ABSTRACT
Climbing plants rely on suitable support to provide the light conditions they require in the canopy. Negative phototropism is a directional search behavior proposed to detect a support-tree, which indicates growth or movement away from light, based on light attenuation. In a Chilean temperate rainforest, we addressed whether the massive woody climber Hydrangea serratifolia (H. et A.) F. Phil (Hydrangeaceae) presents a support-tree location pattern influenced by light availability. We analyzed direction and light received in two groups of juvenile shoots: searching shoots (SS), with plagiotropic (creeping) growth vs. ascending shoots (AS), with orthotropic growth. We found that, in accordance with light attenuation, SS and AS used directional orientation to search and then ascend host trees. The light available to H. serratifolia searching shoots was less than that of the general forest understory; the directional orientation in both groups showed a significant deviation from a random distribution, with no circular statistical difference between them. Circular-linear regression indicated a relationship between directional orientations and light availability. Negative phototropism encodes the light environment’s heterogeneous spatial and temporal information, guiding the shoot apex to the most shaded part of the support-tree base, the climbing start point.
Introduction
Plants are not as passive as they appear, experiencing motion through timescales and distances not always apparent to human observers.1–Citation3 They actively forage for limited abiotic and biotic resources distributed in unequal quantity, quality, space, and time.Citation4–6 Foraging is an iterative processCitation7 based on searching (detecting resources), encountering (growth toward a potential resource) and decision-making (assessing whether it is worth the effort to obtain a resource).Citation8–13 This process is crucial and effective in young plants because it is directly related to the present and future competitive environment that they will experience through their ontogeny.Citation14–19
In this context, plant tropisms, active responses involving acquiring and processing information from directional physical stimuli or environmental cues–coupled with plant modular structure–guide individuals toward favorable conditions.Citation10,Citation20–27 Light performs a dual role in plants as the fundamental resource for photosynthesis and provides external environmental information (cues) as directional stimuli.Citation25,Citation28–32 When light availability is heterogeneous, plant shoots change their directional growth pattern (reorient) toward the optimal light intensity; they then assess the foraging efficiency of obtaining the light and resources associated with light cues.Citation11,Citation32–43
Climbing plants rely on their attachment to suitable external supports through their vegetative structures and directional searching movements.Citation33,Citation35,Citation38–40,Citation44–50 This group of plants also present a life-history strategy of extensive clonal growth, a principal driver that determines their local spatial distribution patterns, regeneration, colonization success, and contributes to their abundance and resources foraging.Citation51–55 Clonal plants can employ as response to light competition in three categories, considered as vertical (confrontational) growth, shade tolerance or lateral avoidance.Citation13 Individuals successfully attached to a support-tree improve their light intake, are more abundant and present higher biomass, physiological yield, reproductive output, and lower herbivory than those unattached.Citation12,Citation47,Citation56–61
In woody climbers with adventitious roots, the plagiotropic (creeping) shoots and seedlings exposed to bright light, or even to low-light intensity, grew toward dark sites and moved away from light, exhibiting negative phototropism.Citation33,Citation35,Citation38–40,Citation42 In the chiaroscuro of the forest floor, potential support-trees have been found in the darkest sectors,Citation33 and under shady conditions, the climbing habit has also been found to be enhanced.Citation51,Citation56,Citation58,Citation59,Citation62 Nevertheless, how the creeping shoots of woody climbers reach and ascend support-trees, thanks to an efficient search behavior, has received little research attention,Citation33,Citation35,Citation42,Citation51,Citation63,Citation64 compared to studies regarding mechanisms that explain property components such as density, biomass, diversity or distribution of climbing plants.Citation53,Citation60,Citation65–72
In a southern Chilean temperate rainforest, the density of climbers was 66 individuals per 0.1 ha, with the native and woody root climber (liana) Hydrangea serratifolia (H. et A.) F. Phil (Hydrangeaceae) being the dominant species, with more than 52 individuals.Citation65,Citation69 H. serratifolia is the only climbing plant that extends to the mature canopy, reaching up to 30 m long, covering 54% of trees in this forest, regardless of support-tree bark type, light requirements, or diameter.Citation73,Citation74 This species is shade-tolerant, with intermediate values of area-based photosynthetic capacity (Amax) and dark respiration (Rd) rates, efficiently intercepts light and reduces gas-exchange rates to cope with the low-light availability found in the mature forest understory.Citation75 Furthermore, H. serratifolia has registered the highest liana population density among 97 worldwide tropical and temperate mature forests sampled with the same methodology.Citation65 H. serratifolia presents two phases to attain resources: First, juvenile shoots – by plagiotropic growth – creep along the forest floor in search of a support-tree. They are singular, slender, and reddish, with ventral white adventitious roots ready to attach to a hostCitation76 and a conspicuous apical greenish bud. The successful juvenile shoots, attached to a tree, climb it by orthotropic growth to access the increased light available in the canopy. At this point, they switch their morphogenesis to the second and adult phase, becoming woody, brownish, and showing reproductive structures (pers. obs). The support-tree location pattern of H. serratifolia, based on the combination of the juvenile shoot response and spatial variation in forest environmental light, is essential to understand and subsequently link the multiple causes of the abundance, distribution, and dominance of this species in Chilean temperate rainforests.
This study addresses the question: Does the massive woody climber H. serratifolia present a support-tree location pattern influenced by light cues in a southern Chilean temperate rainforest? We compared the light received and the direction in two groups of juvenile shoots: Searching shoots (SS), with plagiotropic (creeping) growth versus ascending shoots (AS), with orthotropic growth. We hypothesized that, if low-light availability represents a directional search cue associated with a support-tree location pattern, the light perceived and orientation from the SS group would be similar to those from group AS, exhibiting negative phototropism. The alternative working hypothesis would discard that low-light availability is not a directional search cue nor related to a support-tree location pattern, evidenced by random values of light perceived and orientation in both groups (SS and AS).
Materials and methods
This study was carried out in the mature forest of Parque Nacional Puyehue (40°39’ S, 72°11’ W, 350 m.a.s.l.), a temperate rainforest at the western foothills of the Andes, in central-southern Chile. Parque Nacional Puyehue exhibits a maritime temperate climate, an annual precipitation of 3500 mm, and minimum and maximum annual average temperatures of 5.4°C and 13.8°C, respectively.Citation77–79We set up eight randomly sampling plots of 25 m × 5 m (0.1 ha total) in the forest understory–avoiding gaps, edges or different slopes–, all of them had the same northern facing exposure to prevent possible biases related to different sunlight time exposition.
Inside each plot, we registered the orientation degrees (directionality) of juvenile shoots with plagiotropic (SS = searching shoots group) and orthotropic (AS = ascending shoots group) growth, using a compass (Suunto® A-10, Finland) in relation to the direction of true magnetic north (directional vectors) and the degrees of the sunset across sampling days. For SS, we selected all those juvenile creeping shoots when they pointed to the closest potential support-tree <2.5 m and not to dead ends (e.g., shadow of rocks, dens, or holes in the soil), to avoid possible confounding effects by distanceCitation80 or a possible automated growth toward darkness. We measured the directional orientation in each juvenile creeping shoot (SS) over its active apical bud. For AS, we defined as orientation reference (direction) the first successful shoot contact point (i.e., starts to climb toward the canopy) at the base of the support-tree trunk, to determine if the path taken on the ground leads it to find the support-tree, not by chance. We chose all those juvenile shoots with orthotropic growth (AS) actively ascending with their adventitious roots attached to the first support-tree 50 cm above the ground (considering this as a measure of successful attachment). SS and AS were measured without differentiation in ramets and genets because it was difficult to identify their origin, although their dynamics and effects in the forest were comparable.Citation55,Citation81–84 We did not register tree identity, diameter, and density in potential vs. climbed support-trees because tree stem density has not proven to influence the liana-host selection.Citation81 H. serratifolia has also shown no support-tree type or diameter preferences.Citation73,Citation74 Furthermore, we chose SS and AS because these allow us to compare if there are directional orientation and concordance between the present (SS) and past (AS) information acquiring shoots.Citation85,Citation86 SS and AS were expected to access the trunk face that receives less sunlight exposition (southwest, SW), opposite from the trunk facing the sunrise (northeast, NE).
