ABSTRACT
In addition to its well-established role in plant development, the hormone cytokinin regulates plant responses to biotic and abiotic stresses. It was previously shown that cytokinin signaling acts negatively upon drought and osmotic stress tolerance and that gain-of-function of the cytokinin response regulator ARR1 causes osmotic stress hypersensitivity. Here we show that increased ARR1 action increases tolerance to heat shock and that this is correlated with increased accumulation of the heat shock proteins Hsp17.6 and Hsp70. These results show that the heat shock tolerance of plants can be elevated by increasing the expression of a cytokinin response activator.
Introduction
Cytokinins (CKs) have been predominantly studied as grown hormones and their roles in numerous aspects of plant development (e.g., formation and function of meristems, the control of leaf and root development, source/sink behavior of cells and organs, and the timing of leaf senescence) has been thoroughly investigated.Citation1–4 Recently, with CK biosynthesis and signaling pathways and CK-dependent growth control mechanisms defined,Citation5–8 more studies focused on understanding the role of CKs in stress responses. Although these studies implicated CKs in regulating various biotic and abiotic stress responses,Citation2–4,Citation9–14 a complete understanding of the role of CKs in the regulation of growth under stress conditions is still lacking. The emerging theme is that the role of CK in abiotic stress tolerance depends on the type of stress plants are exposed to. For example, CKs were shown to reduce drought and osmotic stress toleranceCitation15–18 but promote tolerance to temperature stress.Citation10,Citation19
An increase in temperature leads to a heat shock response, a fast inducible response that includes the heat shock factors (Hsps)-mediated transcription of genes encoding for cytoprotective heat shock proteins (Hsps).Citation20,Citation21 Hsps are molecular chaperones that refold misfolded proteins and are involved in any cellular process that requires the maintenance of proteostasis.Citation22,Citation23 Considering that CK is a growth hormone that leads to major changes in protein synthesis,Citation18,Citation24–27 it can be expected that at least some of the Hsp genes are CK-regulated to aid in the folding of the de novo synthesized CK-induced proteins. It follows that if CK content or action increases, the amount of CK-induced Hsps increases, which may affect the heat shock tolerance. Indeed, CK treatments, mutant analyses, transcriptomics, proteomics, and data mining approaches have shown that CKs are directly involved in the regulation of heat stress tolerance.Citation28–30 However, one basic and applied aspect of the CK/heat shock tolerance link has still not been investigated: it has not been shown that plants with a transgenically increased CK action have increased heat shock tolerance. Here, we analyze the heat shock tolerance of Arabidopsis transgenic lines expressing ARR1, an Arabidopsis type-B cytokinin response regulator (RR) that promotes CK responses.Citation31,Citation32 The type-B ARRs are phosphorylated during CK signal transduction, and that converts them from a latent into an active form.Citation6,Citation31–33 We show that overexpression of the wild-type and activated (phosphomimic) variant of ARR1 increases heat shock tolerance at least partly by the upregulation of Hsps, suggesting that stress tolerance can be engineered by transgenically enhancing CK signaling.
Results and discussion
It was previously shown that optimal CK sensitivity is required to maintain heat stress tolerance,Citation28 indicating a positive role for CK. To test whether increased CK action indeed increases heat shock tolerance, we analyzed the heat-shock responses of transgenic lines overexpressing the CK response activator ARR1 (ARR1 OE)Citation18 or the constitutively active phosphomimic ARR1 version (PM OE),Citation34 both of which were generated in the arr1-1 mutant background. In a first response assay, we tested the effect of heat shock on the viability of rosette leaf discs by measuring chlorophyll loss as a marker of the adverse effect of heat shock on chloroplast integrity.Citation35 Both ARR1 gain-of-function (GOF) lines (i.e., ARR1 OE and PM OE) were more tolerant to the heat shock treatment and retained more chlorophyll compared to the wild type (). There was, however, a difference between the GOF lines; the PM OE line retained more chlorophyll than the ARR1 OE line, suggesting a higher heat shock tolerance level (). The CK-resistant mutants arr1-1 and arr1-3 arr10-5 (loss-of-function lines, LOF) also responded significantly differently from the wild type and showed a hypersensitive response as evidenced by the accelerated decrease in chlorophyll content (, inset). In a second response assay, we determined the extent to which heat shock pretreatment of seeds leads to inhibition of hypocotyl elongation.Citation36 Again, both GOF lines were more tolerant to the heat shock treatment, with the PM OE line displaying a higher tolerance level than ARR1 OE, whereas LOF lines were hypersensitive to heat shock (). Therefore, the results of two physiologically and developmentally different heat shock assays show that the GOF transgenic lines have increased heat shock tolerance.
