Abstract
In this work, the spiroorthocarbonate FSOC derived from fluorene was prepared. After characterization by FTIR and NMR spectroscopy, the synthesized FSOC was evaluated as antishrinkage additive in the cationic photopolymerization of bisglycidil ether of Bisphenol-A (DGEBA). The FSOC was mixed at 2.5–10 mol% with DGEBA. It was found that shrinkage decreased with increased concentration of FSOC. At 10 mol% of FSOC not only shrinkage was eliminated, but a small level of volume expansion was also achieved. The presence of FSOC did not interfere with the photopolymerization of DGEBA.
1. Introduction
Shrinkage is a phenomenon inherent to all types of polymerization. It is defined as the reduction in volume caused by an increase in density, by passing from the liquid or viscous state of the monomer to the solid state of the final polymer. Shrinkage can be an adverse factor in certain applications, such as the development of composite materials, materials for electrical insulation, manufacture of optical fiber, and the preparation of dental polymeric materials. Besides, in certain applications such as stereolithography, coatings, adhesives, composites, and sealants, the shrinkage can become a serious problem, because the stress induced by the shrinkage can originate the loss of dimensional stability as well as the formation of microvoids, microcracks, and delamination reducing the performance and life time of the products.
Epoxy resins used in coatings, adhesives, and in dental materials generally shrink 4–6% in volume Citation[1], but even this low level of shrinkage can reduce the adhesion to substrates as a result of volumetric and dimensional changes. For these reasons, the suppression or reduction of the volume shrinkage is of great importance in the design of materials requiring precise dimensions or for the preparation of composites.
Spiroorthocarbonates (SOCs) have demonstrated to be effective volume-control additives reducing the level of shrinking of different polymeric materials. SOCs can reduce the shrinking by effect of the double ring opening polymerization generating poly(ether-carbonates) whose flexible nature and volume expanding ability reduce the internal stress in the resulting polymers Citation[2,3]. Bailey and collaborators pioneered the studies on the usage of SOCs as expanding monomers Citation[4–6]. Since then, numerous reports on the synthesis of new SOCs have appeared Citation[7–11], and employed as shrinkage reduction additives in dioxirane polymerization by using boron trifluoride as catalyst and elevated temperature Citation[12] as well as in cationic photopolymerization of an aromatic dioxirane Citation[13]. Also, previous studies from our group have demonstrated the effectiveness of epoxy and oxetane functionalized SOC Citation[14,15] as low shrinkable agents in cationic UV curing of epoxy and oxetane photocurable formulations.
Aromatic SOCs have the advantage of being more stable to hydrolysis in comparison with the aliphatic ones. It was found that while an aliphatic SOC could resist 24 h in humid conditions, an aromatic SOC could stand the same conditions more than a month before undergoing complete hydrolysis Citation[16].
Taking into consideration the efficiency demonstrated by SOCs to reduce or eliminate shrinkage, in this communication it is reported the preparation of a SOC derived from an aromatic compound such as the 9H-fluorene-9, 9-dimethanol (FDiOH). The desired compound was synthesized by means of a transesterification reaction between tetraethyl orthocarbonate (TEOC) and FDiOH. Once that the fluorene spiroorthocarbonate (FSOC) was synthesized, its activity as antishrinkage additive in the cationic photopolymerization of bis-glycidil ether of Bisphenol-A (DGEBA) was studied.
2 Experimental
2.1 Materials
FDiOH, TEOC, p-toluene sulfonic acid (p-TsOH) were purchased from Aldrich Co. Bisphenol-A-based epoxy resin (Araldite® GY250, weight per epoxide 183–189 g/eq, Huntsman, DGEBA) was used as epoxy matrix. (4-n-decyloxy phenyl) phenyliodonium hexafluoroantimonate (DPPI) was prepared according to a method reported elsewhere Citation[17].