In sampled plots, the light environment on the forest floor and the light radiation received by SS, previously used to register the directional orientation, were quantified using hemispherical photographs. We took a picture 0.3 m above the ground at the center of each plot to characterize the forest light environment. To depict the light availability received by SS, we photographed each creeping shoot just above the active apical bud, as close to the ground as possible (8–10 cm). The photographs were taken with a digital camera (CoolPix 995, Nikon®, Tokyo, Japan), mounted and horizontally leveled on a tripod oriented north to south (by a Suunto® A-10 compass, Finland) with a fisheye lens with a 180° field of view (FCE8, Nikon®). All hemispherical photographs were taken under homogeneous cloudy conditions, near dawn or dusk, to avoid possible bias due to diary natural light fluctuations. The quality of the photos was set at a fine resolution of 1:4 compression in a JPEG format. We calculated, using HemiView® software canopy analysis version 2.1 (2000, Delta-T Devices Ltd, UK) and the geographic location, in each hemispherical photo the indirect site factor (ISF), diffuse proportion of solar radiation expected to reach the point where photographs were takenCitation87 and global site factor (GSF), considered as the total amount of diffuse and direct light that penetrates the canopy, reaching a specific point, relative to the amount that would be received with no canopy interception,Citation88 analogous to the percentage of photosynthetically active radiation (PAR).Citation89
The percentages of diffuse light incidence (ISF %), registered in the forest light environment (inside plots) and above each creeping shoot (SS), were used to compare frequency histograms and density lines to determine whether there were any differences among the values obtained. Likewise, to quantify the light incidence received in the AS, we registered photosynthetic active radiation (PAR; µmol·m−2·s−1) on the trunk base where each juvenile shoot with orthotropic growth was attached to the support-tree and on the opposite free trunk face (180°) using a LiCor 250A light meter (LiCor, Lincoln, NE, USA). PAR readings on AS were carried out under cloudy conditions at noon, avoiding possible bias produced by the diary natural light fluctuations. At the same time, a full sun PAR measurement was taken outside the forest to convert the light availability of each ascending shoot into the maximum PAR percentage for future comparisons.
All directional data were analyzed with circular statistics,Citation90–96 previously used in plant distribution,Citation97,Citation98 and Dynophyceae movement.Citation99 We assessed whether SS and AS presented: (1) an oriented distribution, (2) differences in their orientation distributions and (3) a relationship between the perceived light availability and the directional search orientation toward the structural support. In SS and AS separately, we carried out the statistical hypotheses test sequence – Kuiper, Watson, and Rayleigh –,Citation100 to examine the null hypothesis that SS and AS of H. serratifolia are distributed without showing a preferred orientation (uniform distribution, random orientation). These tests can evaluate if the directional data present evidence for uniform, von Mises and unimodal distribution, respectively.Citation100 Through the Rayleigh z test, due its better performance in comparison with other commonly used tests,Citation101 we assessed if the sample data of SS and AS showed a single modal direction,Citation90,Citation91,Citation100,Citation101 z value represents how large the mean length vector must be to indicate a nonrandom population, with values of more than 0.5 the hypotheses of randomness can be rejected.Citation102 The mean resultant length vector rho of circular data of SS and AS measures the concentration of unimodal circular data [0, 1]. rho values close to 1 denote data strongly clustered around the mean direction (long vector length, reaching the circle border), while values close to 0 indicate data spread more evenly around the circle (short vector length, far from circle border).Citation93,Citation103,Citation104 After that, to analyze if the orientation distributions differed significantly between SS and AS, we applied the Watson's two-sample test of homogeneity to compare their directional means.Citation92,Citation104
Linear–circular regressionsCitation92,Citation105,Citation106 were also performed separately for both types of juvenile shoots (SS and AS) to test a possible relationship between shoot direction and the light radiation received. The circular response variable is the directional orientation measured in degrees, and the linear explanatory variable is light availability, quantified by the GSF (%) for SS and PAR (%) for AS (as a meaningful predictor), respectively. A general procedure is not yet available to quantify and measure circular–linear correlations with well-defined properties.Citation107 Currently, measures of the hippocampal phase precession weakly depend on prior knowledge of the dataCitation107 and associations between two circular variables or correlations (with transformations) for linear–linear data analyses.Citation91,Citation92,Citation105,Citation106,Citation108 Finally, in AS to compare whether the values of light incidence received (PAR %) differed between the support-tree trunk face where the juvenile H. serratifolia shoots were found ascending and the opposite trunk face, we used a paired t-test to determine the difference between the two means.Citation108
All analyses were conducted in the R software environment 4.2.2,Citation109 with a 5% significance level using the “circular” (version 0.4–93),Citation104 “CircStats” (version 0.2–6)Citation110 and “NPCirc”Citation111 packages; including the functions CircularBoxplot,Citation112 lm.circular (version 0.4–93)Citation104 and kern.reg.lin.circ,Citation111 implementing Local-Linear estimators to obtain graphical trend lines.Citation113
Results
H. serratifolia searching and ascending shoots presented a significant deviation from a uniform distribution (, ). A total of 124 individual of H. serratifolia juvenile shoots were found in 0.1 ha. We measured 70 juvenile shoots with plagiotropic (creeping) growth (SS = searching shoots), and 54 ascending on support-trees with orthotropic growth (AS = ascending shoots). The orientation distribution of SS and AS, observed by density lines and rose diagrams, covers the angle range of [135°; 315°] (). The frequency distribution shows that SS and AS share significant frequencies – in orientation degrees – to creep and ascend on the support-tree, among [160°;180] and [225°;250°] (). In detail, SS on the forest floor exhibited an average directional orientation of 226.2° ± 0.92° (mean ± SD), toward southwest (SW), and an interquartile range of [180°;260°] (, ). AS were oriented, on average, at 205.8° ± 0.90° (mean ± SD); with a tendency to climb on south-southwest (SSW) side of the host tree trunk and an interquartile range of [160°;235°] (, ). Both groups of juvenile shoots tended to creep – or climb – on the support-tree trunk face that receives less sun exposition, considering the frequency in sunset degrees measured along the sampling days () and the sun rises in the northeast, NE.
Figure 1. Rose diagrams, density lines and circular boxplots of H. serratifolia juvenile searcher (a, b) and ascending (c, d) shoots. Black arrows represent rho (pointing to the mean direction) and blue line the circular median value. Light gray petal represents the frequency, in sunset degrees, along the sampling days. Dark gray petals represents the frequency distribution of SS (a) and AS (c), respectively. Black dots represent each individual orientation measurement for SS and AS over the circle (a–c).
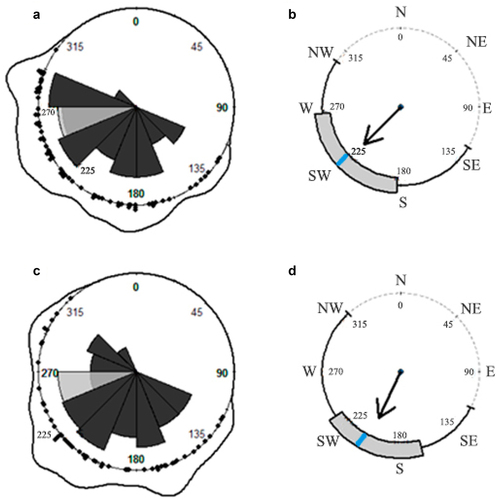
Table 1. Circular summary statistics for H. serratifolia’s juvenile searcher (SS) and ascending shoots (AS).
We found using the circular hypotheses test sequence that SS and AS directional data were not uniform distributed (SS Kuiper’s test, N = 70, V = 4.414, p-value < 0.01; AS Kuiper’s test, N = 54, V = 3.475, p-value< 0.01), fit the von Mises distribution (SS Watson’s test; N = 70, U2 = 1.632, p-value < 0.01; AS Watson’s test, N = 54, U2 = 1.219, p-value < 0.01), and displayed single directional orientation tendencies (SS Rayleigh’s uniformity test, N = 70, z = 0.6644, p-value = 3.79;−14 AS Rayleigh’s uniformity test, N = 54, z = 0.6535, p-value = 9.67 e−11). Rayleigh’s uniformity test z values for SS (z = 0.6644) and AS (z = 0.6535) with values of more than 0.5 in the mean length vector indicate that they are nonrandom. Furthermore, no statistically significant differences were found between the mean directions µ (orientation) of the two groups of juvenile H. serratifolia samples (Watson’s two-sample test, N1 = 70 SS sample size, N2 = 54 AS sample size, critical value = 0.268, U2 = 0.1454).
