-4.
- Vadde BVL, Challa KR, Nath U. The TCP4 transcription factor regulates trichome cell differentiation by directly activating GLABROUS INFLORESCENCE STEMS in Arabidopsis thaliana. Plant J. 2018;93(2):259–269. doi:10.1111/tpj.13772.
- Yu H, Zhang L, Wang W, Tian P, Wang W, Wang K, Gao Z, Liu S, Zhang Y, Irish VF, et al. TCP5 controls leaf margin development by regulating KNOX and BEL-like transcription factors in Arabidopsis. J Exp Bot. 2021;72(5):1809–1821. doi:10.1093/jxb/eraa569.
- Chakraborty A, Viswanath A, Malipatil R, Rathore A, Thirunavukkarasu N. Structural and functional characteristics of mirnas in five strategic millet species and their utility in drought tolerance. Front Genet. 2020;11:608421. doi:10.3389/fgene.2020.608421.
- Giacomelli JI, Weigel D, Chan RL, Manavella PA. Role of recently evolved miRNA regulation of sunflower HaWRKY6 in response to temperature damage. New Phytol. 2012;195(4):766–773. doi:10.1111/j.1469-8137.2012.04259.x.
- Lopez JA, Sun Y, Blair PB, Mukhtar MS. TCP three-way handshake: linking developmental processes with plant immunity. Trends Plant Sci. 2015;20(4):238–245. doi:10.1016/j.tplants.2015.01.005.
- Koyama T, Mitsuda N, Seki M, Shinozaki K, Ohme-Takagi M. TCP transcription factors regulate the activities of ASYMMETRIC LEAVES1 and miR164, as well as the auxin response, during differentiation of leaves in Arabidopsis. Plant Cell. 2010;22(11):3574–3588. doi:10.1105/tpc.110.075598.
- Yang W, Choi M-H, Noh B, Noh Y-S. De novo shoot regeneration controlled by HEN1 and TCP3/4 in Arabidopsis. Plant Cell Physiol. 2020;61(9):1600–1613. doi:10.1093/pcp/pcaa083.
- Li Z, An X, Zhu T, Yan T, Wu S, Tian Y, Li J, Wan X. Discovering and constructing ceRNA-miRNA-Target gene regulatory networks during anther development in maize. Int J Mol Sci. 2019;20(14):3480. doi:10.3390/ijms20143480.
- Yu Y, Jia T, The CX. ‘how’ and ‘where’ of plant microRNAs. New Phytol. 2017;216(4):1002–1017. doi:10.1111/nph.14834.
- Karabin M, Hudcova T, Jelinek L, Dostalek P. Biotransformations and biological activities of hop flavonoids. Biotechnol Adv. 2015;33(6):1063–1090. doi:10.1016/j.biotechadv.2015.02.009.
- Bouaziz M, Grayer RJ, Simmonds MSJ, Damak M, Sayadi S. Identification and antioxidant potential of flavonoids and low molecular weight phenols in olive cultivar chemlali growing in Tunisia. J Agric Food Chem. 2005;53(2):236–241. doi:10.1021/jf048859d.
- Mahmoud AM, Hernández Bautista RJ, Sandhu MA, Hussein OE. Beneficial effects of citrus flavonoids on cardiovascular and metabolic health. Oxid Med Cell Longev. 2019;2019:5484138. doi:10.1155/2019/5484138.
- Ye J, Cheng S, Zhou X, Chen Z, Kim SU, Tan J, Zheng J, Xu F, Zhang W, Liao Y, et al. A global survey of full-length transcriptome of Ginkgo biloba reveals transcript variants involved in flavonoid biosynthesis. Ind Crops Prod. 2019;139:111547. doi:10.1016/j.indcrop.2019.111547.
- Dong N-Q, Lin H-X. Contribution of phenylpropanoid metabolism to plant development and plant-environment interactions. J Integr Plant Biol. 2021;63(1):180–209. doi:10.1111/jipb.13054.
- Shih CH, Chu H, Tang LK, Sakamoto W, Maekawa M, Chu IK, Wang M, Lo C. Functional characterization of key structural genes in rice flavonoid biosynthesis. Planta. 2008;228(6):1043–1054. doi:10.1007/s00425-008-0806-1.
- Wang X-C, Wu J, Guan M-L, Zhao C-H, Geng P, Arabidopsis ZQ. MYB4 plays dual roles in flavonoid biosynthesis. Plant J. 2020;101(3):637–652. doi:10.1111/tpj.14570.
