Abstract
The free radical copolymerization of glycidyl methacrylate (GMA) and octadecyl acrylate (ODA) was carried out at 70 °C in solution using toluene as solvent and 2,2′-azobisisobutyronitrile (AIBN) as initiator. The copolymer compositions were determined using 1H NMR spectroscopy. The monomer reactivity ratios were computed using five linear methods at low conversions, i.e. Mayo–Lewis (ML), Fineman–Ross (FR), inverted Fineman-Ross (IFR), Ezrielev–Brokhina– Roskin (EBR) and Kelen–Tudos (KT). The reactivity ratios resulted from different methods are in agreement with each other and showed higher reactivity for GMA monomer in comparison with ODA monomer (FR: r G = 1.29 and r O = 0.68). Crosslinked GMA–ODA copolymers were also prepared using ethylene glycol dimethacrylate as a crosslinker. Thermal properties and swelling behaviors of crosslinked copolymers in different polar and nonpolar solvents were evaluated. GMA incorporation in the crosslinked copolymers improved T g values, while decreased T m quantities and swelling in nonpolar solvents. The swelling values are in the acceptable range and the prepared functional crosslinked copolymers can be used for nonpolar solvents absorbency applications.
Introduction
In the recent years, the synthesis of polymers with active functional groups as side substituents of the main chain has been an active field of research in polymer science. These kinds of macromolecules possess significant properties from both a fundamental and an applied point of view Citation[1,2]. On this matter, (co)polymerization of monomers comprising functional groups is the most successful method implemented for preparing functional polymers Citation[3]. Glycidyl methacrylate (GMA) is one of the interesting functional monomers with a wide range of industrial applications. Polymers based on GMA have been utilized as surface coatings Citation[4], leather adhesives Citation[5], pharmaceutical use for drug delivery Citation[6], dental composites Citation[7], superabsorbents Citation[8], ion exchanger resin Citation[9], nonlinear optical material Citation[10], compatibilizer Citation[11], and surface modifier Citation[12]. The greatest advantage of GMA-based polymers is the presence of an easily transformable epoxy group, which permits a large number of chemical reactions Citation[13,14]. The reaction of oxirane group with various nucleophiles is an opportunity for chemical modification of the base polymer Citation[15–18].
Long-chain alkyl (meth)acrylates, such as octadecyl acrylate (ODA), are a class of acrylic monomers that have a good affinity for nonpolar solvents or oil. Due to lipophilic nature of long-chain alkyl groups, polymers based on these monomers find widespread application to produce pour point depressants Citation[19], self-assembling amphiphilic polymers Citation[20], amphoteric polymers Citation[21], particle stabilizers Citation[22], and lipophilic gels to remove oil pollutions Citation[23,24]. However, poly(octadecyl acrylate) has a tendency to be in a crystalline state Citation[25,26], long alkyl side chains may crystallize in spite of amorphous backbone, which decreases their oil absorbency. To reduce this undesirable effect, the method of copolymerization with other monomers is used so that amorphous copolymers can be obtained Citation[27].
In the study of copolymerization, monomer reactivity ratios are very important parameters from either academical or industrial point of view, especially in tailor-made copolymers with desired physical and chemical properties and in evaluation of their specific final applications Citation[28]. Monomer reactivity ratios offer information about relative reactivity of monomer pairs, elucidation of copolymer composition, and monomer sequence distribution. They are also useful to understand the kinetic and mechanistic aspects of copolymerization Citation[3]. For this purpose, in the past few decades, 1H NMR spectroscopic analysis has played a dominant role in the knowing polymer stereochemistry and has been established as a powerful tool for the estimation of copolymer composition Citation[3,24,27,29] as well as for the determination of tacticity Citation[30] and sequence distribution due to its simplicity, rapidity, and sensitivity.
