Abstract
Alternative copolymers of maleic anhydride (MA) and ethyl vinyl ether (EVE) [P(MA-alt-EVE)] were prepared in supercritical carbon dioxide (scCO2). The chemical structure of the obtained P(MA-alt-EVE)s was characterized by FT-IR and 13C NMR spectroscopy and showed that the copolymers possess strictly alternating structure. The influences of MA/EVE feed ratio, reaction time, and solvent as well as the initial initiator concentration on the copolymerization were also studied. It was indicated that the increase rate of reaction pressure could be related with good accuracy to monomer conversion, providing a simple and efficient method for monitoring the reaction processes. Gel Permeation Chromatography examination of the alternative copolymers revealed that the molecular weights of P(MA-alt-EVE) synthesized in scCO2 at different pressures were all higher than those prepared in organic solvents. Thermal properties were investigated by TGA and showed P(MA-alt-EVE) has good thermal stability (T d = 251 °C). Comparison studies on the P(MA-alt-EVE) in scCO2 and that prepared in organic solvent exhibit scCO2 solvent and it plays an important role in the copolymerization of MA and EVE.
1. Introduction
Alternating copolymers exhibit some unique properties such as superior thermal stabilities, fire resistance, photo-, X-ray, and E-beam sensitivities, catalytic and chiroptical activities, and extremely low band gap materials Citation[1,2]. Among these copolymers, alternating copolymers of maleic anhydride (MA) with alkyl vinyl ether (AVE) have received significant attention due to their unique properties and wide range of applications such as adhesives, photocrosslinkable and photosetting resin compositions, photographic films, electrophotographic recording and glass fiber coatings, and flocculants, as well as controlled-release coatings, medicinal tablet coatings, animal antidiarrhea capsules, and so forth Citation[3].
Currently, alternating copolymers of MA and AVE are synthesized industrially by two major processes: organic solution polymerization and bulk polymerization. In the first process, copolymerization occurs in organic solvents, such as butanone, dimethylbenzene, and cyclohexane. After the reaction is completed, the copolymer is collected and washed. Then it is dried to prevent remaining organic solvents. Unfortunately, the process generated large amounts of organic waste and required large quantities of energy to isolate the polymer in a dry form. Moreover, chain transfer reactions involving hydrogen atom of the solvent usually lead to low molecular weight copolymer. The bulk copolymerization of MA with AVE is a solvent-free alternative process to synthesize the alternating copolymer, where AVE was considered as solvent and comonomer. This synthetic route enjoys the advantage of avoiding organic waste. However, it was unadvantageous in controlling exothermic polymerizations. The resultant alternating copolymer is highly swollen with the unreacted AVE monomer. Even at a low solid content the reaction medium is practically a gel which cannot be efficiently agitated to keep the molecular weight distribution (MWD) under control. Then copolymerization has to be stopped. Therefore, an alternative method to prepare clean high molecular weight copolymer needs to be developed to avoid the use of toxic organic solvents and to reduce vast energy consumption in post-process.
Recently, the use of scCO2 as an alternative to traditional aqueous and organic solvents has attracted much attention in the fields of extraction, polymer synthesis, and material processing. CO2 is inexpensive, nontoxic, non-flammable, and readily available. Moreover, polymers can be isolated from the reaction mixture by simple depressurization, resulting in clean and dry products. Additionally, the charge-transfer complex (CTC) of MA and AVE, favoring alternating copolymerization, has been verified by many researchers Citation[4–6]. Low temperature, high pressure, and weak polarity can enhance the formation of the CTC of AVE and MA Citation[7]. Accordingly, the supercritical carbon dioxide (scCO2) is a preferred alternative for the alternating copolymerization of MA and AVE due to its nonpolarity, low dielectric constant, and unusually tunable versatile properties in solvent strength, viscosity, and so on.
Copolymerizations of MA and AVE in organic solvents have been mentioned in a few literatures Citation[8–10], however, there is no full article concerning on the copolymerization details in the novel scCO2 reaction medium. In this study, we have carefully investigated the copolymerization (Scheme ) of MA and EVE in scCO2 with 2,2-azoisobutyronitrile (AIBN) as the initiator in detail. The chemical structure of MA–EVE copolymer with FT-IR and 13C NMR, the thermal properties, and copolymerization behaviors are presented and discussed.