Figure 1. Heat-shock response of ARR1 gain- and loss-of-function lines. (a) Leaf discs excised from mature leaves of 30-day-old plants were floated in MES-KOH buffer (pH 6.8) for 2.5 h at 22°C (control) or 45°C (HS, heat shock) and then incubated in the same buffer at 22°C for 2 days before photography. Representative leaf discs of the Col-0 wild type (WT), arr1-1 single and double mutant, and two ARR1 gain-of-function lines are shown. Scale bar: 5 mm. (b) Chlorophyll levels in heat-shocked leaf discs. The chlorophyll content is presented as mean ± SD (n ≥ 3). *, P < .05; ****, P < .0001; ns, not significant (one-way ANOVA with Tukey’s multiple comparisons test). The insert shows the chlorophyll levels in the wild-type leaf discs compared to leaf discs of the arr1-1 and arr1-3 arr10-5 mutant. (c) Basal seed thermotolerance tests. Sterilized and stratified seeds were exposed to light for 4 h at 22°C (control) or at 45°C (heat shock) and then germinated and grown in darkness at 22°C. Etiolated seedlings were photographed after 3 days of growth, and hypocotyl lengths were measured using ImageJ. The data are presented as the percentage inhibition of hypocotyl length in response to heat shock (mean ± SD; n ≥ 20). **, P < .01; ****, P < .0001 (one-way ANOVA with Tukey’s multiple comparisons test).
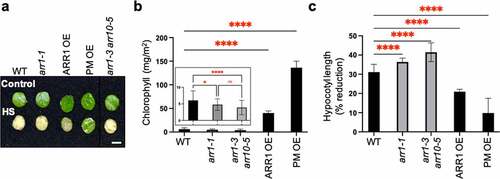
The heat shock response in plants, as in non-plant species, involves the increased expression of Hsps.Citation20,Citation21,Citation23 A previous study described a set of Hsp genes that are both heat shock- and CK-regulated.Citation28 Most of these genes belong to the Hsp20 familyCitation28 and encode small Hsps (sHsps) whose main functions are to unfold proteins, suppress aggregation, and promote protein refolding to a functional state in the ATP-independent manner.Citation37 Most notably, CK treatment upregulated the expression of members of the Hsp17.6 subfamily,Citation28 and the overexpression of one of these CK-inducible Hsp17.6 (Hsp17.6B-CII, At2g29500) promotes heat stress tolerance in transgenic plants.Citation38 That led us to test the Hsp17.6 levels in control and heat-shocked GOF and LOF lines (). The Hsp17.6 proteins were heat shock-induced in all lines, but to a different level: whereas the induction level in arr1-1 was lower than in the wild type, the induction levels were higher in both GOF lines (). In addition, whereas the Hsp17.6 proteins were undetectable in the untreated wild-type and arr1-1 plants, they were detected in the untreated GOF lines (). Considering that Hsp17.6 overexpression promotes heat shock tolerance in Arabidopsis,Citation38 increase in Hsp17.6 levels in both the untreated and heat shock-treated GOF lines is in agreement with their increased heat shock tolerance. Moreover, the PM OE line had a significantly higher level of Hsp17.6 in untreated seedlings than ARR1 OE (), which is in agreement with the higher heat shock tolerance of PM OE (). A recent study has shown that the CK transcriptomics response and the transcriptomics response to the expression of a constitutively active form of ARR1 are strikingly similar,Citation40 implying that the effects of increased ARR1 action closely resembles the effects of CK treatment. Thus, the CK-dependent regulation of Hsp genes is relevant to any phenotypical changes associated with the GOF lines. To test whether Hsp20 gene family members other than Hsp17.6 are involved in the CK response, we determined the transcript levels of all Hsp20 gene family members in plants treated with the CK trans-zeatinCitation39 (). We selected this gene expression data set for analysis because it involves treatment with a high CK concentration, which is expected to reveal a larger set of CK-regulated genes.Citation39 Indeed, it was recently shown that using a higher CK dose for treatments leads to the identification of a larger number of CK- genes.Citation40 In addition to Hsp17.6 genes, Hsp18.5, Hsp23.5, and Hsp23.6 genes were significantly upregulated by CK treatment ().