This article presents synthesis and characterization studies of linear and crosslinked GMA–ODA copolymers. The copolymers were characterized through 1H NMR, FTIR, differential scanning calorimetry (DSC) measurements, and solubility test. As the first goal, the monomer reactivity ratios were determined using five conventional methods (i.e. Mayo–Lewis [ML] Citation[31], Fineman–Ross [FR] Citation[32], inverted Fineman–Ross [IFR] Citation[32], Ezrielev–Brokhina– Roskin [EBR] Citation[33] and Kelen–Tudos [KT] Citation[34]). Investigating the influence of comonomer feed ratio on the thermal and swelling properties of crosslinked copolymers was the second goal of this study.
Experimental
Materials
GMA (Merck) was purified by distillation under reduced pressure. ODA (free of inhibitor, Aldrich) as monomer and 1,2-ethylene glycol dimethacrylate (EGDMA, Merck) as crosslinker were used as received. The 2,2′-azobisisobutyronitrile (AIBN, Merck) as initiator was recrystallized from ethanol and dried under vacuum before use. Tetrahydrofurane (THF), benzene, and toluene as solvents and ethanol as nonsolvent (all from Merck) were used after purification by distillation.
Synthesis of linear GMA-ODA copolymers
GMA and ODA with different comonomer feed ratios were dissolved in toluene with a concentration of 0.347–0.605 mol/l (5 g comonomers per 50 ml toluene). The solution was bubbled with nitrogen for 10 min and poured into a standard test tube (as a reactor) containing a magnetic stirrer. AIBN as an initiator, 2% based on the weight of the comonomers, was added to the solution after being heated at 70 °C. To keep monomer conversion below 12%, the polymerization was inhibited by adding hydroquinone (10% ethanol solution, 10 ml) after 30–60 s. The products were poured into excess ethanol with stirring. The obtained precipitate was filtered, washed three times with toluene/ethanol solution (50/50), and dried under vacuum at 40 °C to a constant weight. The exact weights of the reagents and the molar percent of GMA monomer in the feed and in the copolymer are summarized in Table .
Table 1. Reaction parameters for linear GMA–ODA copolymers.
Synthesis of crosslinked GMA–ODA copolymers
The comonomers (10 g) with different molar ratios were mixed together with 4% EGDMA crosslinker (w/w) and 100 ml toluene. The solution was bubbled with nitrogen for 10 min and poured into a standard test tube containing a magnetic stirrer. After being heated at 70 °C, 2% AIBN was added to the monomeric solution. The reaction was continued until gel formation. Produced gels were cut into small pieces and dried in a vacuum oven at 70 °C for 7 h. The exact weights of the reagents and the corresponding molar percent of comonomers in the feed are given in Table .
Table 2. Reaction parameters for crosslinked GMA–ODA copolymers.
Characterization
Monomer conversion was determined gravimetrically Citation[35] according to Equation (1):
where W p and W m are weight of produced polymers and initial weight of monomers in the feed, respectively.
The 1H NMR spectra of linear copolymers were recorded by a 400 MHz FT NMR spectrometer (Bruker Instruments, model Advance 400, Germany) at room temperature using CDCl3 as a solvent. Infrared spectra were obtained on a FTIR spectrometer (Bruker Instruments, model Aquinox 55, Germany) in the 4000–400 cm−1 range at a resolution of 0.5 cm−1. Thermal transitions of the crosslinked copolymers were recorded with DSC (Netzach, model 200F-3 Maia, Germany) at heating rate of 10 °C/min under nitrogen atmosphere. Samples were initially heated from 20 to 120 °C then cooled to −80 °C and again heated to 400 °C. Second scan was reported. The T g values were estimated as the temperatures at the inflection-point of the change in the base-line.
For determining the gel content of crosslinked copolymers, 20 mg of sample was swelled in excess amount of toluene as an extraction solvent for 48 h. The swollen gel was filtered and dried at 60 °C in a vacuum oven to a constant weight. Gel content values were calculated as following:
where W d and W i are the weights of dried and initial sample, respectively.