2 Experimental
2.1 Materials
MA was used after recrystallization in methanol and dried in the vacuum drying oven for 24 h. Ethyl vinyl ether (EVE) was purified through distillation. The 2,2-azobisisobutyronitrile (AIBN) was recrystallized twice from absolute methanol. Carbon dioxide ( > 99.9%) was further purified through columns of activatedalumina and a copper catalyst to remove water and oxygen. Butanone, cyclohexane, ethyl acetate, and other organic solvents were used without further purification.
2.2 Copolymerization of MA and EVE in scCO2
Polymerization was conducted in a 50 mL high pressure reactor equipped with a magnetic stirring bar, a thermocouple, and a rupture disk. The reactor was immersed in a water bath with a thermocouple connected to a temperature controller. In a typical experiment, EVE (0.02 mol), MA (0.02 mol), and AIBN (0.0102 g) were loaded to the reactor. The reactor was then sealed. Next, the reactor immersed in an ice bath was first purged with a flow of CO2 to remove air from the vessel and then filled with liquid CO2 to the desired amount (i.e. 30 g). Then the reactor was gradually heated to 70 °C to start the reaction (t = 20–30 min). When the reaction ended, the reactor was cooled, and CO2 was slowly vented. Then the reactor was opened to collect the polymer that usually was under the form of white powders mainly located at the bottom of the vessel. The collected powder was washed out by dissolving in acetone, precipitated in methanol, and then collected and dried under reduce pressure.
2.3 Characterization
The monomer conversion was determined gravimetrically as the ratio between the mass of resulting polymer and that of the feeded monomer. Chemical titration was utilized for determining the compositions of the obtained copolymers Citation[11]. Infrared spectra were obtained using a Bio-Rad FTS165 spectrometer. 13C NMR spectra were measured on Bruker Avance III-400 MHz instrument with acetone as solvent at room temperature. The molecular weight and its distribution of the copolymer was determined by Gel Permeation Chromatography (GPC) analysis relative to polystyrene calibration (Waters 515 HPLC pump, a Waters 2414 differential refractomerer, and two Waters Styragel columns (HR5E and HR4E)) using tetrahydrofuran (THF) as eluent at a flow rate of 1.0 ml/min at 25 °C. Differential scanning calorimetry (DSC) analysis was performed on a Perkin–Elmer Diamond DSC at a heating rate of 10 °C/min under nitrogen. Thermogravimetric analysis (TGA) was conducted with a Pyris Diamond TG/DTA (Perkin–Elmer Co., USA) with the heating rate 10 °C/min in a nitrogen atmosphere. The solubility was evaluated by charging approximately 5 mg polymer into 2 ml solvents, respectively, under stirring overnight at ambient temperature.
3 Results and discussion
3.1 Polymer characterization
Spectroscopic techniques were employed to characterize the structures of the polymeric products. All the copolymers give satisfactory analysis data corresponding to their expected structures. The typical FT-IR spectrum of P(MA-alt-EVE) is given in Figure . The sharp peaks at 1781 and 1863 cm−1 are attributed to symmetric and antisymmetric stretching vibration absorption peak of C=O group in MA units, respectively. The peak at 1225 cm−1 corresponds to the C–O–C stretching of EVE units. The symmetrical and asymmetrical stretching due to the methyl and methylene groups can be observed at 2982 and 2889 cm−1, respectively.