2.2 Synthesis of the 3, 9-Di (9-fluorenylidenyl)-1, 5, 7, 11-tetraoxa-5, 5-undecane (FSOC)
In a three-necked, 50-mL, round-bottom flask provided with magnetic stirrer, condenser, nitrogen inlet, and thermometer, were put in 2 g of molecular sieves and 40 mL of diethyl ether. Then 4 g (1.76 × 10−2 mol) of FDiOH and 4.87 × 10−2 g (2.8 × 10−4 mol) of p-TsOH were also added. After 5 min were added 1.47 g (7.66 × 10−3 mol) of TEOC and 8.93 × 10−2 g (8.42 × 10−3 mol) of Na2CO3. The system was flushed with nitrogen to set anhydrous conditions, and the reaction was left for 9 h at room temperature. After the reaction time, the reaction mixture was filtered and the solvent was rotoevaporated obtaining a white solid with a melting point of 261–263 °C. The yield of the reaction was 93.5%.
1H NMR (500 MHz, CDCl3, δ ppm): 7.80 (dd, 8H, aromatic protons), 7.45 (dt, 8H aromatic protons), 4.35 (s, 8H, –CH2–O–). 13C NMR: (125 MHz, CDCl3, δ ppm): 48.2, 70.4, 114.5, 122.3, 126.3, 128.6, 129.2, 142.4, 146.3.
2.3 Sample preparation
Photocurable formulations were prepared by the addition of the FSOC monomer in the range between 2.5 and 10 mol% to the epoxy monomer DGEBA, which was used as base monomer. DPPI was added at 1 mol% to all photocurable formulations, as cationic photoinitiator.
2.4 Determination of kinetics of photopolymerization by real-time FTIR spectroscopy
The progress of the photopolymerization process was followed by real time FTIR (RT-FTIR) in a Magna Nicolet 550 spectrometer. During kinetics, the spectra were obtained co-adding seven individual scans at 8 cm−1 resolution. About 25 μm-thickness films were coated on silicon wafers and simultaneously exposed to the UV beam (UVEX portable UV lamp) with an intensity of 10 mW/cm2 to induce polymerization, and to the IR beam, to make an in situ evaluation of the extent of reaction. The conversion of the epoxy group was followed by monitoring the decrease in the absorbance of the epoxy groups centered at 918 cm−1.
2.5 Analysis of gel content
Gel content values of the cured coatings were obtained by measuring weight loss after 24 h in chloroform at room temperature (ASTM D2765-84).
2.6 Analysis of polymer by dynamic mechanical analysis
The viscoelastic properties were determined using dynamic mechanical analysis instrument Rheometric Scientific Dynamic Mechanical Analyzer at a frequency of 1 Hz. The photocurable formulations were placed in plastic mold (which dimensions were 10 × 40 mm with a thickness of 2 mm), and cured in a UV oven provided with a Fusion 300S lamp. This lamp has emission spectrum with peaks at 254, 313, 365, and 420 nm. After 20 min in the UV oven the samples were subjected to a postthermal curing at 120 °C by an hour to ensure complete curing.
2.7 Determination of reduction of shrinkage
Volume changes of the obtained polymers were determined by measuring the density of the formulations and that of the polymers. The formulation density before curing was measured by weighing a precise amount of liquid formulation and measuring accurately the volume. The same rectangular specimens prepared for DMA analysis were used for these measurements. The density of polymers was measured by means of a Sartorius balance equipped with an YDK01density determination kit. The Archimedes principle was applied for determining the specific gravity of the solid with this measuring device: a solid immersed in a liquid is exposed to the force of buoyancy. The value of this force is the same as that of the weight of the liquid displaced by the volume of the solid. The density measurements were performed with the Sartorius hydrostatic balance which enables to weigh the solid in air as well as in a liquid, the specific gravity of the solid is determined when the density of the liquid causing buoyancy is known, through the following formula:
where ρ = specific gravity of the solid: ρ(l) = density of the liquid; W(a) = weight of the solid in air; W(l) = weight of the solid in liquid.