To study the potential absorbency of crosslinked copolymers for various nonpolar and polar organic solvents, i.e. benzene, toluene, crude oil (diluted with toluene, 10%), and THF, 0.10 g of dried sample was separately immersed in each of solvents for 6 h at room temperature. Then, swollen gel was removed, drained for 30 s, taped with filter paper to remove excess solvent and finally weighed. The swelling ratio (g/g) of the copolymers was determined according to Equation (3):
where W s and W d are the weights of swollen and dried sample, respectively.
Results and characterization
The present study aimed to synthesize functional copolymers based on GMA and ODA monomers containing both hydrophobic (octadecyl moiety) and functional reactive (epoxy moiety) groups. To this end, linear copolymers of GMA and ODA were initially prepared to determine the monomer reactivity ratios of them during free radical solution polymerization. Then, a series of crosslinked copolymers with various comonomer ratios were prepared to investigate their potential application for organic solvent absorbency.
Copolymer composition
To determine the monomer reactivity ratios of GMA and ODA, a number of linear copolymers were prepared at low degree of conversion (Scheme ). 1H NMR analysis was used to find out the copolymer compositions of prepared samples.
In Figure , a representative 1H NMR spectrum of L3 sample is typically depicted. The chemical structure of prepared copolymer is represented and all recorded peaks were indicated in the structure. The peaks related to geminal protons of methylene groups (–CH2–) of epoxy moiety (appeared at 2.62 and 2.81 ppm) and the singlet peak belonging to middle methylene groups of octadecyl moiety (appeared at 1.24 ppm) were used as indicative peaks for GMA and ODA contents in the copolymers, respectively. Mole percent of GMA in the structures of copolymers were calculated by using the indicative peaks integrations, and are summarized in Table .
Reactivity ratios
Knowledge of the reactivity ratios is the key to predict the copolymer composition and monomer sequence distribution. On this point, from the comonomer feed ratios and the determined copolymer compositions, the monomer reactivity ratios of GMA and ODA were calculated by five conventional linear methods based on Equation (4) (ML method Citation[31]), Equations (5) and (6) (FR and IFR methods Citation[32]), Equation (7) (EBR method Citation[33]), and Equation (8) (KT method Citation[34]):
where f, F, r G, and r O are the molar ratios of the comonomers in the feed and in the copolymer and reactivity ratios of GMA and ODA monomers, respectively. The G, H, k, η, and ξ as defined in Table stand for mathematical functions of f and F. The parameter α is an arbitrary denominator which produces more homogeneous distribution of data along the η–ξ axes (Figure ).
Table 3. Parameters of Equations (4)–(8) for linear GMA–ODA copolymers.
Figure 2 Plots of (a) Mayo–Lewis, (b) Fineman–Ross, (c) Inverted Fineman–Ross, and (d) Kelen–Tudos methods for solution copolymerization of GMA and ODA.
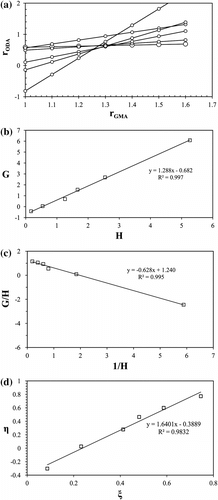
In this ML method for each experiment with different feed and copolymer compositions, r O values are plotted as a function of various assumed values of r G according to Equation (4). Each experiment yielded a straight line and the intersection of the lines gives the best values of r G and r O (Figure ). In FR method, G values are plotted against H values to yield a straight line with r G as its slope and −r O as its intercept (Equation (5)). The IFR plot (G/H vs. 1/H) gives −r O as the slope and r G as the intercept (Equation (6)). The FR and IFR plots are given in Figure , respectively.