Figure 2. Cytokinin affects Hsp17.6 levels in ARR1 gain- and loss-of-function lines. (a) Representative immunoblot probed with anti-Hsp17.6 antibodies. Seven-day-old seedlings were kept for 2 h at 22°C or at 40°C. Ponceau S-stained membrane region encompassing the large subunit of RuBisCO (LSU) is shown as the loading control. (b) Quantification of the Hsp17.6 levels. Signal intensities of the control samples and the heat-shocked samples were quantified separately as they required quantification at images obtained after different exposure times. Data was normalized to the wild type and shown as mean± SD; n = 3 (**, P < .01; ****, P < .0001; one-way ANOVA with Tukey’s multiple comparisons test). (c) Relative expression levels of the Hsp20 gene family after 3-hour-long treatment with cytokinin trans-zeatin (t-z). Microarray data were extracted from publicly available series GSE5698 (AtGenExpress: Cytokinin treatment of seedlings,Citation39). Values are presented relative to the control. The significance of expression change between wild type and treatment and the intensity scale are shown on the right. Non-significant differences are not marked and significant changes are marked with an asterisks (*, P < .05; ** < 0.01; ****, P < .0001). Cytokinin-upregulated genes are circled in red.
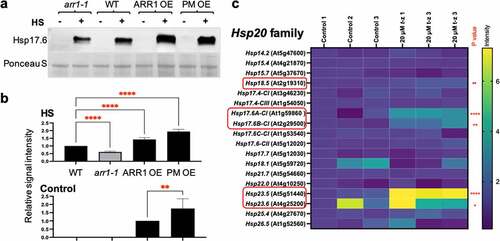
To test if ARR1 GOF also impacts heat shock tolerance by increasing the expression of other Hsps, we considered Hsp70, a heat shock protein encoded in Arabidopsis by a gene family of 17 members.Citation41 Hsp70s are proven to be tightly linked to heat shock tolerance as their overexpression increases heat stress tolerance in transgenic plants,Citation42–44 and the combined loss of function of a subset of Hsp70 genes causes heat stress hypersensitivity.Citation45 Because some,Citation46,Citation47 but not allCitation28,Citation31,Citation48–50 transcriptomic studies of CK-inducible gene sets listed an Hsp70 family member as a CK-regulated gene, we re-analyzed the effect of CK on the transcription of all Hsp70 genes. This analysis revealed that most Hsp70 genes are significantly upregulated by a three-hour-long CK treatment, while one gene (Hsp70-13) is CK repressed, and three Hsp genes are not affected (). This suggested that the upregulation of Hsp70 protein levels is an important part of the CK response.
Figure 3. Cytokinin affects Hsp70 levels. (a) Expression levels of the Hsp70 gene family after 3-hour-long treatment of 21-day-old plants with cytokinin trans-zeatin (t-z). Values are presented relative to the control. Non-significant differences (ns) are marked. *, P < .05; ****, P < .0001. The AGI code of Hsp70-11 (BIP1) is At5g28540, Hsp70-12 (BIP2) is At5g42020, Hsp70-14 is At1g79930, and Hsp70-15 is At1g79920. (b) Representative immunoblot probed with anti-Hsp70 antibodies. The experimental conditions as the same as in . Ponceau S-stained membrane region encompassing the large subunit of RuBisCO (LSU) is shown as the loading control. (c) Quantification of the Hsp70 levels. Data were normalized to the wild type and shown as mean± SD; n = 3 (* < 0.05; two-way ANOVA with Šídák’s multiple comparisons test).