The chosen liquid was distilled water with a density value of 0.9976 g/ml at 23 °C, which was the temperature used to perform the analysis. The specific volume of the system before and after curing was defined as the inverse of the density and the shrinkage was calculated with the following formula:
where ΔV = volume change; V s = specific volume of the cured polymer; V L = specific volume of the liquid formulation.
3 Results and discussion
3.1 Synthesis of FSOC
The aim of this work was to prepare a bulky aromatic SOC that could have better efficiency as antishrinking agent than other aliphatic SOCs that we have already reported Citation[18,19]. After reviewing the literature, it was decided to prepare the SOC derived from available starting material FDiOH. Though the synthesis of FSOC was previously reported Citation[20], in that paper, this compound was prepared by a different synthetic method, using FDiOH and n-butyl tin oxide to obtain a cyclic intermediate that reacted in a second stage with CS2, obtaining the FSOC with 78% yield. The properties of this compound as antishrinkage agent in cationic photopolymerizations were not studied, and the paper focused only on its crystallographic properties. In our case, we are reporting a facile one-pot synthesis of the FSOC. The compound was achieved at 93.5% yield, by the reaction of FDiOH with TEOC by means of a transesterification reaction in the presence of p-TsOH as shown in Scheme . The proton spectrum of FSOC showed peaks at 7–8 ppm corresponding to the protons of the aromatic moiety, whereas a singlet peak at 4.3 ppm, corresponds to the protons of the carbons adjacent to the oxygens of the spiroorthocarbonate moiety.
Figure displays the IR spectra of FDiOH and FSOC. It can be seen that the absorption band that corresponds to the hydroxyl groups of FDiOH at 3318 cm−1, completely disappeared in FSOC when the transesterification reaction was carried out. It is also shown the presence of the peaks characteristics for spiroorthocarbonate groups located at 1196, 1113, and 1041 cm−1. UV spectrum of FSOC was also determined. Figure shows that the absorption of FSOC in the UV range is narrow with a maximum at 280 nm.
3.2 Evaluation of photocuring process by RT-FTIR
In Figure are reported the conversion curves as a function of irradiation time for the pristine DGEBA resin and for the photocurable formulations containing increasing FSOC content.
Figure 3 Real-time conversion curves as a function of irradiation time for the pristine DGEBA epoxy monomer and for the formulations containing increasing amount of FSOC monomer. DPPI at 1 mol% was used as photoinitiator for all formulations. The intensity of the UV light was 10 mW/cm2.
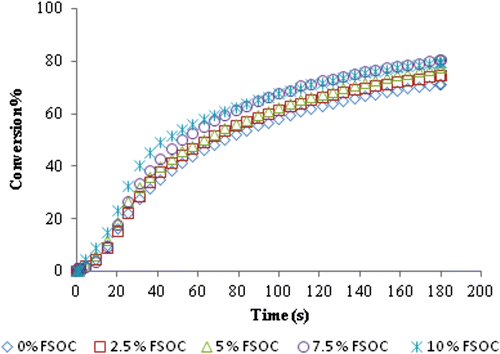
The DGEBA resin shows a quite high reactivity toward cationic UV curing, reaching a final epoxy group conversion of about 70% after 3 min of irradiation. The epoxy group consumption did not proceed further because of a vitrification effect: a highly glassy polymer network is formed during curing and a large number of unreacted epoxy groups remain trapped in the polymer network.