The formation of P(MA-alt-EVE) is also confirmed by 13C NMR spectrum giving their characteristic peaks as shown in Figure . The presence of signals at 10–20 and 33.3 ppm could be assigned to C8 and C1, respectively; the peaks of C4 in MA appeared to 38.35 ppm; the presence of signal at 50.14 ppm assigned to the presences of C3; and the presence of signals at 66.88 ppm assigned to C7. The presence of signals at 74.50 ppm could be attributed to C2 in vinyl ethers. The presence of signals between 170 and 180 ppm could be attributed C5 and C6 in MA. In the aliphatic carbons region, the three broad peaks between 30 and 60 ppm, related to the alternating triad (MA-EVE-MA) Citation[12] were observed. Figure shows the methylene subspectra of the EVE/MA copolymer. Only one broad methylene signal at 33–38 ppm of MA-EVE-MA is observed, demonstrating that the EVE/MA copolymers prepared in scCO2 possess the strictly alternating sequence structure Citation[13].
3.2 Polymerization behavior
3.2.1 Effect of MA/EVE feed ratio
Monomer feed ratios will greatly affect the monomer conversions and resulting copolymer compositions. To evaluate such an effect for the copolymerization of MA and EVE in scCO2, copolymerizations with different monomer feed ratios are conducted and the results are shown in Table . From Table it can be seen that the conversion of MA in Run 1 is only 3.4%, which shows that MA monomer is difficult to homopolymerize in scCO2 while MA and EVE monomer could copolymerize well. As the mole fraction of MA in feed ratio was 50%, as shown in Run 2, the conversion of MA was high (up to 88.3%) when the mole fraction MA in copolymer was 51.5 mol%. As the mole fraction MA in feed ration decrease from 33.3 to 16.7%, the conversions of MA were improved slowly from 89.1 to 92.7% while the mole fraction of MA in copolymer was almost unchanged at about 50 mol%.
Table 1. Copolymerization of MA/EVE in scCO2 with different monomer feed ratio.a
The copolymerization behavior of the comonomers can be better understood by the determination of reactivity ratios. The reactivity ratios of MA with EVE were determined by the application of Fineman-Ross (FR) linearization method from the monomer feed ratios and the copolymer compositions Citation[15–17]. According to the FR method the monomer reactivity ratios can be obtained by the equation:
With
where M 1 and M 2 are the monomer molar compositions in feed and m 1 and m 2 are the copolymer molar compositions.
The linear extrapolation plot using the FR method is depicted in Figure , and the reactivity ratios for MA (r MA) and EVE (r EVE) in scCO2 are 0.035 and 0.018, respectively. The low values of r MA, r EVE, and r MA·r EVE (r MA·r EVE <<1) show that, the studied system has a high tendency to form alternating copolymers. The calculated results of r MA and r EVE from FR method were similar with those (r MA = 0.0033 and r EVE=0.0025) from Q and e valves of MA and EVE Citation[14]. Furthermore, the reactivity ratios of MA and EVE in scCO2 was concordant with the 13C NMR result and show the copolymer of MA/EVE was an alternating copolymer.
3.2.2 Effect of reaction time
The relations of reaction time with monomer conversion are shown in Figure . At 70 °C, the conversion increased slowly at first 30 min and then increased quickly up to 90.3% after 10 h and then increased slowly to 93.3% to the end (16 h) of the polymerization. The change of conversion with time is well consist with the first-order decomposition of AIBN initiator or the change of the concentration of radical [C(CH3)2(CN)] in the early stage of the reaction. Namely, t 1/2 of AIBN at 70 °C is about 8 h, after 16 h. (2 × t1/2) over 75% of AIBN was consumed, and the continuation heating will never affect a significant increase of the conversion.
Figure 4 Effect of reaction time on the monomer conversion for the copolymerization of MA and EVE in scCO2.