The main criticism that can be put forward against the aforementioned methods is that r
G and r
O do not play equal roles in the equation of the copolymer composition (Equation (4)). The EBR method enhances some improvements in this regard, by entering k = F/f in Equation (5) and dividing it by to result in Equation (7) in which r
G and r
O play equal roles. Solving Equation (7) for all the tests it would result in determining r
G and r
O. The KT method also intends to prevent the nonsymmetrical character of the equation of copolymer composition (Equation (4)) by introducing new parameters such as η, ξ, and α (Equation (8)). Plotting the values of η vs. ξ provided a straight line that yielded −r
O/α and r
G as intercepts on extrapolation to ξ = 0 and ξ = 1, respectively (Figure ).
The parameters of Equations (4)–(8) for linear GMA–ODA are given in Table and the copolymerization reactivity ratios of GMA and ODA are listed in Table .
Table 4. Copolymerization parameters for solution copolymerization of GMA and ODA.
The regression coefficient (R 2) is a statistical measure of how well a regression line approximates the real data points. It can be calculated for each method using Equation (9) Citation[36,37].
According to Table , as expected, GMA showed higher reactivity ratio rather than ODA. This is because of higher reactivity of methacrylates toward acrylates, due to steric factors, polarities of monomers, and resonance stabilization of their radicals Citation[38,39]. On the other hand, flexibility and free movement of the butyl side group in ODA hinders the approach of incoming monomer to react the radical (shielding effect) Citation[38]. It is also mentioned in Table that r G × r O < 1, which means the copolymerization is apt to form random copolymers.
As well, the monomer relativity ratios were theoretically calculated based on revised patterns of reactivity scheme Citation[40] using Equation (10):
where r 12 is the reactivity ratio of monomer 1 in the copolymerization with monomer 2, r 1S is the general reactivity of the radical of monomer 1 polymerized with styrene, u 2 is the polarity of monomer 2, π 1 is the polarity of the radical of monomer 1, and υ 2 is the general reactivity of the monomer 2.
Calculated monomer reactivity ratios were reported in Table , which showed a considerable difference with experimental ones. Same conflict was also observed in the case of copolymerization of methyl methacrylate and ODA in benzene Citation[41]. The main reason for this deviation is attributed to different solubility of monomers in toluene, which has not been considered in revised patterns of reactivity scheme. ODA by means of its nonpolar long chain alkyl group has higher solubility in toluene in comparison to GMA containing polar epoxy group. Therefore, reactivity of GMA radicals is lower than theoretical value, while that for ODA radicals is higher.
Molar fraction of GMA in the copolymer (F G) as a function of GMA molar fraction in the feed (f G) is given in Figure . Data are calculated based on our reactivity ratios from FR method (r G = 1.29 and r O = 0.68). Figure presents not only the relationship between molar fraction of monomer in the feed and copolymer but also shows the changing molar fraction of comonomers in the copolymer during polymerization. According to Figure , the GMA molar fraction in the feed is decreased through polymerization period (increasing conversion) because of higher consumption rate of GMA. Molar fraction of GMA in the copolymer will also decrease by increasing conversion due to its decreasing content in the feed.
Characterization of crosslinked GMA–ODA copolymer
To study the potential of GMA–ODA copolymers for organic solvent absorbency application, crosslinked copolymers with different comonomer ratio were prepared (Scheme ).
All of crosslinked samples showed considerable yield of reaction (>94%) and significant gel content values (>81%, Table ). The chemical structure of crosslinked copolymers was also investigated using FTIR spectroscopy. The representative FTIR spectrum of C6 sample is shown in Figure . The peaks at 843 and 958 cm−1 belong to asymmetrical stretching vibrations of epoxy group. The peak of stretching vibration of C=O and C–O bonds of ester groups appeared at 1734 and 1166 cm−1, respectively. Absorption signals at 2849 and 2918 cm−1 were attributed to stretching vibration of C–H bonds of methyl and methylene groups. As well, the peaks of bending vibration C–H bonds appeared at 1468 and 1379 cm−1. The mentioned peaks not only confirmed the incorporation of both comonomers in the copolymer structure, but also indicated that the epoxy groups remained stable during the radical copolymerization.