- Viola IL, Camoirano A, Gonzalez DH. Redox-dependent modulation of anthocyanin biosynthesis by the TCP transcription factor TCP15 during exposure to high light intensity conditions in Arabidopsis. Plant Physiol. 2016;170(1):74–85. doi:10.1104/pp.15.01016.
- Li S, Zachgo S. TCP3 interacts with R2R3-MYB proteins, promotes flavonoid biosynthesis and negatively regulates the auxin response in Arabidopsis thaliana. Plant J. 2013;76(6):901–913. doi:10.1111/tpj.12348.
- Yu S, Li P, Zhao X, Tan M, Ahmad MZ, Xu Y, Tadege M, Zhao J. CsTCPs regulate shoot tip development and catechin biosynthesis in tea plant (Camellia sinensis). Hortic Res. 2021;8(1):104. doi:10.1038/s41438-021-00538-7.
- Guan R, Zhao Y, Zhang H, Fan G, Liu X, Zhou W, Shi C, Wang J, Liu W, Liang X, et al. Draft genome of the living fossil Ginkgo biloba. Gigascience. 2016;5(1):49. doi:10.1186/s13742-016-0154-1.
- Chen C, Chen H, Zhang Y, Thomas HR, Frank MH, He Y, Xia R. TBtools: an integrative toolkit developed for interactive analyses of big biological data. Mol Plant. 2020;13(8):1194–1202. doi:10.1016/j.molp.2020.06.009.
- Horton P, Park K-J, Obayashi T, Fujita N, Harada H, Adams-Collier CJ, Nakai K. WoLF PSORT: protein localization predictor. Nucleic Acids Res. 2007;35(Web Server issue):W585–W587. doi:10.1093/nar/gkm259.
- Hu B, Jin J, Guo A-Y, Zhang H, Luo J, Gao G. GSDS 2.0: an upgraded gene feature visualization server. Bioinformatics. 2015;31(8):1296–1297. doi:10.1093/bioinformatics/btu817.
- Lescot M, Déhais P, Thijs G, Marchal K, Moreau Y, Van De Peer Y, Rouzé P, Rombauts S. PlantCARE, a database of plant cis-acting regulatory elements and a portal to tools for in silico analysis of promoter sequences. Nucleic Acids Res. 2002;30(1):325–327. doi:10.1093/nar/30.1.325.
- Saitou N, Nei M. The neighbor-joining method: a new method for reconstructing phylogenetic trees. Mol Biol Evol. 1987;4(4):406–425. doi:10.1093/oxfordjournals.molbev.a040454.
- Ye J, Zhang X, Tan J, Xu F, Cheng S, Chen Z, Zhang W, Liao Y. Global identification of Ginkgo biloba microRNAs and insight into their role in metabolism regulatory network of terpene trilactones by high-throughput sequencing and degradome analysis. Ind Crops Prod. 2020;148:112289. doi:10.1016/j.indcrop.2020.112289.
- Zhou X, Liao Y, Kim S-U, Chen Z, Nie G, Cheng S, Ye J, Xu F. Genome-wide identification and characterization of bHLH family genes from Ginkgo biloba. Sci Rep. 2020;10(1):13723. doi:10.1038/s41598-020-69305-3.
- Zhang L, Xu D, Huang Y, Zhu X, Rui M, Wan T, Zheng X, Shen Y, Chen X, Ma K, et al. Structural and functional characterization of deep-sea thermophilic bacteriophage GVE2 HNH endonuclease. Sci Rep. 2017;7(1):42542. doi:10.1038/srep42542.
- Meng X, Xu F, Song Q, Ye J, Liao Y, Zhang W. Isolation, characterization and functional analysis of a novel 3-hydroxy-3-methylglutaryl-coenzyme A synthase gene (GbHMGS2) from Ginkgo biloba. Acta Physiol Plant. 2018;40(4):72. doi:10.1007/s11738-018-2650-7.
- Jansson S, Meyer-Gauen G, Cerff R, Martin W. Nucleotide distribution in gymnosperm nuclear sequences suggests a model for GC-content change in land-plant nuclear genomes. J Mol Evol. 1994;39(1):34–46. doi:10.1007/BF00178247.
- Ye J, Mao D, Cheng S, Zhang X, Tan J, Zheng J, Xu F. Comparative transcriptome analysis reveals the potential stimulatory mechanism of terpene trilactone biosynthesis by exogenous salicylic acid in Ginkgo biloba. Ind Crops Prod. 2020;145:112104. doi:10.1016/j.indcrop.2020.112104.