The linear–circular regressions suggested a possible relationship between directional orientation (circular response variable) and light availability (independent linear predictor) for H. serratifolia SS (GSF %, N = 70, μˆ = −2.04 radians, κˆ = 1.82 and γˆ1 = – 0.008, S.E. = 0.005, p-value <0.01; Appendix 1) and AS (PAR %, N = 50, μˆ = −2.54 radians, κˆ = 1.83 and γˆ1 = – 0.075, S.E. = 0.039, p-value = 0.028; Appendix 1). The results propose that SS ([7;13] GSF%) and AS ([0.5; 1.5] PAR%) oriented toward the mean direction presented lower light availability values than those oriented away from that direction (Appendix 1). Thereby, the orientation angle of shoots (considering the concentration as well as the mean direction of the distribution of the circular response variable) may be influenced by low values of light availability (linear predictor). Light attenuation influences the directional support-tree pattern of H. serratifolia shoots. Furthermore, we found that the diffuse light (ISF %) received by H. serratifolia searching shoots (SS) presented a range of lower values ([0; 0.15] ISF %) than those obtained on the forest floor, evidenced by the differences in frequency distributions and density lines between this group and the general forest light environment (). Finally, we also found differences between the percentage values of photosynthetic active radiation (PAR %) received by the support-tree trunk face (mean = 1.31) where H. serratifolia juvenile shoots were ascending and the opposite (mean = 2.27) free trunk face (paired t-test, df = 36.39, t = −4.125, p-value <0.001).
Discussion
H. serratifolia, a massive and successful woody climber (liana) in Parque Nacional Puyehue, presented a directional pattern of support-tree location based on light attenuation and shoot orientation, with goal-directed biological motion. Searching shoots (SS) were found in reasonably dark sites on the forest floor () and ascending shoots (AS) in very dark sites on support-tree trunks that receive little light exposition. Both groups of juvenile H. serratifolia shoots were oriented toward the southwest, with no circular statistical difference among them (, ) and a circular–linear relationship between the direction (orientation angles) and light availability perceived (Appendix 1). Support-tree location, including perception and action, requires precisionCitation33–35,Citation46 and a speed-accuracy trade-off, where rapid and impulsive movements are prone to be more inaccurate than slower and more cautious actions.Citation114–116
Climbers are ecological perceivers of possible interactions and key invariant environmental information, such as the opportunity to reach a support that is worth climbing, where perception of affordances and support-tree location mechanisms constitute the climber-support coupled system, considered as a continuous cyclic loop.Citation33,Citation42,Citation117–123 In functional terms, plant orientation toward a potential support is similar to an animal running toward its prey,Citation2,Citation12,Citation122–126 suggesting the ability to process the features of the support, with a goal-directed and anticipatory behavior.Citation127 This study is the first one related to support-tree location by climbing plants in Chilean temperate rainforests.
Negative phototropism, with intrinsic variability while remaining stable and predictable across many climbing plant species, indicates growth or movement away from the light, based on light attenuation and evidenced with a directional pattern of plagiotropic (creeping) shoots and seedlings exposed to bright light, or even to low-light intensity.Citation38–40,Citation128–130 This directional pattern can be explained by the inverse square law, which states that light intensity is attenuated in inverse proportion to the square of the distance from the light source.Citation40,Citation131 Previous findings related with support-tree location and low-light availability as cueCitation33,Citation35 could be considered cases of negative phototropism.Citation42 Balcázar et al.Citation64 suggested that Heteropsis spp. randomly searched for support – without light availability or directional statistical measurements – because their seedlings were found ascending unsuitable support-trees. A random search effort implies pointless plant resource investmentCitation17; when climbing plants move toward a high light availability, they veer away from support.Citation44 Support-trees are a fundamental resource not regularly granted to woody climbers; they provide the darkest sector of the horizon in the chiaroscuro of the forest floor.Citation33 Negative phototropism, as an active response involving acquiring and processing information (light discrimination), allows climbing plants to be attracted and reach the darkest side of the support-tree base, to ascend toward the forest canopy.Citation38 We found in H. serratifolia juvenile shoots that low-light availability represents a search cue associated with a directional support-tree location pattern based on light attenuation (Appendix 1), exhibiting negative phototropism. The orientation and light received by searching (SS) and ascending (AS) shoots of H. serratifolia are similar between them (, ). These outcomes support our alternative work hypothesis and discard the fact that H. serratifolia juvenile searching (SS) and ascending (AS) shoots randomly search for support-trees. Negative phototropism greatly influences a shoot’s ability to reach a support-tree. Furthermore, H. serratifolia juvenile shoots based on our work hypothesis, the selection of those individuals toward some potential support-tree and not toward dead ends, and the results obtained express the possibility that this plant present light discrimination, as it has been reported in previous studiesCitation132–134 and may be a more refined process of perception and action, considered as cognitive behavior.Citation2,Citation127,Citation135
Support-tree location patterns of lianas (woody climbers) have been documented mainly in tropical,Citation33,Citation35,Citation51,Citation64,Citation83,Citation84 north temperate or subtropical forests.Citation81,Citation117,Citation118,Citation136–140 Factors including the characteristics of the support-tree, its proximity, and availability all strongly influence the selection by climbing plants.Citation33,Citation47,Citation81,Citation83,Citation141–146 Also, on the forest floor, under low-light availability, when H. serratifolia shoots do not find a host tree, they have been observed as transient erect shoots, as has been the case of Hydrangea petiolaris, which plays the role of a seedling bank,Citation147 thriving near the survival limit by its shade tolerance.Citation148 Our study, in a southern temperate rainforest, presents the inherent limitation of not controlling all the variables, including the microclimate (e.g., wind, moisture) and ecological factors (e.g., herbivory, competition) that could influence the circular distribution along with natural local scale orientation patterns.Citation98,Citation103 Plants can sense several cues, biotic and abiotic vectors, including electrical, magnetic, or volatile organic compounds.Citation2,Citation18,Citation149,Citation150 This means that noise must be added to the relationship between light and direction accuracy. The support-tree location pattern presented in this survey is not enough to explain the dominance of H. serratifoila, though it is an important contribution to understand its natural history. A probable answer to explain the dominance of H. serratifoila in this temperate rainforest could be based on its characteristic traits set like infestation rate, shade tolerance, adventitious root climber, clonal nonrandom foraging, and exhibition of negative phototropism (as light discrimination), that could confer an environmental advantage over other coexisting climbing plant species in this temperate rainforest, due the high abundance of support-trees in this forest.Citation74
Clonal growth in climbing plants allows plagiotropic shoots to spread horizontally and influences the nonrandom foraging behavior for resources.Citation4,Citation51,Citation54,Citation55,Citation151–153 This anticipatory behavior, and clonal ability, incorporates epigenetic memory of past, present information and environmental interactions.Citation85,Citation154–156 Shoot typologies aid in understanding the growth and branching patterns of woody climber stems, according to their dynamics in time and space.Citation51 If only present information prevailed, with no input from acquired memory, every juvenile shoot of H. serratifolia would start from scratch in the development of strategies to acquire its necessary resources; then the orientations of ascending and searching shoots of H. serratifolia should have differed in each growing season. An eternal and generational error-loop. The light discrimination, as negative phototropism exhibited by H. serratifolia juvenile shoots, could be a demonstration of anticipatory behavior, showing that searching shoots are not attracted to any dark site in the chiaroscuro of the forest floor, they are attracted to shadows in the understory to find a lighter environment like the canopy. However, studies in the climbing plant species of this temperate rainforest are still lacking when it comes to clonal genetic diversity, population structure,Citation157–159 physiological shoots integration,Citation160–162 community clonal growth and foraging.Citation163–166
Plant behavior,Citation6,Citation167–169 intelligenceCitation11,Citation123,Citation149,Citation167,Citation169,Citation170 and cognitionCitation2,Citation171 concepts have been discussed metaphorically and literally.Citation44,Citation45,Citation122,Citation126,Citation150,Citation172 Nonetheless, a prevailing misconstruction is that a precise scientific study is not viable without an unambiguous definition, even though the definition and the field of research could evolve together.Citation168,Citation173 The search for semantic purity in plant behavior has encouraged the tendency to reject important concepts and results in behavioral ecology, in the framework of ultimate causation.Citation122,Citation126,Citation167,Citation168,Citation172 Plant physiology cannot explain plant behavior before a phenomenon appears. It is far more interesting and relevant to understand how plants live under a plural approach, discovering morpho-physiological, cognitive processes and ecological causes and consequences of their sensory systems. This broad approach allows us to understand how the transmission and integration of cues occur in real time, leading to survival and adaptation in ever-changing environments.Citation174–176 Sensory biology is an emerging frontier in the study of the growth and functioning of climbing plantsCitation177 and the experimental test of the ocelli-based plant visionCitation132–134 would be the logical next step in our quest for understanding the plant sensory complexity. Future studies in H. serratifolia need to take into account the possibilities that (1) negative phototropism combined with the effect of gravitropism could guide and refine the growth in these plants and (2) how in physiological and behavior terms, H. serratifolia twin around toward the sunniest part of the support-tree and the concomitant switch in morphogenesis from juvenile to adult phase.