Copolymerization often brings about changes in the glass transition temperatures (T g), which are one of the most important physical properties of a polymer. DSC thermograms for C1, C2, and C6 samples are shown in Figure . Samples showed a change in the base-line at temperature range of −35–−4 °C and an endothermic peak at temperatures of 48–55 °C were attributed to T g and melting of octadecyl groups (T m) of samples, respectively. According to Figure , enhancing the GMA content in the copolymers resulted in an increase for T g values. This was expected due to higher T g of GMA homopolymer (94 °C) in comparison to that of ODA homopolymer (−75 to −60 °C) Citation[26,42]. As well, melting points of copolymers were shifted to lower temperatures with increasing the GMA portion in the copolymers. This phenomenon is attributed to decrease in crystallinity of octadecyl groups via introducing of polar epoxy groups of GMA, which limits the close packing of alkyl chains Citation[27]. As previously mentioned, this decrease in crystallinity is favored for organic solvent absorbency applications Citation[27]. Meanwhile, ODA homopolymer (C1 sample) showed a thermal degradation with exothermic peak at 230 °C, while no degradation peak appeared for copolymers (C2 and C6 sample). This may be attributed to both lower thermostability of linear alkyl chain and the possibility of crosslinking of the GMA epoxy groups under thermal treatment higher than 200 °C.
Swelling behaviors
It is well known that polymeric networks based on hydrophobic monomers such as long chain alkyl (meth)acrylates possess high tendency for absorption of organic solvents and oil Citation[23,24,27]. For this respect, the swelling behaviors of the crosslinked ODA-GMA copolymers with different comonomer ratio were studied for nonpolar solvents (benzene, toluene, and crude oil) as well as a polar organic solvent (THF). The results of swelling test are illustrated in Figure . For nonpolar solvents, the swelling ratio of gels decreased with higher GMA content in the copolymers. This is due to the decrease of hydrophobicity of the crosslinked network and therefore lower van der Waals interaction with the nonpolar solvents. Meanwhile, all of the crosslinked copolymers showed higher affinity for absorption of toluene comparing to benzene and crude oil. For crude oil, due to higher viscosity, oil molecules cannot easily diffuse from the surface of the gels into the internal space of the networks. Similar observation has been previously reported by others Citation[24,27]. In the case of benzene, such difference probably is pointed out to higher nonpolarity of toluene than benzene and therefore superior physical interactions with the network structure.
Figure 6 Swelling characteristics of crosslinked copolymers in various solvents at room temperature.
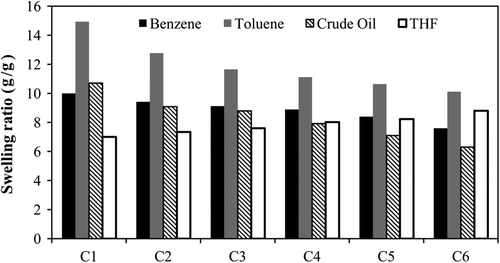
On the contrary, the THF absorption was improved using copolymers with higher GMA content. This is because of increasing of network polarity due to incorporation of polar epoxy groups and consequently higher interactions with THF molecules. Swelling data also reflected that in spite of using GMA as a polar monomer in the construction of the copolymers, their non-polar solvent absorbency was not lessened considerably and the prepared copolymer can be used as functional gels for oil absorption application. The epoxy groups have a potential for further modifications of the prepared polymers such as reaction with aromatic amines to improve the oil absorbency of gels or crosslinking via reaction with diamines.