- Schmittgen TD, Livak KJ. Analyzing real-time PCR data by the comparative C(T) method. Nat Protoc. 2008;3(6):1101–1108. doi:10.1038/nprot.2008.73.
- Liu -M-M, Wang -M-M, Yang J, Wen J, Guo P-C, Wu Y-W, Ke Y-Z, Li P-F, Li J-N, Du H, et al. Evolutionary and comparative expression analyses of TCP transcription factor gene family in land plants. Int J Mol Sci. 2019;20(14):3591. doi:10.3390/ijms20143591.
- Liu X-M, Cheng S-Y, Ye J-B, Chen Z-X, Liao Y-L, Zhang -W-W, Kim S-U, Xu F. Screening and identification of miRNAs related to sexual differentiation of strobili in Ginkgo biloba by integration analysis of small RNA, RNA, and degradome sequencing. BMC Plant Biol. 2020;20(1):387. doi:10.1186/s12870-020-02598-8.
- Lan J, Qin G. The regulation of CIN-like TCP transcription factors. Int J Mol Sci. 2020;21(12):4498. doi:10.3390/ijms21124498.
- Xie Y-G, Ma -Y-Y, Bi -P-P, Wei W, Liu J, Hu Y, Gou Y-J, Zhu D, Wen Y-Q, Feng J-Y, et al. Transcription factor FvTCP9 promotes strawberry fruit ripening by regulating the biosynthesis of abscisic acid and anthocyanins. Plant Physiol Biochem. 2020;146:374–383. doi:10.1016/j.plaphy.2019.11.004.
- Wei W, Hu Y, Cui M-Y, Han Y-T, Gao K, Feng J-Y. Identification and transcript analysis of the TCP transcription factors in the diploid woodland strawberry Fragaria vesca. Front Plant Sci. 2016;7:1937. doi:10.3389/fpls.2016.01937.
- Orozco-Solis R, Aguilar-Arnal L. Circadian regulation of immunity through epigenetic mechanisms. Front Cell Infect Microbiol. 2020;10:96. doi:10.3389/fcimb.2020.00096.
- Simon NML, Graham CA, Comben NE, Hetherington AM, Dodd AN. The circadian clock influences the long-term water use efficiency of Arabidopsis. Plant Physiol. 2020;183(1):317–330. doi:10.1104/pp.20.00030.
- Lei N, Yu X, Li S, Zeng C, Zou L, Liao W, Peng M. Phylogeny and expression pattern analysis of TCP transcription factors in cassava seedlings exposed to cold and/or drought stress. Sci Rep. 2017;7(1):10016. doi:10.1038/s41598-017-09398-5.
- Li S. TheArabidopsis thaliana TCP transcription factors: a broadening horizon beyond development. Plant Signal Behav. 2015;10(7):e1044192. doi:10.1080/15592324.2015.1044192.
- Zhang S, Zhou Q, Chen F, Wu L, Liu B, Li F, Zhang J, Bao M, Liu G. Genome-wide identification, characterization and expression analysis of TCP transcription factors in Petunia. Int J Mol Sci. 2020;21(18):6594. doi:10.3390/ijms21186594.
- Gao Y, Zhang D, Li J. TCP1 modulates DWF4 expression via directly interacting with the GGNCCC motifs in the promoter region of DWF4 in Arabidopsis thaliana. J Genet Genomics. 2015;42(7):383–392. doi:10.1016/j.jgg.2015.04.009.
- Wang L, Wang B, Yu H, Guo H, Lin T, Kou L, Wang A, Shao N, Ma H, Xiong G, et al. Transcriptional regulation of strigolactone signalling in Arabidopsis. Nature. 2020;583(7815):277–281. doi:10.1038/s41586-020-2382-x.
- Mukhopadhyay P, Tyagi AK. OsTCP19 influences developmental and abiotic stress signaling by modulatingABI4-mediated pathways. Sci Rep. 2015;5(1):9998. doi:10.1038/srep09998.
- Liu H-L, Wu M, Li F, Gao Y-M, Chen F, Xiang Y. TCP transcription factors in Moso Bamboo (Phyllostachys edulis): genome-wide identification and expression analysis. Front Plant Sci. 2018;9:1263. doi:10.3389/fpls.2018.01263.
- Wang X, Gao J, Zhu Z, Dong X, Wang X, Ren G, Zhou X, Kuai B. TCP transcription factors are critical for the coordinated regulation of ISOCHORISMATE SYNTHASE 1 expression in Arabidopsis thaliana. Plant J. 2015;82(1):151–162. doi:10.1111/tpj.12803.