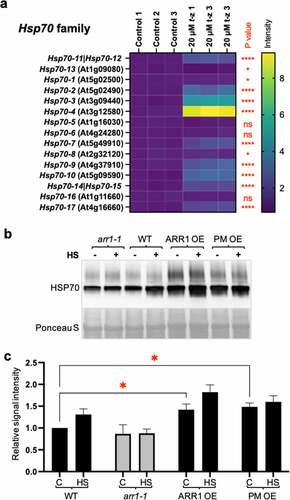
To test whether we can detect changes in Hsp70s levels in GOF and LOF lines, we used anti-Hsp70 antisera raised against a synthetic peptide conserved in all higher plant Hsp70 proteins, which allowed us to recognize the total change in Hsp70 levels. Hsp70 levels were increased in both untreated GOFs, whereas the decrease in arr1-1 was trending but not significantly lower compared to the wild type (). Hsp70s were induced by heat shock in the wild type, but no induction was apparent in the arr1-1 mutant, suggesting that heat shock induction of Hsp70s requires a functional CK response pathway (). The elevated Hsp70 protein level in GOF lines was not further increased by a two-hour heat shock, indicating that the increased CK action raised the Hsp70 level to a maximum (). Contrary to the different upregulation levels of Hsp17.6 in the GOF lines, the Hsp70 increase was nearly identical, suggesting that the increased CK action in both of these lines was sufficient to raise the Hsp70 level to this maximum. We concluded that the heat shock tolerance conferred by increased ARR1 action involves the increased expression of genes belonging to at least two Hsp gene families, namely Hsp20 and Hsp70.
Summary
We have shown that the gain of function of the CK response activator ARR1 enhances heat shock tolerance and that this is – at least in part – mediated by the increased accumulation of Hsp17.6 and Hsp70. Since both the CK signaling and heat shock tolerance mechanisms are conserved throughout higher plants,Citation5,Citation20 we suggest that increasing CK action is a promising approach for improving the heat shock tolerance of agriculturally important plant species.
Materials and methods
Plant materials and growth conditions
The lines used were Arabidopsis (Arabidopsis thaliana L. Heynh) Columbia 0 (Col-0) as the wild-type, and the Col-0 mutants arr1-1Citation51 (a gift from Atsuhiro Oka) and arr1-3 arr10-5Citation31 (obtained from the ABRC Seed Stock Center). The wild-type ARR1 overexpression line (ARR1 OE) and phosphomimic ARR1 expression line (PM OE) in the arr1-1 mutant background were previously described.Citation34,Citation52 For all experiments, seeds were surface sterilized, stratified for one day, and plated on half-strength Murashige and Skoog medium containing 1% sucrose and 0.8% PhytoAgar (MS/2, pH 5.7). Plants were grown in a growth chamber at 22°C under continuous light (80 mmol m−2 s−1).
Heat shock treatments and immunoblotting
Seedlings were grown for seven days on MS/2 media in the growth chamber set at 22°C. Half of the samples were then transferred to an illuminated incubator set at 40°C and heat-shocked for two hours. For protein extraction, seedlings were weighed, three volumes of 2x Laemmli sample buffer were added, and tissue was disrupted using zirconium beads in a bead beater. After pelleting the debris, proteins were separated on 4–20% SDS-PAGE gels (Bio-Rad) and transferred to nitrocellulose membranes. Membranes were blocked using 10% fat-free milk and washed with PBS containing 0.2% Tween‐20. Antibodies used were anti-Hsp17.6 (1:5,000 dilution; Agrisera Product no AS07 254), anti-Hsp70 (1:5,000; Agrisera Product no AS08 371), and horseradish peroxidase-conjugated goat anti-rabbit antisera (1:1000; Santa Cruz, CA, USA). Immunoblots were developed using SuperSignal West Femto substrate (Thermo-Pierce, Rockford, IL, USA) using a ChemiDoc XRS molecular imager and quantified using Quantity One® software (Bio-Rad).