The selective foraging behavior response and resource acquisition pattern observed in H. serratifolia reflect its ability to adaptively assess, adjust and construct flexible information processes in ecological time, optimizing its performance in unpredictable and dynamic environments.Citation2,Citation7–11,Citation170,Citation178,Citation179 The genome level, with heritable variation, produces morphology and physiology, which sequentially guided by sensory information, develops behavior, and this, in turn, generates the fitness that selection can act upon.Citation180 Behavior is a morpho-physiological, inescapable sensory-guided consequence of natural selection, acting upon any living being, with an evolutionary function as an adaptive mechanism; a causal link exists between fitness and sensory systems.Citation1–3,Citation172,Citation181,Citation182 H. serratifolia explores the chiaroscuro on the forest floor, where low-light availability represents specific and anticipatory information regarding a potential support-tree (causal pattern), due to the inherent, invariant, and interactive climber-support coupled system. Negative phototropism, as light discrimination, provides and represents a parsimonious influence to guide the shoots of H. serratifolia toward the support-tree in the forest, cues of low-light availability (“how”), its direction (“where”), and encoding spatial and temporal (“when”) information in the heterogeneous light environment.
Geolocation information
The study location is “Parque Nacional Puyehue”, 40°39’ S/72°11’ W, central-southern Chile, at the western foothills of the Andes.
Supplemental Material
Download MS Word (7.9 MB)Acknowledgments
W. D. Rodríguez-Quintero would like to thank Marleny Quintero, Barbara Toledo, Dr Ernesto Gianoli and members of the Ecología Funcional Lab at the Universidad de Concepción. W. D. Rodríguez-Quintero was supported by CONICYT Grant 21140252 and FONDECYT 1181688
Disclosure statement
No potential conflict of interest was reported by the author(s).
Data availability statement
All data and/or R codes that support the results of this survey are available from the corresponding author upon reasonable request.
Supplementary material
Supplemental data for this article can be accessed online at https://doi.org/10.1080/15592324.2022.2122244
Additional information
Funding
References
- Mescher MC, Smith J, de Moraes CM. Host location and selection by holoparasitic plants. In: Plant-Environment Interactions. Berlin (Heidelberg): Springer; 2009. p. 101–11. doi:10.1007/978-3-540-89230-4_6.
- Calvo P, Trewavas A. Cognition and intelligence of green plants. Information for animal scientists. Biochem Biophys Res Comm. 2020. doi:10.1016/j.bbrc.2020.07.139.
- Kumar A, Memo M, Mastinu A, Wicke S. Plant behaviour: an evolutionary response to the environment? Plant Biol. 2020;22(6):961–970. doi:10.1111/plb.13149.
- Cain ML. Consequences of foraging in clonal plant species. Ecology. 1994;75(4):933–944. doi:10.2307/1939417.
- Cain ML, Dudle DA, Evans JP. Spatial models of foraging in clonal plant species. Am J Bot. 1996;83(1):76–85. doi:10.1002/j.1537-2197.1996.tb13877.x.
- Karban R. Plant behaviour and communication. Ecol Lett. 2008;11(7):727–739. doi:10.1111/j.1461-0248.2008.01183.x.
- Oborny B, Englert P. Plant growth and foraging for a patchy resource: a credit model. Ecol Model. 2012;234:20–30. doi:10.1016/j.ecolmodel.2011.12.017.
- Kelly CK. Plant foraging: a marginal value model and coiling response in Cuscuta subinclusa. Ecology. 1990;71(5):1916–1925. doi:10.2307/1937599.
- Kelly CK. Resource choice in Cuscuta europaea. Proc Natl Acad Sci. 1992;89(24):12194–12197. doi:10.1073/pnas.89.24.12194.
- Trewavas A. What is plant behaviour? Plant Cell Environ. 2009;32(6):606–616. doi:10.1111/j.1365-3040.2009.01929.x.
- Trewavas A. Plant behaviour and intelligence. OUP Oxford. 2014. doi:10.1093/acprof:oso/9780199539543.003.0022.
- Gianoli E. The behavioural ecology of climbing plants. AoB Plants. 2015:7. doi:10.1093/aobpla/plv013.
- Gruntman Gruntman M, Groß D, Májeková M, Tielbörger K. Decision-making in plants under competition. Nat Comm. 2017;8(1):2235. doi:10.1038/s41467-017-02147-2.
- Bloom AJ, Chapin IIIFS, Mooney HA. Resource limitation in plants-an economic analogy. Annu Rev Ecol Syst. 1985;16(1):363–392. doi:10.1146/annurev.es.16.110185.002051.
- Novoplansky A, Cohen D, Sachs T. How Portulaca seedlings avoid their neighbors. Oecologia. 1990;82(4):490–493. doi:10.1007/bf00319791.
- Novoplansky A. Developmental responses of portulaca seedlings to conflicting spectral signals. Oecologia. 1991;88(1):138–140. doi:10.1007/bf00328414.
- Hutchings MJ, de Kroon H. Foraging in plants: the role of morphological plasticity in resource acquisition. Adv Ecol Res. 1994;25:159–238. doi:10.1016/s0065-2504(08)60215-9.
- Runyon JB, Mescher MC, De Moraes CM. Volatile chemical cues guide host location and host selection by parasitic plants. Science. 2006;313(5795):1964–1967. doi:10.1126/science.1131371.
- Novoplansky A. Picking battles wisely: plant behaviour under competition. Plant Cell Environ. 2009;32(6):726–741. doi:10.1111/j.1365-3040.2009.01979.x.
- Iino M. Phototropism: mechanisms and ecological implications. Plant Cell Environ. 1990;13(7):633–650. doi:10.1111/j.1365-3040.1990.tb01080.x.
- Stanković B, Volkmann D, Sack FD. Autotropism, automorphogenesis, and gravity. Physiol Plantarum. 1998;102(2):328–335. doi:10.1034/j.1399-3054.1998.1020222.x.
- Iino M. Phototropism in higher plants. In: Comprehensive series in photosciences. Vol. 1. Elsevier; 2001. p. 659–811. doi:10.1016/s1568-461x(01)80027-2.
- Esmon CA, Pedmale UV, Liscum E. Plant tropisms: providing the power of movement to a sessile organism. Int J Dev Biol. 2005;49(5–6):665–674. doi:10.1387/ijdb.052028ce.
- Iino M. Toward understanding the ecological functions of tropisms: interactions among and effects of light on tropisms. Curr Opin Plant Biol. 2006;9(1):89–93. doi:10.1016/j.pbi.2005.11.012.
- de Kroon H, Visser EJ, Huber H, Mommer L, Hutchings MJ. A modular concept of plant foraging behaviour: the interplay between local responses and systemic control. Plant Cell Environ. 2009;32(6):704–712. doi:10.1111/j.1365-3040.2009.01936.x.
- Molas ML, Kiss JZ. Phototropism and gravitropism in plants. Adv Bot Res. 2009;49:1–34. doi:10.1016/s0065-2296(08)00601-0.
- Karban R. Plant sensing and communication. University of Chicago Press; 2015.
- Ballaré CL, Sánchez RA, Scopel AL, Casal JJ, Ghersa CM. Early detection of neighbour plants by phytochrome perception of spectral changes in reflected sunlight. Plant Cell Environ. 1987;10(7):551–557. doi:10.1111/j.1365-3040.1987.tb01835.x.
- Aphalo PJ, Ballare CL, Scopel AL. Plant-plant signalling, the shade-avoidance response and competition. J Exp Bot. 1999;50(340):1629–1634. doi:10.1093/jxb/50.340.1629.