- Lu TX, Rothenberg ME. MicroRNA. J Allergy Clin Immunol. 2018;141(4):1202–1207. doi:10.1016/j.jaci.2017.08.034.
- Song X, Li Y, Cao X, MicroRNAs QY. and their regulatory roles in plant-environment interactions. Annu Rev Plant Biol. 2019;70:489–525. doi:10.1146/annurev-arplant-050718-100334.
- Zhang Z, Yu J, Li D, Zhang Z, Liu F, Zhou X, Wang T, Ling Y, Su Z. PMRD: plant microRNA database. Nucleic Acids Res. 2010;38(Database issue):D806–D813. doi:10.1093/nar/gkp818.
- Spanudakis E, Jackson S. The role of microRNAs in the control of flowering time. J Exp Bot. 2014;65(2):365–380. doi:10.1093/jxb/ert453.
- Jia Z, Zhao B, Liu S, Lu Z, Chang B, Jiang H, Cui H, He Q, Li W, Jin B, et al. Embryo transcriptome and miRNA analyses reveal the regulatory network of seed dormancy in Ginkgo biloba. Tree Physiol. 2021;41(4):571–588. doi:10.1093/treephys/tpaa023.
- Palatnik JF, Wollmann H, Schommer C, Schwab R, Boisbouvier J, Rodriguez R, Warthmann N, Allen E, Dezulian T, Huson D, et al. Sequence and expression differences underlie functional specialization of Arabidopsis microRNAs miR159 and miR319. Dev Cell. 2007;13(1):115–125. doi:10.1016/j.devcel.2019.09.016.
- Wang L, Cui J, Jin B, Zhao J, Xu H, Lu Z, Li W, Li X, Li L, Liang E, et al. Multifeature analyses of vascular cambial cells reveal longevity mechanisms in old Ginkgo biloba trees. Proc Natl Acad Sci USA. 2020;117(4):2201–2210. doi:10.1073/pnas.1916548117.
- Gupta OP, Dahuja A, Sachdev A, Kumari S, Jain PK, Vinutha T, Praveen S. Conserved miRNAs modulate the expression of potential transcription factors of isoflavonoid biosynthetic pathway in soybean seeds. Mol Biol Rep. 2019;46(4):3713–3730. doi:10.1007/s11033-019-04814-7.
- Albert NW, Thrimawithana AH, McGhie TK, Clayton WA, Deroles SC, Schwinn KE, Bowman JL, Jordan BR, Davies KM. Genetic analysis of the liverwort Marchantia polymorpha reveals that R2R3MYB activation of flavonoid production in response to abiotic stress is an ancient character in land plants. New Phytol. 2018;218(2):554–566. doi:10.1111/nph.15002.
- Kennedy DO, Wightman EL. Herbal extracts and phytochemicals: plant secondary metabolites and the enhancement of human brain function. Adv Nutr. 2011;2(1):32–50. doi:10.3945/an.110.000117.
- Zhao B, Wang L, Pang S, Jia Z, Wang L, Li W, Jin B. UV-B promotes flavonoid synthesis in Ginkgo biloba leaves. Ind Crop Prod. 2020;151:112483. doi:10.1016/j.indcrop.2020.112483.
- Xu F, Ning Y, Zhang W, Liao Y, Li L, Cheng H, Cheng S. An R2R3-MYB transcription factor as a negative regulator of the flavonoid biosynthesis pathway in Ginkgo biloba. Funct Integr Genomics. 2014;14(1):177–189. doi:10.1007/s10142-013-0352-1.
- Su X, Xia Y, Jiang W, Shen G, Pang Y. GbMYBR1 from Ginkgo biloba represses phenylpropanoid biosynthesis and trichome development in Arabidopsis. Planta. 2020;252(4):68. doi:10.1007/s00425-020-03476-1.
- Guo Y, Wang T, Fu -F-F, El-Kassaby YA, Wang G. Temporospatial flavonoids metabolism variation in leaves. Front Genet. 2020;11:589326. doi:10.3389/fgene.2020.589326.
- An J-P, Liu Y-J, Zhang X-W, Bi S-Q, Wang X-F, You C-X, Hao Y-J. Dynamic regulation of anthocyanin biosynthesis at different light intensities by the BT2-TCP46-MYB1 module in apple. J Exp Bot. 2020;71(10):3094–3109. doi:10.1093/jxb/eraa056.