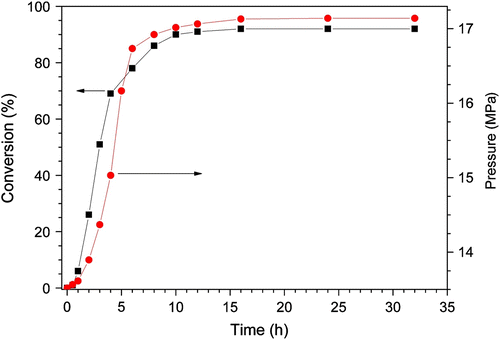
Figure also shows the relevance of the pressure in the polymerization system to reaction time and the result shows that the pressure increase could be related with good accuracy to monomer conversion. As shown in Figure , the pressure has almost no change at first 1 h and all the conversions are lower than 5 wt.%. While, the pressure increased quickly from 13.6 MPa at 2 h to 16.1 MPa at 6 h. The result could be tentatively explained considering phase change of the polymer and the monomers in the system under consideration. The variations of the pressure during the synthesis of macromolecules in scCO2 were studied by Beckman Citation[18] and Chen Citation[19] in the case of the polymerization of methyl methacrylate and acrylic acid, respectively. These authors have shown that the instantaneous pressure change can be related to the polymerization rate, the degree of compressibility of the polymerization mixture, the difference between molar volumes of the polymer and the monomer, and the variation of the volume change upon mixing terms for each phase. At preliminary stage, monomers and initiator are completely soluble in scCO2 while the copolymer P(MA-alt-EVE) with high molecular weight was hardly miscible and can be separated from the reaction system. On the one hand, the total volume would shrink when MA and EVE monomers are copolymerized to polymer. On the other hand, the separation of the copolymer from the solvent of scCO2 caused the phase disengagement in the polymerization system. Therefore, the total volume of two phases would be greater than the volume of the miscible phase containing monomers, initiator, and scCO2 solvent in the reaction reactor with fixed volume. Based on an overall consideration of the two factors, the expansion effect of phase disengagement would be greater than the shrinking effect in our experiments. When the reaction carried on after 8 h, the pressure was nearly stable at 17 MPa. Thats because the monomer concentration and the number of free radical were very low, reaction was almost finished at this time. So, the pressure of the system was stable. Consequently, the system showed the pressure increased with the increase of conversion and both curves have favorable relationship. The conversion of the copolymerization of MA and EVE in scCO2 can be tracked with the change of reaction pressure. For example, the degree of increase of reaction pressure can be used to predict the polymerization conversion. In addition, it can be deduced when the reaction pressure are steady after a period of pressure increase polymerization would essentially end. Hence, an efficient method was presented for monitoring the reaction processes.
3.2.3 Effect of initiator concentration
The polymerizations were conducted at four different concentrations of the initiator AIBN, as shown in Figure . When the polymerization was conducted at the lowest initiator concentration (0.0035 mol/L), there was a low monomer conversion (38%) at 4 h. However, at a higher initiator concentration (0.0076 mol/L), the monomer conversion reaches to 76% at the same polymerization time. This increasing trend of conversion is attributed to the creation of more numbers of reaction sites arising from an increase in the concentration of AIBN in the reaction system (50 ml). Further increasing the initiator concentration from 0.0076 to 0.0102 mol/L there was a slight increase in the polymer–monomer conversions ranging from 76 to 79%. These data indicate that adding much quantity of initiator does not accelerate the reaction further (i.e. there exists an optimal value of initial concentration that maximizes the polymerization rate).
3.2.4 Effect of reaction solvent
In the work, several organic solvents, such as butanone, dimethylbenzene, and cyclohexane are compared with scCO2 and are aimed to investigation of the solvent effects on the copolymerization of MA and EVE and the results are shown in Table .
Table 2. Copolymerizations of MA and EVE in different solvents.a
Morphology of polymer products varied with the polymerization solvents. Descriptions of the physical state based on the visual observation of the products immediately after the recovery of the polymers from the reactor are summarized in Table . The copolymerization of MA and EVE conducted in scCO2 solvent yielded white powers ( Run 1–3). When organic solvents replaced scCO2, glassy gel or viscous liquid products were obtained (Table Run 4–6), which would waste much energy if dry products are needed. So, scCO2 solvent is a favorable alternative for the preparation of dry and clean P(MA-alt-EVE) copolymers, eliminating the necessity for energy-intensive drying procedures often required in organic synthesis system.
The monomer conversions and the molecular weights for the copolymerization of MA and EVE in scCO2 are both higher than those in organic solvents. As can be seen from Table , the conversions for Run 1–3 in scCO2 ranged from 88.7–93.2% are all higher than those in organic solvents (Run 4–6 expect for Run 5, the conversions are all lower than 50%). In addition, the conversion for the copolymerization in scCO2 was improved from 88.7 to 93.2% when the pressure increased from 17 to 34 MPa. The molecular weights of P(MA-alt-EVE) synthesized in dimethylbenzene and butanone were lower than those synthesized in scCO2.