- Ballaré CL. Keeping up with the neighbors: phytochrome sensing and other signalling mechanisms. Trends Plant Sci. 1999;4(3):97–102. doi:10.1016/s1360-1385(99)01408-9.
- Leuchner M, Hertel C, Rötzer T, Seifert T, Weigt R, Werner H, Menzel A. Solar radiation as a driver for growth and competition in forest stands. In: Growth and defence in plants. Berlin (Heidelberg): Springer; 2012. p. 175–191. doi:10.1007/978-3-642-30645-7_8.
- Gagliano M, Vyazovskiy VV, Borbély AA, Grimonprez M, Depczynski M. Learning by association in plants. Sci Rep-UK. 2016;6(1):1–9. doi:10.1038/srep38427.
- Strong J, Donald R, Ray J, Thomas S, Ray J, Thomas S, Thomas S. Host tree location behavior of a tropical vine (Monstera gigantea) by Skototropism. Science. 1975;190(4216):804–806. doi:10.1126/science.190.4216.804.
- Ray TS. Slow-motion world of plant “behavior” visible in rainforest. Smithsonian. 1979;9:121–130.
- Ray TS. Foraging behaviour in tropical herbaceous climbers (Araceae). J Ecol. 1992:189-203. doi:10.2307/2261006.
- Schmitt J, Stinchcombe JR, Heschel MS, Huber H. The adaptive evolution of plasticity: phytochrome-mediated shade avoidance responses. Integr Comp Biol. 2003;43(3):459–469. doi:10.1093/icb/43.3.459.
- Ballaré CL. Illuminated behaviour: phytochrome as a key regulator of light foraging and plant anti‐herbivore defence. Plant Cell Environ. 2009;32(6):713–725. doi:10.1111/j.1365-3040.2009.01958.x.
- Kato S, Hosoi K, Kawakubo N, Komiyama A. Negative phototropism of the creeping shoots in Japanese ivy, Hedera rhombea. J Jap For Soc. 2011;93(3):123–128. doi:10.4005/jjfs.93.123.
- Kato S, Kanematsu T, Kawakubo N, Komiyama A. Positive and negative phototropism in Schizophragma hydrangeoides and Parthenocissus tricuspidata. Jap J For Environ. 2012a;54:1–5.
- Kato S, Yamamoto T, Kawakubo N, Komiyama A. Responses of Trachelospermum asiaticum (Apocynaceae) seedlings to growth in a light intensity gradient. Ecol Res. 2012b;27(1):229–231. doi:10.1007/s11284-011-0871-y.
- Gagliano M, Renton M, Depczynski M, Mancuso S. Experience teaches plants to learn faster and forget slower in environments where it matters. Oecologia. 2014;175(1):63–72. doi:10.1007/s00442-013-2873-7.
- Kato S. Assessing negative and positive phototropism in lianas. In: Phototropism. New York (NY): Humana Press; 2019. p. 19–26. doi:10.1007/978-1-4939-9015-3_2.
- Wang Q, Guerra S, Ceccarini F, Bonato B, Castiello U. Sowing the seeds of intentionality: motor intentions in plants. Plant Signal Behav. 2021;16(11):1949818. doi:10.1080/15592324.2021.1949818.
- Darwin C. The movements and habits of climbing plants. John Murray. London; 1875.
- Darwin C. The power of movement in plants. Appleton; 1897.
- Ray TS. Cyclic heterophylly in Syngonium (Araceae). Am J Bot. 1987;74(1):16–26. doi:10.1002/j.1537-2197.1987.tb08575.x.
- Putz FE, Holbrook NM. Biomechanical studies of vines. In: Putz FE, Mooney HA, editors. The biology of vines. Cambridge: Cambridge University Press; 1991. p. 73–97.
- Larson KC. Circumnutation behavior of an exotic honeysuckle vine and its native congener: influence on clonal mobility. Am J Bot. 2000;87(4):533–538. doi:10.2307/2656597.
- Isnard S, Silk WK. Moving with climbing plants from Charles Darwin’s time into the 21st century. Am J Botany. 2009;96(7):1205–1221. doi:10.3732/ajb.0900045.
- Wyka TP, Zadworny M, Mucha J, Żytkowiak R, Nowak K, Oleksyn J. Species-specific responses of growth and biomass distribution to trellis availability in three temperate lianas. Trees. 2019;33(3):921–932. doi:10.1007/s00468-019-01828-3.
- Peñalosa J. Basal branching and vegetative spread in two tropical rain forest lianas. Biotropica. 1984;16(1):1–9. doi:10.2307/2387886.
- Sutherland WJ, Stillman RA. The foraging tactics of plants. Oikos. 1988:239-244. doi:10.2307/3565196.
- Schnitzer SA, Bongers F. Increasing liana abundance and biomass in tropical forests: emerging patterns and putative mechanisms. Ecol Lett. 2011;14(4):397–406. doi:10.1111/j.1461-0248.2011.01590.x.
- Ledo A, Schnitzer SA. Disturbance and clonal reproduction determine liana distribution and maintain liana diversity in a tropical forest. Ecology. 2014;95(8):2169–2178. doi:10.1890/13-1775.1.
- Mori H, Ueno S, Matsumoto A, Kamijo T, Tsumura Y, Masaki T. Large contribution of clonal reproduction to the distribution of deciduous liana species (Wisteria floribunda) in an old-growth cool temperate forest: evidence from genetic analysis. Ann Bot. 2018;121(2):359–365. doi:10.1093/aob/mcx153.
- Gianoli E. Maternal environmental effects on the phenotypic responses of the twining vine Ipomoea purpurea to support availability. Oikos. 2002;99(2):324–330. doi:10.1034/j.1600-0706.2002.990213.x.
- Gianoli E. Evolution of a climbing habit promotes diversification in flowering plants. Proc Roy Soc Lond Series B: Biological Sciences. 2004;271(1552):2011–2015. doi:10.1098/rspb.2004.2827.
- Gianoli E, Molina‐Montenegro MA. Leaf damage induces twining in a climbing plant. New Phytol. 2005;167(2):385–390. doi:10.1111/j.1469-8137.2005.01484.x.
- González‐Teuber M, Gianoli E. Damage and shade enhance climbing and promote associational resistance in a climbing plant. J Ecol. 2008;96(1):122–126. doi:10.1111/j.1365-2745.2007.01321.x.
- van der Heijden G, Phillips O. What controls liana success in neotropical forest? Global Ecol Biogeogr. 2008;17(3):372–383. doi:10.1111/j.1466-8238.2007.00376.x.
- Gianoli E, Carrasco-Urra F. Leaf mimicry in a climbing plant protects against herbivory. Curr Biol. 2014;24(9):984–987. doi:10.1016/j.cub.2014.03.010.
- Gianoli E. Lack of differential plasticity to shading of internodes and petioles with growth habit in Convolvulus arvensis (Convolvulaceae). Int J Plant Sci. 2001;162(6):1247–1252. doi:10.1086/322950.
- Sakai A, Nomiya H, Suzuki W. Horizontal distribution of stolons of a temperate liana Wisteria floribunda DC. and its ecological significance. J For Res. 2002;7(3):125–130. doi:10.1007/bf02762600.
- Balcázar‐Vargas MP, Peñuela‐Mora MC, van Andel TR, Zuidema PA. The quest for a suitable host: size distributions of host trees and secondary hemiepiphytes search strategy. Biotropica. 2012;44(1):19–26. doi:10.1111/j.1744-7429.2011.00767.x.
- Gentry AH. The distribution and evolution of climbing plants. In: Putz FE, Mooney HA, editors. The biology of vines. Cambridge: Cambridge University Press; 1991. p. 3–49.
- Dewalt SJ, Schnitzer SA, Denslow JS. Density and diversity of lianas along a chronosequence in a central Panamanian lowland forest. J Trop Ecol. 2000;16(1):1–9. doi:10.1017/s0266467400001231.
- Schnitzer SA, Bongers F, Bongers F. The ecology of lianas and their role in forests. Trends Ecol Evol. 2002;17(5):223–230. doi:10.1016/s0169-5347(02)02491-6.
- DeWalt SJ, Schnitzer SA, Chave J, Bongers F, Burnham RJ, Cai Z, Chuyong GB, Clark D, Ewango CNE, Gerwing JJ, et al. Annual rainfall and seasonality predict pan‐tropical patterns of liana density and basal area. Biotropica. 2010;42(3):309–317. doi:10.1111/j.1744-7429.2009.00589.x.