Heat shock response assays
Basal seed thermotolerance was tested using the protocol described by Hong and Vierling.Citation36 In brief, the seeds were sterilized, sown on MS/2 media, stratified, and either exposed to light for 4 hours at 45°C (heat-shocked samples) or exposed to light at 22°C (control samples). The plates were then wrapped in aluminum foil and transferred to a growth chamber set at 22°C, where they were kept for three days. The etiolated seedlings were photographed, and their hypocotyl lengths were measured by using ImageJ software.
In the leaf disc assay, the leaf discs were punched out from mature leaves of 30-day-old soil-grown plants transferred to 12-well cell culture plates with 10 mM MES-KOH buffer, pH 6.8 (2 ml per well). Control samples were kept at 22°C, and the test samples were kept at 45°C for 2.5 hours. Heat-shocked samples were then returned to 22°C, and leaf discs were photographed after two days. The chlorophyll content was measured using a CCM 300 chlorophyll content meter (Opti-Sciences).
Statistical and transcriptomic analyses
Descriptive statistics, plotting, and hypothesis testing were done by using Prism 9.3 (GraphPad Software Inc., La Jolla, CA, USA). All data are presented as mean ± SD. The number of replicates and statistical tests used for the analyses are indicated in Figure Legends. The publicly available series GSE5698 (AtGenExpress: Cytokinin treatment of seedlingsCitation39) was obtained from the Gene Expression Omnibus (GEO) database at the National Center for Biotechnology Information (NCBI). GEO2R was used to obtain sample values used for heat map generation. The sample values were normalized to wild-type control, analyzed, and displayed using Prism 9.3.
Disclosure statement
No potential conflict of interest was reported by the author(s).
Additional information
Funding
References
- Kieber JJ, Schaller GE. Cytokinin signaling in plant development. Development. 2018:145. doi:10.1242/dev.149344.
- Li SM, Zheng HX, Zhang XS, Sui N. Cytokinins as central regulators during plant growth and stress response. Plant Cell Rep. 2021;40(2):271–6. doi:10.1007/s00299-020-02612-1.
- McIntyre KE, Bush DR, Argueso CT. Cytokinin regulation of source-sink relationships in plant-pathogen interactions. Front Plant Sci. 2021;12:677585. doi:10.3389/fpls.2021.677585.
- Kieber JJ, Schaller GE. Cytokinins. Arabidopsis Book. 2014;12:e0168. doi:10.1199/tab.0168.
- Gruhn N, Heyl A. Updates on the model and the evolution of cytokinin signaling. Curr Opin Plant Biol. 2013;16(5):569–574. doi:10.1016/j.pbi.2013.09.001.
- Hwang I, Sheen J, Muller B. Cytokinin signaling networks. Annu Rev Plant Biol. 2012;63(1):353–380. doi:10.1146/annurev-arplant-042811-105503.
- Kang J, Lee Y, Sakakibara H, Martinoia E. Cytokinin transporters: GO and STOP in signaling. Trends Plant Sci. 2017;22(6):455–461. doi:10.1016/j.tplants.2017.03.003.
- Sakakibara H. Cytokinins: activity, biosynthesis, and translocation. Annu Rev Plant Biol. 2006;57(1):431–449. doi:10.1146/annurev.arplant.57.032905.105231.
- Gujjar RS, Supaibulwatana K. The mode of cytokinin functions assisting plant adaptations to osmotic stresses. Plants (Basel). 2019;8(12). doi:10.3390/plants8120542.