This could be attributed to higher plasticizing capability of CO2 to polymeric materials that enhances the small molecules permeated into even precipitation copolymer. According to the study of Kazarian Citation[20], specific intermolecular interactions between carbon dioxide and copolymers containing carbonyl groups enhance the capability of CO2 to swell and plasticize the copolymers. As a consequence of this intermolecular interactions, the free volume and segment mobility of the copolymers are increased in scCO2, which would lead to a higher diffusion rate of small molecules in the copolymer matrix and a lower viscosity for the reaction system Citation[21]. In the present study, the P(MA-alt-EVE) copolymer containing many carbonyl groups would have strong interaction with CO2. The effects of scCO2 solvent on the copolymerization of EVE and MA can be explained from four aspects. First, because of a depletion of electron density on the carbon atom, CO2 is a weak Lewis acid and interacts with oxygen atom of carbonyl groups having lone pair electron as Lewis base Citation[20]. The specific acid–base interaction can decrease electron density of MA and facilitate the formation of the CTC between EVE and MA. The CTC has a far greater reactivity toward the propagating radical compared to free monomer Citation[5]. Second, the intermolecular interactions between carbon dioxide and copolymers containing carbonyl groups enhance the capability of scCO2 to swell and plasticize EVE/MA alternative copolymers, which promote interphase transport of monomers Citation[12]. So, the chain propagation of P(MA-alt-EVE) is still high, although the polymerization locus shifts to the interior of polymer particles with the increase of polymer chains. Third, because chain entanglements restrict the mobilibity of the active polymer chains, the termination rate decrease. As a result, the molecular weight of the copolymer obtained in scCO2 is higher than the copolymer obtained in organic solvent. Finally, for the intermolecular interactions between scCO2 and EVE/MA copolymers, the CO2–MA interactions likely enhance the solubility of EVE/MA copolymer and restrain the copolymer precipitation, which result in the high molecular weight copolymer. Compared to copolymerization conducted in organic solvents, the copolymerization carried out in scCO2 would reduce the precipitation of the resulted copolymer and promote the chain propagation continuing the polymerization reaction, resulted in higher monomer conversion and molecular weight.
3.3 Thermal stability of P(MA-alt-EVE)
Most polymers are processed under high temperatures. It is thus important to investigate their thermal properties. We first examined the thermal stability of the copolymer by TGA under nitrogen (Figure ). Temperature for 5 wt.% loss has often been used as degradation temperature (T d) to estimate thermal stability of a synthetic polymer. As shown in Figure , P(MA-alt-EVE) exhibited good thermal stability has the T d value at 251 °C, which endow the copolymer with enough thermal stability at post processes. It is also seen from Figure , the weight loss of P(MA-alt-EVE) accelerated greatly after T d and the weight decreased quickly down to 30 wt.% at 479 °C. It is understandable that acid anhydride and ether groups in P(MA-alt-EVE) increase the speed of copolymer decomposition at high temperature. Finally, the rate of weight loss decelerated after 479 °C, and then the weight of P(MA-alt-EVE) sample decreased down to 21.8 wt.% to the end (800 °C) of the TGA analysis. It is possible, during the decomposition of the copolymer, that a cross-linked structure would be formed that yielded a thermal-stable char residue of about 20% weight for the original P(MA-alt-EVE).
We further investigated the transition behaviors of Poly(MA-co-EVE) through DSC measurement under the N2 atmosphere at a heating rate of 10 °C/min from 30 to 240 °C. The DSC curve is shown in Figure . It can be seen that the glass transition temperature (T g) of P(MA-alt-EVE) synthesized in scCO2 (T g=144 °C) was higher than that for the copolymer synthesized in DMB (T g=131 °C). The difference would be related with the higher molecular weight of P(MA-alt-EVE) synthesized in scCO2 (M n=4.36 × 10−4 g/mol) than that synthesized in dimethylbenzene (M n = 0.53 × 10−4 g/mol).