- Gianoli E, Saldaña A, Jiménez-Castillo M, Valladares F. Distribution and abundance of vines along the light gradient in a southern temperate rain forest. J Veg Sci. 2010;21(1):66–73. doi:10.1111/j.1654-1103.2009.01124.x.
- Tang Y, Kitching RL, Cao M. Lianas as structural parasites: a re-evaluation. Chin Sci Bull. 2012;57(4):307–312. doi:10.1007/s11434-011-4690-x.
- DeWalt SJ, Schnitzer SA, Alves LF, Bongers F, Burnham RJ, Cai Z, Carson WP, Chave J, Chuyong GB, Costa FRC, et al. Biogeographical patterns of liana abundance and diversity. Ecology of Lianas. 2015;131–146.
- Schnitzler A, Amigo J, Hale B, Schnitzler C. Patterns of climber distribution in temperate forests of the Americas. J Plant Ecol. 2016;9(6):724–733. doi:10.1093/jpe/rtw012.
- Carrasco‐Urra F, Gianoli E. Abundance of climbing plants in a southern temperate rain forest: host tree characteristics or light availability? J Veg Sci. 2009;20(6):1155–1162. doi:10.1111/j.1654-1103.2009.01115.x.
- JIMÉNEZ-CASTILLO M, Lusk CH. Host infestation patterns of the massive liana hydrangea serratifolia (hydrangeaceae) in a Chilean temperate rainforest. Aust Ecol. 2009;34(7):829–834. doi:10.1111/j.1442-9993.2009.01990.x.
- Gianoli E, Saldaña A, Jiménez-Castillo M. Ecophysiological traits may explain the abundance of climbing plant species across the light gradient in a temperate rainforest. PloS one. 2012;7(6):e38831. doi:10.1371/journal.pone.0038831.
- Marticorena A, Alarcón D, Abello L, Atala C. Plantas trepadoras, epífitas y parásitas nativas de Chile. Guía de Campo. 2010.
- Almeyda E, Sáez F. Recopilación de datos climáticos de Chile y mapas sinópticos respectivos. Santiago (Chile): Ministerio de Agricultura; 1958.
- Armesto J, Villagrán C, Donoso C. La historia del bosque templado chileno. Ambiente y Desarrollo. 1994;10:66–72.
- Armesto J, León-Lobos P, Arroyo MK. Los bosques templados del sur de Chile y Argentina: una isla biogeográfica. In: Armesto JJ, Villagrán C, Arroyo MK, editors. Ecología de los bosques nativos de Chile. Santiago (Chile): Editorial Universitaria; 1996. p. 23–28.
- Roeder M, Slik JF, Harrison RD, Paudel E, Tomlinson KW. Proximity to the host is an important characteristic for selection of the first support in lianas. J Veg Sci. 2015;26(6):1054–1060. doi:10.1111/jvs.12316.
- Gerwing JJ, Schnitzer SA, Burnham RJ, Bongers F, Chave J, DeWalt SJ, Ewango CEN, Foster R, Kenfack D, Martínez-Ramos M, et al. A standard protocol for liana censuses 1. Biotropica. 2006;38(2):256–261. doi:10.1111/j.1744-7429.2006.00134.x.
- Schnitzer SA, DeWalt SJ, Chave J. Censusing and measuring lianas: a quantitative comparison of the common methods 1. Biotropica. 2006;38(5):581–591. doi:10.1111/j.1744-7429.2006.00187.x.
- Campanello PI, Garibaldi JF, Gatti MG, Goldstein G. Lianas in a subtropical Atlantic forest: host preference and tree growth. For Ecol Manag. 2007;242(2):250–259. doi:10.1016/j.foreco.2007.01.040.
- Castagneri D, Garbarino M, Nola P. Host preference and growth patterns of ivy (Hedera helix L.) in a temperate alluvial forest. Plant Ecol. 2013;214(1):1–9. doi:10.1007/s11258-012-0130-5.
- Louâpre P, Bittebière AK, Clément B, Pierre JS, Mony C, Moora M. How past and present influence the foraging of clonal plants? PLoS One. 2012;7(6):e38288. doi:10.1371/journal.pone.0038288.
- Michmizos D, Hilioti Z. A roadmap towards a functional paradigm for learning & memory in plants. J Plant Physiol. 2019;232:209–215. doi:10.1016/j.jplph.2018.11.002.
- Valladares F, Gianoli E, Saldana A. Climbing plants in a temperate rainforest understorey: searching for high light or coping with deep shade? Ann Bot. 2011;108(2):231–239. doi:10.1093/aob/mcr132.
- Siderhurst LA, Griscom HP, Hudy M, Bortolot ZJ. Changes in light levels and stream temperatures with loss of eastern hemlock (Tsuga canadensis) at a southern Appalachian stream: implications for brook trout. For Ecol Manage. 2010;260(10):1677–1688. doi:10.1016/j.foreco.2010.08.007.
- Clark DB, Clark DA, Rich PM. Comparative analysis of microhabitat utilization by saplings of nine tree species in neotropical rain forest. Biotropica. 1993;25(4):397–407. doi:10.2307/2388863.
- Batschelet E. Circular statistics in biology. London: Academic Press; 1981.
- Fisher NI. Statistical analysis of circular data. Cambridge University Press. 1995.
- Jammalamadaka SR, Sengupta A. Topics in circular statistics. Vol. 5, Singapore: World Scientific; 2001.
- Pewsey A, Neuhäuser M, Ruxton GD. Circular statistics in R. Oxford University Press; 2013.
- Mardia KV, Jupp PE. Directional statistics. John Wiley & Sons; 2009. Vol. 494.
- Jona Lasinio G, Santoro M, Mastrantonio G. CircSpaceTime: an R package for spatial and spatio-temporal modelling of circular data. J Stat Comput Simul. 2020;90(7):1315–1345. doi:10.1080/00949655.2020.1725008.
- Pewsey A, García-Portugués E. Recent advances in directional statistics. TEST. 2021;30:1–58. doi:10.1007/s11749-021-00759-x.
- Drezner TD. Plant facilitation in extreme environments: the non-random distribution of saguaro cacti (Carnegiea gigantea) under their nurse associates and the relationship to nurse architecture. J Arid Environ. 2006;65(1):46–61. doi:10.1016/j.jaridenv.2005.06.027.
- García-González A, Damon A, Riverón-Giró FB, Ávila-Díaz I. Circular distribution of three species of epiphytic orchids in shade coffee plantations, in Soconusco, Chiapas, Mexico. Plant Ecol Evol. 2016;149(2):189–198. doi:10.5091/plecevo.2016.1150.
- Ekelund N, Häder DP. Photomovement and photobleaching in two Gyrodinium species. Plant Cell Physiol. 1988;29(7):1109–1114. doi:10.1093/oxfordjournals.pcp.a077610.
- Barros AM, Pereira JM, Lund UJ. Identifying geographical patterns of wildfire orientation: a watershed-based analysis. For Ecol Manage. 2012;264:98–107. doi:10.1016/j.foreco.2011.09.027.
- Landler L, Ruxton GD, Malkemper EP. Circular data in biology: advice for effectively implementing statistical procedures. Behav Ecol Sociobiol. 2018;72(8):128. doi:10.1007/s00265-018-2538-y.
- Morellato LPC, Alberti LF, Hudson IL. Applications of circular statistics in plant phenology: a case studies approach. In: Phenological research. Dordrecht: Springer; 2010. p. 339–359. doi:10.1007/978-90-481-3335-2_16.
- Tremblay RL, Velázquez-Castro J. Circular distribution of an epiphytic herb on trees in a subtropical rain forest. Trop Ecol. 2009;50:211.
- Agostinelli C, Lund U. R package circular: circular statistics (version 0.4-93). CA: department of environmental sciences, informatics and statistics, Ca’ Foscari University, Venice, Italy. UL: Department of Statistics, California Polytechnic State University, San Luis Obispo, California (USA). Available from: https://r-forge.r-project.org/projects/circular/ (2017).
- Fisher NI, Lee AJ. A correlation coefficient for circular data. Biometrika. 1983;70(2):327–332. doi:10.1093/biomet/70.2.327.
- Fisher NI, Lee AJ. Regression models for an angular response. Biometrics. 1992;48(3):665–677. doi:10.2307/2532334.