Conclusion
In this research, linear and crosslinked GMA–ODA copolymers were synthesized via radical copolymerization in toluene solvent. The copolymer compositions were determined 1H NMR spectroscopy. The reactivity ratios computed with ML, FR, IFR, EBR, and KT methods showed higher activity for GMA monomer in comparison with ODA monomer (FR: r G = 1.29 and r O = 0.68). Incorporation of GMA in the crosslinked copolymers led to an increase in T g value opposite of Tm values. As expected, samples with higher GMA content showed higher swelling ratio in polar solvent and lower in nonpolar solvents. Meanwhile, copolymers had higher affinity for toluene in comparison to benzene and crude oil. Although incorporation of GMA in the crosslinked copolymers led to decreased absorbency in nonpolar organic solvents, however, the swelling values are so acceptable that the prepared functional copolymers can be used for nonpolar solvents absorption applications. As well, the epoxy groups can be used for further modifications of the prepared copolymers such as reaction with aromatic amines to improve the oil absorbency or crosslinking via reaction with diamines.
References
- Taylor , JW and Winnik , MA . 2004 . Functional latex and thermoset latex films . Journal of Coatings Technology and Research , 1 : 163 – 93 .
- Kawaguchi , H . 2000 . Functional polymer microspheres . Progress in Polymer Science , 25 : 1171 – 210 .
- Godwin , GG , Selvamalar , CSJ , Penlidis , A and Nanjundan , S . 2004 . Homopolymer of 4-propanoylphenyl methacrylate and its copolymers with glycidyl methacrylate: synthesis, characterization, reactivity ratios and application as adhesives . Reactive & Functional Polymers , 59 : 197 – 209 .
- Bakhshi , H , Zohuriaan-Mehr , MJ , Bouhendi , H and Kabiri , K . 2011 . Effect of functional monomer GMA on the physical-mechanical properties of coatings from poly(BA-MMA) latexes . Journal of Materials Science , 46 : 2771 – 7 .
- Selvamalar , CSJ , Vijayanand , PS , Penlidis , A and Nanjundan , S . 2004 . Homopolymer and copolymers of 4-benzyloxycarbonylphenyl acrylate with glycidyl methacrylate: synthesis, characterization, reactivity ratios, and application as adhesive for leather . Journal of Applied Polymer Science , 91 : 3604 – 3612 .
- Song , P , Zhang , Y and Kuang , J . 2007 . Preparation and characterization of hydrophobically modified polyacrylamide hydrogels by grafting glycidyl methacrylate . Journal of Materials Science , 42 : 2775 – 81 .
- Labella , R , Braden , M and Davy , KWM . 1992 . Novel acrylic resins for dental applications . Biomaterials , 13 : 937 – 43 .
- Guilherme , MR , Reis , AV , Takahashi , SH , Rubir , AF , Feitosa , JPA and Muniz , EC . 2005 . Synthesis of a novel superabsorbent hydrogel by copolymerization of acrylamide and cashew gum modified with glycidyl methacrylate . Carbohydrate Polymers , 61 : 464 – 71 .
- Horak , D , Benes , M , Gumargalieva , K and Zaikov , G . 2001 . A novel highly copper(II)-selective chelating ion exchanger based on poly(glycidyl methacrylate-co-ethylene dimethacrylate) beads modified with aspartic acid derivative . International Journal of Polymeric Materials , 50 : 85 – 91 .
- Monnereau , C , Blart , E , Fontaine , VLM and Odobel , F . 2005 . Synthesis of new crosslinkable co-polymers containing a push-pull zinc porphyrin for non-linear optical applications . Tetrahedron , 61 : 10113 – 21 .
- He , S , Wu , W , Wang , R , Pu , W and Chen , Y . 2011 . Investigation on the polyamide 6/SEBS-g-MA/organoclay nanocomposites with glycidyl methacrylate as a compatibilizer . Polymer – Plastics Technology and Engineering , 50 : 719 – 26 .
- Salmawi , KME and Ibrahim , SM . 2005 . Radiation grafting of 2-hydroxy ethyl methacrylate (HEMA)/glycidyl methacrylate (GMA) onto polyethylene-coated polypropylene non-woven fabric . Polymer – Plastics Technology and Engineering , 44 : 1671 – 87 .