3.4 Solubility
The solubility of P(MA-alt-EVE) in various solvents was carefully evaluated and summarized in Table . It was found that P(MA-alt-EVE) are soluble in such polar solvents as acetone, dimethylformamide, and dimethyl sulfoxide, which were consistent with the fact that all these solvents and P(MA-alt-EVE) contained carbonyl group. In addition, although P(MA-alt-EVE) did not dissolve in most low-polarity solvents, such as cyclohexane, dimethylbenzene, and ether, it can well dissolve in THF, mainly due to their similar ring-like structure according to the principle of ‘similarity and intermiscibility’.
Table 3. Solubility of Poly(MA-co-EVE) in various liquids. Key: (−) insoluble, (+) soluble.
3.5 The copolymerization mechanism analysis of P(MA-alt-EVE)
According to the research of Kazarain Citation[20], CO2 is a nonpolar molecule with low dielectric constant, which has the properties of Lewis acid and Lewis base. Especially when CO2 is together with carbonyl, the carbon atom of CO2, which is lack of electron, interacts with the carbon atom from carbonyl and generates Lewis acid–base. The copolymer of EVE and MA has many anhydride groups, so there are strong interactions between the copolymer and CO2, the interaction will make CO2 swelling and plastifying the copolymer. CO2 also has interaction with MA, it cause the formation of CTC model which is propitious to the chain growth. According to Qiu Citation[22], when CO2 interacts with the carbonyl oxygen of anhydride groups, CO2 tends to close to MA at the side and was away from the alkyl. The steric hindrance of CTC(EVE-MA) helping to the living polymer chains attacks MA of CTC(EVE-MA) from the side of alkyl. It results that there are two configurerations MA in the copolymer (there are two integral areas at about 50 ppm in Figure . 13C NMR spectrums of P(MA-alt-EVE) prepared in scCO2) Citation[22]. The mechanism of the copolymerization and CTC model of EVE and MA is presented in Figure .
4 Conclusion
In this paper, we have successfully synthesized the alternating copolymer of MA and EVE in scCO2 and the copolymer was obtained in high yield as dry, clear, and white powders directly from the reaction vessel. The alternating structure of obtained P(MA-alt-EVE) was clearly confirmed by FT-IR and 13C NMR. It was demonstrated that P(MA-alt-EVE) main chain units have two configurations and the potential copolymerization mechanism was proposed. GPC examination of the alternative copolymers revealed that the molecular weights of P(MA-alt-EVE) synthesized in scCO2 at different pressures were all higher than those prepared in organic solvents. The increase rate of reaction pressure was related with good accuracy to monomer conversion, providing a simple and efficient method for monitoring the reaction processes. Comparison studies on the P(MA-alt-EVE) synthesized in scCO2 and that prepared in organic solvent exhibit scCO2 solvent and it plays an important role in the copolymerization of MA and EVE.
Acknowledgments
We are grateful to the financial support by Shandong Provincial Natural Science Foundation of China (No. ZR2009FM057) and National Natural Science Foundation of China (No. 20774073).
References
- Hill , DJT , O’Donnell , JH and O’Sullivan , PW . 1982 . Analysis of the mechanism of copolymerization of styrene and acrylonitrile . Macromolecules. , 15 : 960 – 966 .
- Ng , SC , Lu , HF , Chan , HSO , Fuji , A , Laga , T and Yoshino , K . 2000 . Analysis of the mechanism of copolymerization of styrene and acrylonitrile . Adv. Mater. , 12 : 1122 – 1125 .
- Zhao , LJ . 2004 . Study on the synthesis of copolymer of methyl vinyl ether/maleic anhydride, chemistry world . Chem. World. , 6 : 299 – 301 .
- Barb , WGJ . 1953 . Effect of nonterminal monomer units on the reactivity of polymeric free radicals . J. Polym. Sci. , 11 : 117 – 126 .