- Kempter R, Leibold C, Buzsáki G, Diba K, Schmidt R. Quantifying circular–linear associations: hippocampal phase precession. J Neurosci Met. 2012;207(1):113–124. doi:10.1016/j.jneumeth.2012.03.007.
- Zar JH. Biostatistical analysis. India: Pearson Education; 1999.
- R Core Team. R: a language and environment for statistical computing. R Foundation for Statistical Computing, Vienna (Austria). URL https://www.R-project.org/ (2022).
- Lund U, Agostinelli C. CircStats: circular statistics, from “topics in circular statistics” ( 2001) (Rpackage version 0.2-6). Available from: https://CRAN.R-project.org/package=CircStats. (2018).
- Oliveira Pérez M, Crujeiras Casais RM, Rodríguez Casal A. NPCirc: an R package for nonparametric circular methods. Am Stat Assoc. 2014. doi:10.18637/jss.v061.i09.
- Buttarazzi D, Pandolfo G, Porzio GC. A boxplot for circular data. Biometrics. 2018;74(4):1492–1501. doi:10.1111/biom.12889.
- Di Marzio M, Panzera A, Taylor CC. Non‐parametric regression for circular responses. Scand J Stat. 2013;40(2):238–255. doi:10.1111/j.1467-9469.2012.00809.x.
- Guerra S, Peressotti A, Peressotti F, Bulgheroni M, Baccinelli W, D’Amico E, Gómez A, Massaccesi S, Ceccarini F, Castiello U. Flexible control of movement in plants. Sci Rep-Uk. 2019;9(1):1–9. doi:10.1038/s41598-019-53118-0.
- Ceccarini F, Guerra S, Peressotti A, Peressotti F, Bulgheroni M, Baccinelli W, Bonato B, Castiello U. Speed–accuracy trade-off in plants. Psychon Bull Rev. 2020;27(5):966–973. doi:10.3758/s13423-020-01753-4.
- Ceccarini F, Guerra S, Peressotti A, Peressotti F, Bulgheroni M, Baccinelli W, Bonato B, Castiello U. On-line control of movement in plants. Biochem Biophys Res Comm. 2020. doi:10.31234/osf.io/svkc3.
- Melzer B, Steinbrecher T, Seidel R, Kraft O, Schwaiger R, Speck T. The attachment strategy of English ivy: a complex mechanism acting on several hierarchical levels. J Roy Soc Interf. 2010;7(50):1383–1389. doi:10.1098/rsif.2010.0140.
- Melzer B, Seidel R, Steinbrecher T, Speck T. Structure, attachment properties, and ecological importance of the attachment system of English ivy (Hedera helix). J Exp Bot. 2012;63(1):191–201. doi:10.1093/jxb/err260.
- Calvo P, Martín E, Symons J. 16 the emergence of systematicity in minimally cognitive agents. The Architecture of Cognition: Rethinking Fodor and Pylyshyn’s Systematicity Challenge. 2014:397. doi:10.7551/mitpress/9559.003.0021.
- Calvo P, Baluška F, Sims A. “Feature detection” vs. “predictive coding” models of plant behavior. Front Psych. 2016;7:1505. doi:10.3389/fpsyg.2016.01505.
- Calvo P, Friston K. Predicting green: really radical (plant) predictive processing. J. R. Soc. Interface. 2017;14(131):20170096. doi:10.1098/rsif.2017.0096.
- Calvo P, Raja V, Lee DN. Guidance of circumnutation of climbing bean stems: an ecological exploration. BioRxiv. 2017:122358. doi:10.1101/122358.
- Abramson CI, Calvo P. General issues in the cognitive analysis of plant learning and intelligence. In: Memory and learning in plants. Cham: Springer; 2018. p. 35–49. doi:10.1007/978-3-319-75596-0_3.
- Lee DN. Guiding Movement by Coupling Taus. Ecol Psychol. 1998;10:221–250.
- Lee DN, Bootsma RJ, Frost BJ, Land M, Regan D. General tau theory: evolution to date. Special issue: landmarks in perception. Perception. 2009;38(6):837–858. doi:10.1068/pmklee.
- Carello C, Vaz D, Blau JJ, Petrusz S. Unnerving intelligence. Ecol Psychol. 2012;24(3):241–264. doi:10.1080/10407413.2012.702628.
- Segundo-Ortin M, Calvo P. Are plants cognitive? A reply to Adams. Stud Hist Philos Sci, Part A. 2019;73:64–71. doi:10.1016/j.shpsa.2018.12.001.
- Kataoka H. Negative phototropism of Vaucheria terrestris regulated by calcium II. Inhibition by Ca2+-channel blockers and mimesis by A23187. Plant Cell Physiol. 1990;31(7):933–940. doi:10.1093/oxfordjournals.pcp.a078007.
- Kataoka H, Takahashi F, Ootaki T. Bimodal polarotropism of Vaucheria to polarized blue light: parallel polarotropism at high fluence rate corresponds to negative phototropism. J Plant Res. 2000;113(1):1–10. doi:10.1007/pl00013908.
- Kataoka H. Quantitative analysis of tip growth, phototropic responses, and other blue light-dependent photoresponses of vaucheria. In: Phototropism. New York (NY): Humana Press; 2019. p. 83–120. doi:10.1007/978-1-4939-9015-3_8.
- Fei L, Zhang Q, Deng Y. Identifying influential nodes in complex networks based on the inverse-square law. Phys A: Stat Mech Appl. 2018;512:1044–1059. doi:10.1016/j.physa.2018.08.135.
- Wagner H. The perception of light in plants. Ann Bot. 1909;23(3):459–489. doi:10.1093/oxfordjournals.aob.a089231.
- Mancuso S, Baluška F. Plant ocelli for visually guided plant behavior. Trends Plant Sci. 2017;22(1):5–6. doi:10.1016/j.tplants.2016.11.009.
- White J, Yamashita F. Boquila trifoliolata mimics leaves of an artificial plastic host plant. Plant Signal Behav. 2022;17(1). doi:10.1080/15592324.2021.1977530.
- Calvo Garzon F. The quest for cognition in plant neurobiology. Plant Signal Behav. 2007;2(4):208–211. doi:10.4161/psb.2.4.4470.
- Collins BS, Wein GR. Understory vines: distribution and relation to environment on a southern mixed hardwood site. B Torrey Bot Club. 1993:38-44. doi:10.2307/2996661.
- Metcalfe DJ. Hedera helix L. J Ecol. 2005;93(3):632–648. doi:10.1111/j.1365-2745.2005.01021.x.
- Ladwig LM, Meiners SJ. Spatiotemporal dynamics of lianas during 50 years of succession to temperate forest. Ecology. 2010;91(3):671–680. doi:10.1890/08-1738.1.
- Leicht-Young SA, Pavlovic NB, Frohnapple KJ, Grundel R. Liana habitat and host preferences in northern temperate forests. For Ecol Manage. 2010;260(9):1467–1477. doi:10.1016/j.foreco.2010.07.045.
- Mori H, Kamijo T, Masaki T. Liana distribution and community structure in an old-growth temperate forest: the relative importance of past disturbances, host trees, and microsite characteristics. Plant Ecol. 2016;217(10):1171–1182. doi:10.1007/s11258-016-0641-6.
- Putz FE. The natural history of lianas on Barro Colorado Island, Panama. Ecology. 1984;65(6):1713–1724. doi:10.2307/1937767.
- Campbell EJF, Newbery D. Ecological relationships between lianas and trees in lowland rain forest in Sabah, East Malaysia. J Trop Ecol. 1993;9:469–490. doi:10.1017/s0266467400007549.
- Talley SM, Setzer WM, Jackes BR. Host associations of two adventitious-root-climbing vines in a north Queensland tropical rain forest. Biotropica. 1996;28(3):356–366. doi:10.2307/2389199.
- Schnitzer SA, Dalling JW, Carson WP. The impact of lianas on tree regeneration in tropical forest canopy gaps: evidence for an alternative pathway of gap‐phase regeneration. J Ecol. 2000;88(4):655–666. doi:10.1046/j.1365-2745.2000.00489.x.
- Nabe-Nielsen J. Diversity and distribution of lianas in a neotropical rain forest, Yasuní National Park, Ecuador. J Trop Ecol. 2001;17(1):1–19. doi:10.1017/s0266467401001018.