- Zwack PJ, Rashotte AM. Interactions between cytokinin signalling and abiotic stress responses. J Exp Bot. 2015;66(16):4863–4871. doi:10.1093/jxb/erv172.
- Kurepa J, Smalle JA. Auxin/cytokinin antagonistic control of the shoot/root growth ratio and its relevance for adaptation to drought and nutrient deficiency stresses. Int J Mol Sci. 2022;23(4):1933. doi:10.3390/ijms23041933.
- Verma V, Ravindran P, Kumar PP. Plant hormone-mediated regulation of stress responses. BMC Plant Biol. 2016;16(1). doi:10.1186/s12870-016-0771-y.
- Bielach A, Hrtyan M, Tognetti V. Plants under stress: involvement of auxin and cytokinin. Int J Mol Sci. 2017;18(7):1427. doi:10.3390/ijms18071427.
- Argueso CT, Ferreira FJ, Kieber JJ. Environmental perception avenues: the interaction of cytokinin and environmental response pathways. Plant Cell Environ. 2009;32(9):1147–1160. doi:10.1111/j.1365-3040.2009.01940.x.
- Nishiyama R, Watanabe Y, Leyva-Gonzalez MA, Ha CV, Fujita Y, Tanaka M, Seki M, Yamaguchi-Shinozaki K, Shinozaki K, Herrera-Estrella L, et al. Arabidopsis AHP2, AHP3, and AHP5 histidine phosphotransfer proteins function as redundant negative regulators of drought stress response. Proc Natl Acad Sci USA. 2013;110(12):4840–4845. doi:10.1073/pnas.1302265110.
- Nishiyama R, Watanabe Y, Fujita Y, Le DT, Kojima M, Werner T, Vankova R, Yamaguchi-Shinozaki K, Shinozaki K, Kakimoto T, et al. Analysis of cytokinin mutants and regulation of cytokinin metabolic genes reveals important regulatory roles of cytokinins in drought, salt and abscisic acid responses, and abscisic acid biosynthesis. Plant Cell. 2011;23(6):2169–2183. doi:10.1105/tpc.111.087395.
- Tran LS, Urao T, Qin F, Maruyama K, Kakimoto T, Shinozaki K, Yamaguchi-Shinozaki K. Functional analysis of AHK1/ATHK1 and cytokinin receptor histidine kinases in response to abscisic acid, drought, and salt stress in Arabidopsis. Proc Natl Acad Sci USA. 2007;104(51):20623–20628. doi:10.1073/pnas.0706547105.
- Karunadasa SS, Kurepa J, Shull TE, Smalle JA. Cytokinin-induced protein synthesis suppresses growth and osmotic stress tolerance. New Phytol. 2020;227(1):50–64. doi:10.1111/nph.16519.
- Zwack PJ, Compton MA, Adams CI, Rashotte AM. Cytokinin response factor 4 (CRF4) is induced by cold and involved in freezing tolerance. Plant Cell Rep. 2016;35(3):573–584. doi:10.1007/s00299-015-1904-8.
- Haq SU, Khan A, Ali M, Khattak AM, Gai WX, Zhang HX, Wei AM, Gong ZH. Heat shock proteins: dynamic biomolecules to counter plant biotic and abiotic stresses. Int J Mol Sci. 2019;20:5321. doi:10.3390/ijms20215321.
- Richter K, Haslbeck M, Buchner J. The heat shock response: life on the verge of death. Mol Cell. 2010;40(2):253–266. doi:10.1016/j.molcel.2010.10.006.
- Hartl FU, Bracher A, Hayer-Hartl M. Molecular chaperones in protein folding and proteostasis. Nature. 2011;475(7356):324–332. doi:10.1038/nature10317.
- Baniwal SK, Bharti K, Chan KY, Fauth M, Ganguli A, Kotak S, Mishra SK, Nover L, Port M, Scharf K-D, et al. Heat stress response in plants: a complex game with chaperones and more than twenty heat stress transcription factors. J Biosci. 2004;29(4):471–487. doi:10.1007/bf02712120.