- Hill , DJT , O’Donnell , JH and O’Sullivan , PW . 1985 . Analysis of the mechanism of copolymerization of styrene and maleic anhydride . Macromolecules. , 18 : 9 – 17 .
- Dubin , P . 1967 . Hydrophobic hypercoiling in copolymers of maleic acid and alkyl vinyl ethers . J. Phys. Chem. , 71 : 2757 – 2759 .
- DeSimone , JM . 2002 . Practical approaches to green solvents . Science. , 297 : 799 – 803 .
- Kramer , MC , Stegar , JR and McCormick , CL . 1995 . Water-soluble copolymers: 66. Phase transfer studies of structural and environmental effects on domain organization in aqueous solutions of hydrophobically modified poly(sodium maleate-alt-ethyl vinyl ether)s . Polymer. , 37 : 4539 – 4546 .
- Dubin , PJ . 1970 . Hydrophobic bonding in alternating copolymers of maleic acid and alkyl vinyl ethers . J. Phys. Chem. , 74 : 2842 – 2847 .
- Wang , JF , Tian , J and Ye , P . 2010 . Synthesis and characterization of new optically active poly(amide-imide)s based on N,N′-(pyromellitoyl)-bis-L-amino acids and 1,3,4-oxadiazole moieties . Des. Monomers Polym. , 13 : 207 – 220 .
- Sclavonsa , M , Franquineta , P , Carliera , V , Verfailliea , G , Fallaisa , I , Legrasa , R , Laurentb , M and Thyrionb , FC . 2000 . Quantification of the maleic anhydride grafted onto polypropylene by chemical and viscosimetric titrations, and FT-IR spectroscopy . Polymer. , 41 : 1989 – 1999 .
- Ha , NTH . 1999 . Determination of triad sequence distribution of copolymers of maleic anhydride and its derivates with donor monomers by 13C NMR spectroscopy . Polymer. , 40 : 1081 – 1086 .
- Montaudo , MS . 2001 . Determination of the compositional distribution and compositional drift in styrene/maleic anhydride copolymers . Macromolecules. , 34 : 2792 – 2797 .
- Xu , T . 2011 . Cross-linking polymerization of N-isopropylacrylamide and diethylene glycol-dimethacrylate in supercritical carbon dioxide . Mater. Sci. Eng. , 8 : 27 – 30 .
- Finemann , M and Ross , SD . 1950 . Linear method for determining monomer reactivity ratios in copolymerization . J. Polym. Sci. , 5 : 259 – 262 .
- Kelen , T and Tüdös , F . 1975 . Analysis of the linear methods for determining copolymerization reactivity ratios. I. A new improved linear graphic method . J. Macromol. Sci. Chem. , A9 : 1 – 27 .
- Tüdös , F , Kelen , T and Turcsanyi , B . 1976 . Analysis of linear methods for determining copolymerization reactivity ratios. III. Linear graphic method for evaluating data obtained at high conversion levels . J. Macromol. Sci. Chem. , A10 : 1513 – 1540 .
- Chaudhary , AK , Beckman , EJ and Russell , AJ . 1995 . Rational control of polymer molecular weight and dispersity during enzyme-catalyzed polyester synthesis in supercritical fluids . J. Am. Chem. Soc. , 117 : 3728 – 3733 .
- Chen . 2003 . Syntheses and properties of novel high performance series poly(aromatic ethers) polymers containing phthalazinone moieties . Acta Polym. Sinica. , 4 : 6 – 14 .
- Kazarian , SG , Vincent , MF , Bright , FV , Liotta , CL and Eckert , CA . 1996 . Intermolecular interaction of carbon dioxide with polymers . J. Am. Chem. Soc. , 118 : 1729 – 1736 .
- Baruah , SD and Laskar , NC . 1996 . Styrene-maleic anhydride copolymers: synthesis, characterization, and thermal properties . J. Appl. Polym. Sci. , 60 : 649 – 656 .
- Qiu , GG . 2006 . Synthesis of ultrahigh molecular weight poly(styrene-alt-maleic anhydride) in supercritical carbon dioxide . Macromolecules. , 39 : 3231 – 3237 .