- Schnitzer SA, Carson WP. Treefall gaps and the maintenance of species diversity in a tropical forest. Ecology. 2001;82(4):913–919. doi:10.1890/0012-9658(2001)082[0913:tgatmo]2.0.co;2.
- Kato S, Morito H, Hanaoka S, Komiyama A. Relationship between distribution of erect shoots in two root-climbing lianas Schizophragma hydrangeoides and Hydrangea petiolaris (Saxifragaceae) and light environment on the forest floor. Jap J For Environ. 2014;56:49–54.
- Gommers CM, Visser EJ, St Onge KR, Voesenek LA, Pierik R. Shade tolerance: when growing tall is not an option. Trends Plant Sci. 2013;18(2):65–71. doi:10.1016/j.tplants.2012.09.008.
- Trewavas A. Intelligence, cognition, and language of green plants. Front Psychol. 2016;7:588. doi:10.3389/fpsyg.2016.00588.
- Calvo P, Trewavas A. Physiology and the (neuro)biology of plant behavior: a farewell to arms. Trends in Plant Sci. 2020;25(3):214–216. doi:10.1016/j.tplants.2019.12.016.
- Evans JP, Cain ML. A spatially explicit test of foraging behavior in a clonal plant. Ecology. 1995;76(4):1147–1155. doi:10.2307/1940922.
- Schnitzer SA, Mangan SA, Dalling JW, Baldeck CA, Hubbell SP, Ledo A, Muller-Landau HC, Tobin MF, Aguilar S, Brassfield D, et al. Liana abundance, diversity, and distribution on Barro Colorado Island, Panama. PLoS one. 2012;7(12):e52114. doi:10.1371/journal.pone.0052114.
- Waters EM, Watson MA. Live substrate positively affects root growth and stolon direction in the woodland strawberry, Fragaria vesca. Front Plant Sci. 2015;6:814. doi:10.3389/fpls.2015.00814.
- Latzel V, Rendina González AP, Rosenthal J. Epigenetic memory as a basis for intelligent behavior in clonal plants. Front Plant Sci. 2016;7:1354. doi:10.3389/fpls.2016.01354.
- Latzel V, Münzbergová Z. Anticipatory behavior of the clonal plant Fragaria vesca. Front Plant Sci. 2018;9:1847. doi:10.3389/fpls.2018.01847.
- Rendina-González AP, Preite V, Verhoeven KJ, Latzel V. Transgenerational effects and epigenetic memory in the clonal plant Trifolium repens. Front Plant Sci. 2018;9:1677. doi:10.3389/fpls.2018.01677.
- Arnaud‐Haond S, Duarte CM, Alberto F, Serrao EA. Standardizing methods to address clonality in population studies. Molec Ecol. 2007;16(24):5115–5139. doi:10.1111/j.1365-294x.2007.03535.x.
- Torres-Diaz C, Ruiz E, Salgado-Luarte C, Molina-Montenegro MA, Gianoli E. Within-population genetic diversity of climbing plants and trees in a temperate forest in central Chile. Gayana Bot. 2013;70(1):36–43. doi:10.4067/s0717-66432013000100005.
- Kamvar ZN, Brooks JC, Grünwald NJ. Novel R tools for analysis of genome-wide population genetic data with emphasis on clonality. Front Genetics. 2015;6:208. doi:10.3389/fgene.2015.00208.
- Huber H, Hutchings MJ. Differential response to shading in orthotropic and plagiotropic shoots of the clonal herb Glechoma hirsuta. Oecologia. 1997;112(4):485–491. doi:10.1007/s004420050336.
- Song YB, Yu FH, Keser LH, Dawson W, Fischer M, Dong M, van Kleunen M. United we stand, divided we fall: a meta-analysis of experiments on clonal integration and its relationship to invasiveness. Oecologia. 2013;171(2):317–327. doi:10.1007/s00442-012-2430-9.
- Liu F, Liu J, Dong M. Ecological consequences of clonal integration in plants. Front Plant Sci. 2016;7:770. doi:10.3389/fpls.2016.00770.
- Alpert P. Does clonal growth increase plant performance in natural communities? Abstracta Botanica. 1995;11–16.
- Oborny B, Bartha S. Clonality in plant communities-an overview. Abstracta Botanica. 1995;115–127.
- Eilts JA, Mittelbach GG, Reynolds HL, Gross KL. Resource heterogeneity, soil fertility, and species diversity: effects of clonal species on plant communities. Am Nat. 2011;177(5):574–588. doi:10.1086/659633.
- Klimešová J, Herben T. Clonal and bud bank traits: patterns across temperate plant communities. J Veg Sci. 2015;26(2):243–253. doi:10.1111/jvs.12228.
- Cvrčková F, Lipavská H, Žárský V. Plant intelligence: why, why not or where? Plant Signal Behav. 2009;4(5):394–399. doi:10.4161/psb.4.5.8276.
- Cvrčková F, Žárský V, Markoš A. Plant studies may lead us to rethink the concept of behavior. Front Psychol. 2016;7:622. doi:10.3389/fpsyg.2016.00622.
- Calvo P, Gagliano M, Souza GM, Trewavas A. Plants are intelligent, here’s how. Ann Bot. 2020;125(1):11–28. doi:10.1093/aob/mcz155.
- van Loon LC, van Loon LC. The intelligent behavior of plants. Trends Plant Sci. 2016;21(4):286–294. doi:10.1016/j.tplants.2015.11.009.
- Parise AG, Gagliano M, Souza GM. Extended cognition in plants: is it possible? Plant Signal Behav. 2020;15(2):1710661. doi:10.1080/15592324.2019.1710661.
- Cahill JF. The inevitability of plant behavior. Am J Bot. 2019;106(7):903–905. doi:10.1002/ajb2.1313.
- Miguel-Tomé S, Llinás RR. Broadening the definition of a nervous system to better understand the evolution of plants and animals. Plant Signal Behav. 2021:1927562. doi:10.1080/15592324.2021.1927562.
- Scorza LCT, Dornelas MC. Plants on the move: towards common mechanisms governing mechanically-induced plant movements. Plant Signal Behav. 2011;6(12):1979–1986. doi:10.4161/psb.6.12.18192.
- Chamovitz DA. Plants are intelligent; now what? Nature Plants. 2018;4(9):622–623. doi:10.1038/s41477-018-0237-3.
- Chamovitz D. What a Plant Knows: a Field Guide to the Senses, Updated and Expanded ed. Scientific American/Farrar, Straus and Giroux; 2020.
- Sousa‐Baena MS, Hernandes-Lopes J, Van Sluys MA. Reaching the top through a tortuous path: helical growth in climbing plants. Curr Op Plant Biol. 2021;59:101982. doi:10.1016/j.pbi.2020.101982.
- Trewavas A. Green plants as intelligent organisms. Trends Plant Sci. 2005;10(9):413–419. doi:10.1016/j.tplants.2005.07.005.
- Marder M. Plant intelligence and attention. Plant Signal Behav. 2013;8(5):e23902. doi:10.4161/psb.23902.
- Nilsson DE. Eye evolution and its functional basis. Vis Neurosci. 2013;30(1–2):5–20. doi:10.1017/s0952523813000035.
- Muñoz-Parra E, Salmerón Barrera G, Ruiz-Herrera LF, Valencia-Cantero E, López-Bucio J. Self-plant perception via long-distance signaling. Plant Signal Behav. 2017;12(12):e1404218. doi:10.1080/15592324.2017.1404218.
- Parise AG, Reissig GN, Basso LF, Schultz LG, de Carvalho Oliveira TF, de Toledo Gr GRA, Silva Ferreira A, Maia Souza G, Oliveira TFDC. Detection of different hosts from a distance alters the behaviour and bioelectrical activity of Cuscuta racemosa. Front Plant Sci. 2021;12:594195. doi:10.3389/fpls.2021.594195.
Appendix 1.
Linear representation of Local-Linear estimators (black line) for H. serratifolia juvenile searcher (SS) and ascending shoots (AS) used to model the angles regarding the light availability received.
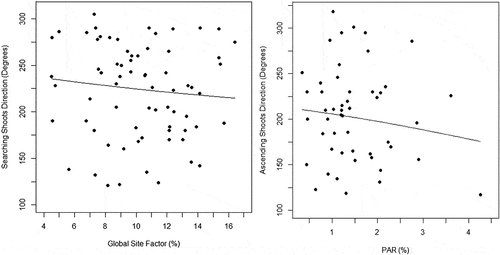