- Horak , D . 2001 . Magnetic polyglycidylmethacrylate microspheres by dispersion polymerization . Journal of Polymer Science Part A: Polymer Chemistry , 39 : 3707 – 15 .
- Kalal , J , Svec , F and Marousek , V . 1974 . Reactions of epoxide groups of glycidyl methacrylate copolymers . Journal of Polymer Science Polymer Symposium , 47 : 155 – 66 .
- Safa , KD and Nasirtabrizi , MH . 2006 . Ring opening reactions of glycidyl methacrylate copolymers to introduce bulky organosilicon side chain substituents . Polymer Bulletin , 57 : 293 – 304 .
- Hradil , J , Svec , F and Frechet , JM . 1987 . Mechanism of phase-transfer catalysis using glycidyl methacrylate-ethylene dimethacrylate copolymers modified with tributylammonium groups in nucleophilic displacement reactions . Journal of Polymer , 28 : 1593 – 98 .
- Paul , S and Ranby , B . 1976 . Studies of methyl methacrylate-glycidyl methacrylate copolymers: copolymerization to low molecular weight and modification by ring-opening reaction of epoxy side groups. Journal of Polymer Science: Polymer Chemistry Edition , 14 : 2449 – 61 .
- Iwakura , Y , Kurosaki , T , Ariga , N and Ito , T . 1966 . Copolymerization of methyl methacrylate with glycidyl methacrylate and the reaction of the copolymer with amines . Die Makromolekulare Chemie , 97 : 128 – 38 .
- Alvares , D and Lucas , EF . 2000 . Chemical structure effect of (meth) acrylic ester copolymers and modified poly (ethylene-co-vinyl acetate) copolymers on paraffin deposition prevention in crude oil . Petrol Science Technology , 18 : 195 – 202 .
- Zhou , J , Wang , L , Dong , X , Yang , Q , Wang , J , Yu , H and Chen , X . 2007 . Preparation of organic/inorganic hybrid nanomaterials using aggregates of poly(stearyl methacrylate)-b-poly(3-(trimethoxysilyl) propyl methacrylate) as precursor . European Polymer Journal , 43 : 1736 – 43 .
- Li , P , Shen , Y , Li , X and Yang , X . 2011 . Preparation and application of amphoteric polyacrylate containing long hydrophobic aliphatic chain in the presence of poly(vinyl alcohol) . Polymer – Plastics Technology and Engineering , 50 : 29 – 35 .
- Harris , HV and Holder , SJ . 2006 . Octadecyl acrylate based block and random copolymers prepared by ATRP as comb-like stabilizers for colloidal micro-particle one-step synthesis in organic solvents . Polymer , 47 : 5701 – 6 .
- Dutertre , F , Pennarun , PY , Colombani , O and Nicol , E . 2011 . Straightforward synthesis of poly(lauryl acrylate)-b-poly(stearyl acrylate) diblock copolymers by ATRP . European Polymer Journal , 47 : 343 – 51 .
- Atta , AM , El-Ghazawy , RAM , Farag , RK and Abdel-Azim , A-AA . 2006 . Swelling and network parameters of oil sorbers based on alkyl acrylates and cinnamoyloxy ethyl methacrylate copolymers . Journal of Polymer Research , 13 : 257 – 66 .
- Hirabayashi , T , Kikuta , T , Kasabou , K and Yokota , K . 1988 . Main-chain flexibility and side-chain crystallization of widely spaced combe-like polymers . Polymer Journal , 20 : 693 – 8 .
- Jordan , EF . 1971 . Side-chain crystallinity. III. Influence of side-chain crystallinity on the glass transition temperatures of selected copolymers incorporating n-octadecyl acrylate or vinyl stearate . Journal of Polymer Science Part A: Polymer Chemistry , 9 : 3367 – 78 .