- Klämbt D. Cytokinin effects on protein synthesis of in vitro systems of higher plants. Plant Cell Physiol. 1976;17(1):73–76. doi:10.1093/oxfordjournals.pcp.a075267.
- Szweykowska A, Gwóźdź E E, Spychała M. 1981. The cytokinin control of protein synthesis in plants. Metabolism and Molecular Activities of Cytokinins. Berlin (Heidelberg): Springer, 212–217.
- Muren RC, Fosket DE. Cytokinin-mediated translational control of protein synthesis in cultured cells of glycine max. J Exp Bot. 1977;28(4):775–784. doi:10.1093/jxb/28.4.775.
- Tepfer DA, Fosket DE. Hormone-mediated translational control of protein synthesis in cultured cells of Glycine max. Dev Biol. 1978;62(2):486–497. doi:10.1016/0012-1606(78)90230-0.
- Černý M, Jedelský P, Novák J, Schlosser A, Brzobohatý B. Cytokinin modulates proteomic, transcriptomic and growth responses to temperature shocks in Arabidopsis. Plant Cell Environ. 2014;37:1641–1655. doi:10.1111/pce.12270.
- Cortleven A, Leuendorf JE, Frank M, Pezzetta D, Bolt S, Schmülling T. Cytokinin action in response to abiotic and biotic stresses in plants. Plant Cell Environ. 2019;42(3):998–1018. doi:10.1111/pce.13494.
- Pavlů J, Novák J, Koukalová V, Luklová M, Brzobohatý B, Černý M. Cytokinin at the crossroads of abiotic stress signalling pathways. Int J Mol Sci. 2018;19(8):2450. doi:10.3390/ijms19082450.
- Argyros RD, Mathews DE, Chiang Y-H, Palmer CM, Thibault DM, Etheridge N, Argyros DA, Mason MG, Kieber JJ, Schaller GE, et al. Type B response regulators of Arabidopsis play key roles in cytokinin signaling and plant development. Plant Cell. 2008;20(8):2102–2116. doi:10.1105/tpc.108.059584.
- Ishida K, Yamashino T, Yokoyama A, Mizuno T. Three type-B response regulators, ARR1, ARR10 and ARR12, play essential but redundant roles in cytokinin signal transduction throughout the life cycle of Arabidopsis thaliana. Plant Cell Physiol. 2008;49(1):47–57. doi:10.1093/pcp/pcm165.
- Kieber JJ, Schaller GE. The perception of cytokinin: a story 50 years in the making. Plant Physiol. 2010;154(2):487–492. doi:10.1104/pp.110.161596.
- Kurepa J, Li Y, Perry SE, Smalle JA. Ectopic expression of the phosphomimic mutant version of Arabidopsis response regulator 1 promotes a constitutive cytokinin response phenotype. BMC Plant Biol. 2014;14(1):28. doi:10.1186/1471-2229-14-28.
- Hu S, Ding Y, Zhu C. Sensitivity and responses of chloroplasts to heat stress in plants. Front Plant Sci. 2020;11:375. doi:10.3389/fpls.2020.00375.
- Hong SW, Vierling E. Mutants of Arabidopsis thaliana defective in the acquisition of tolerance to high temperature stress. Proc Natl Acad Sci USA. 2000;97(8):4392–4397. doi:10.1073/pnas.97.8.4392.
- Haslbeck M, Vierling E. A first line of stress defense: small heat shock proteins and their function in protein homeostasis. J Mol Biol. 2015;427(7):1537–1548. doi:10.1016/j.jmb.2015.02.002.
- Tawab F, Munir I, Ullah F, Iqbal A. Over-expressed HSP17.6b, encoding HSP20-like chaperones superfamily protein, confers heat stress tolerance in Arabidopsis thaliana. Pak J Bot. 2019;51(3):855–864. doi:10.30848/PJB2019-3(36).
- Goda H, Sasaki E, Akiyama K, Maruyama-Nakashita A, Nakabayashi K, Li W, Ogawa M, Yamauchi Y, Preston J, Aoki K, et al. The AtGenExpress hormone and chemical treatment data set: experimental design, data evaluation, model data analysis and data access. Plant J. 2008;55(3):526–542. doi:10.1111/j.0960-7412.2008.03510.x.
- Xie M, Chen H, Huang L, O’Neil RC, Shokhirev MN, Ecker JR. A B-ARR-mediated cytokinin transcriptional network directs hormone cross-regulation and shoot development. Nat Commun. 2018:9. doi:10.1038/s41467-018-03921-6.
- Sung DY, Vierling E, Guy CL. Comprehensive expression profile analysis of the Arabidopsis Hsp70 gene family. Plant Physiol. 2001;126(2):789–800. doi:10.1104/pp.126.2.789.
- Cazalé AC, Clemént M, Chiarenza S, Roncato MA, Pochon N, Creff A, Marin E, Leonhardt N, Noël LD. Altered expression of cytosolic/nuclear HSC70-1 molecular chaperone affects development and abiotic stress tolerance in Arabidopsis thaliana. J Exp Bot. 2009;60(9):2653–2664. doi:10.1093/jxb/erp109.
- Sung DY, Guy CL. Physiological and molecular assessment of altered expression of Hsc70-1 in Arabidopsis. Evidence for pleiotropic consequences. Plant Physiol. 2003;132(2):979–987. doi:10.1104/pp.102.019398.
- Montero-Barrientos M, Hermosa R, Cardoza RE, Gutierréz S, Nicolàs C, Monte E. Transgenic expression of the Trichoderma harzianum hsp70 gene increases Arabidopsis resistance to heat and other abiotic stresses. J Plant Physiol. 2010;167(8):659–665. doi:10.1016/j.jplph.2009.11.012.
- Leng L, Liang Q, Jiang J, Zhang C, Hao Y, Wang X, Su W. A subclass of HSP70s regulate development and abiotic stress responses in Arabidopsis thaliana. J Plant Res. 2017;130(2):349–363. doi:10.1007/s10265-016-0900-6.
- Brenner WG, Ramireddy E, Heyl A, Schmülling T. Gene regulation by cytokinin in Arabidopsis. Front Plant Sci. 2012;3:8. doi:10.3389/fpls.2012.00008.
- Kiba T, Naitou T, Koizumi N, Yamashino T, Sakakibara H, Mizuno T. Combinatorial microarray analysis revealing Arabidopsis genes implicated in cytokinin responses through the His->Asp Phosphorelay circuitry. Plant Cell Physiol. 2005;46(2):339–355. doi:10.1093/pcp/pci033.
- Bhargava A, Clabaugh I, To JP, Maxwell BB, Chiang YH, Schaller GE, Loraine A, Kieber JJ. Identification of cytokinin-responsive genes using microarray meta-analysis and RNA-Seq in Arabidopsis. Plant Physiol. 2013;162(1):272–294. doi:10.1104/pp.113.217026.
- Hoth S, Ikeda Y, Morgante M, Wang X, Zuo J, Hanafey MK, Gaasterland T, Tingey SV, Chua NH. Monitoring genome-wide changes in gene expression in response to endogenous cytokinin reveals targets in Arabidopsis thaliana. FEBS Lett. 2003;554(3):373–380. doi:10.1016/s0014-5793(03)01194-3.
- Rashotte AM, Carson SD, To JP, Kieber JJ. Expression profiling of cytokinin action in Arabidopsis. Plant Physiol. 2003;132:1998–2011. doi:10.1104/pp.103.021436.
- Sakai H, Honma T, Aoyama T, Sato S, Kato T, Tabata S, Oka A. ARR1, a transcription factor for genes immediately responsive to cytokinins. Science. 2001;294(5546):1519–1521. doi:10.1126/science.1065201.
- Li Y, Kurepa J, Smalle J. AXR1 promotes the Arabidopsis cytokinin response by facilitating ARR5 proteolysis. Plant J. 2013;74(1):13–24. doi:10.1111/tpj.12098.