- Jang , J and Kim , B-S . 2000 . Studies of crosslinked styrene–alkyl acrylate copolymers for oil absorbency application. I. Synthesis and characterization . Journal of Applied Polymer Science , 77 : 903 – 13 .
- Polic , AL , Duever , TA and Penlidis , A . 1998 . Case studies and literature review on the estimation of copolymerization reactivity ratios . Journal of Polymer Science Part A: Polymer Chemistry , 36 : 813 – 22 .
- Bakhshi , H , Zohuriaan-Mehr , MJ , Bouhendi , H and Kabiri , K . 2009 . Spectral and chemical determination of copolymer composition of poly (butyl acrylate-co-glycidyl methacrylate) from emulsion polymerization . Polymer Testing , 28 : 730 – 6 .
- Cheng , HN and Lee , GH . 1985 . Two-dimensional NMR studies of polypropylene tacticity . Polymer Bulletin , 13 : 549
- Mayo , FR and Lewis , FM . 1944 . Copolymerization. I: a basis for comparing the behavior of monomers in copolymerization: the copolymerization of styrene and methyl methacrylate . Journal of the American Chemical Society , 66 : 1594 – 601 .
- Fineman , M and Ross , SD . 1950 . Linear method for determining monomer reactivity ratios in copolymerization . Journal of Polymer Science , 5 : 259 – 62 .
- Ezrielev , AL , Brokhina , EI and Roskin , YS . 1969 . Analytical method of evaluation of the copolymerization constants . Vysokomolekulyarnye Soedineniya, Seriya A , 11 : 1670 – 84 .
- Kelen , I and Tudos , F . 1975 . Analysis of the linear methods for determining copolymerization reactivity ratios. I: a new improved linear graphic method . Journal of Macromolecular Science, Part A , 9 : 1 – 27 .
- Bakhshi , H , Bouhendi , H , Zohuriaan-Mehr , MJ and Kabiri , K . 2010 . Semibatch emulsion copolymerization of butyl acrylate and glycidyl methacrylate: effect of operating variables . Journal of Applied Polymer Science , 117 : 2771 – 80 .
- Cohen , J , Cohen , P , West , SG and Aiken , LS . 2003 . Applied Multiple Regression/Correlation Analysis for the Behavioral Sciences , New Jersey : Lawrence Erlbaum Associates .
- Lee , H , Tae , G and Kim , YH . 2008 . A study on the copolymerization kinetics of phenylethyl acrylate and phenylethyl methacrylate . Macromolecular Research , 16 : 614 – 9 .
- Dhal , PK , Ramakrishna , MS and Babu , GN . 1982 . Copolymerization of glycidyl methacrylate with alkyl acrylate monomers. Journal of Polymer Science: Polymer Chemistry Edition , 20 : 1581 – 5 .
- Habibi , A , Vagheghani-Farahani , E , Semsarzadeh , MA and Sadeghiani , K . 2004 . Estimation of monomer reactivity ratios in free-radical solution copolymerization of lauryl methacrylate-isobutyl methacrylate . Journal of Polymer Science Part A: Polymer Chemistry , 42 : 112 – 29 .
- Jenkins , AD and Jenkins , J . 1997 . The revised patterns scheme for the prediction of monomer reactivity ratios. Part 4: a table of parameters and the r11 check . Polymer International , 44 : 391 – 6 .
- Jordan , EF , Bennett , R , Shuman , AC and Wrigley , AN . 1970 . Reactivity ratios and copolymerization parameters for copolymers incorporating n-octadecyl acrylate and N-n-octadecylacrylamide . Journal of Polymer Science Part A: Polymer Chemistry , 8 : 3113 – 21 .
- Freckmann DMM, Idem AT, Ellsworth MW. Tuning the mechanical properties of side chain crystallizable block copolymers. In: Theato P, Kilbinger AFM, Coughlin EB, editors. Non-conventional functional block copolymers, ACS Symposium Series, Vol. 1066; Washington, DC: American Chemical Society; 2011. p. 1–8