By adding FSOC monomer in the photocurable formulations, the rate of polymerization is not very much affected up to a content of 10 mol%; nonetheless, it can be observed a slight increase in the epoxy group conversion with increasing concentration of FSOC (see Table ). This behavior can be explained by the flexibility of the poly (ether-carbonate) chains formed after cationic induced double ring opening of FSOC, delaying the vitrification of the polymer and allowing in this way higher mobility of the reacting species, which finally results in slightly higher conversions. Thus, the presence of FSOC had a positive effect, as conversion increased when the concentration of FSOC was also increased above 5 mol%. In this process is assumed that both FSOC and DGEBA can copolymerize. In other work Citation[13] the cationic photopolymerization of a spiroorthocarbonate with an aromatic dioxirane was demonstrated to give rise to a copolymerization reaction with the insertion of the polycarbonate units in the polymeric backbone. A schematic representation of the copolymerization of FSOC and BADGE is shown in Scheme . In Figure are shown the IR spectra of the formulation of DGEBA with 10 mol% of FSOC, prior to irradiation and that of the polymer after 3 min of irradiation. It can be seen clearly the diminution of the band at 918 cm−1 corresponding to the epoxy group, as well as the increase of the band at 1126 cm−1 characteristic for the C–O absorption of the formed polyether. After UV irradiation, is obvious the formation of a band at 1748 cm−1 that belongs to the carbonyl group of the polyether carbonate formed, confirming in this way the cationic ring opening polymerization of FSOC. All the UV-cured films showed high gel content values (see Table ) indicating the absence of extractable monomer or oligomer, therefore in agreement with the copolymerization hypothesis between the FSOC and the DGEBA resin.
Table 1. Properties of UV-cured films.
3.3 Determination of viscoelastic properties of the obtained polyethers formulated with FSOC
Bulk samples were prepared and analyzed by means of DMA. Figure shows the curves of storage modulus vs temperature of the different samples studied. It can be seen that the addition of 2.5–10 mol% of FSOC to DGEBA did not affect significantly the mechanical properties of the cured polymer; there was only a slight decrease in the elastic modulus passing from 1990 MPa for pristine DGEBA to 1775 MPa for the polymer with 10% FSOC. In all cases the profiles of decay of elastic modulus were very similar. It is worth mentioning that the obtained polymers where FSOC were added, acquired a brown color.
Figure 5 Storage modulus obtained by DMA analysis for cured pristine DGEBA system and for DGEBA systems containing increasing content of FSOC.
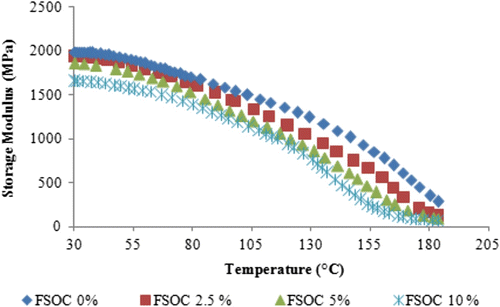
Figure shows the tan δ curves of the bulk samples. Tan δ curves show a maximum which is assumed as the T g of the cured films. In our case, it is possible to observe a shift of maximum of tan δ curves towards lower values by increasing the amount of FSOC in the photocurable formulation (see also Table ). The presence of a single tan δ peak is also an evidence of the formation of a uniform network. These data are in agreement with the RT-FTIR data previously discussed. The FSOC copolymerization with the epoxy resin induces a flexibilization of the network when FSOC is above 5 mol%. This flexibilization is responsible for the delay of vitrification which allowed reaching a higher epoxy group conversion upon curing.
3.4 Determination of shrinkage produced during the photopolymerization of DGEBA
The efficiency of the FSOC as low shrinkage additive was evaluated by adding increasing concentrations of this compound, separately into the monomer DGEBA and measuring the specific density of the starting formulations and those of the corresponding cured polymers. The volume changes values are reported in Table (negative values imply shrinkage, while positive values indicate expansion).
Table 2. Densities, specific volumes, and volume changes during UV curing for all samples studied.
An important decrease on the shrinkage extent upon photopolymerization was evidenced in the cured polymers obtained in the presence of FSOC monomer. It can be observed that pristine DGEBA displayed a shrinkage value of −4.77, while the polymer with only 2.5% of FSOC evidenced a 50% decrease of the shrinkage. In the case of the polymer with 5%, the reduction of the shrinkage was 60% while at 10 mol% of FSOC, it was observed a positive value of 0.19 which indicates that not only the shrinkage was suppressed but a slight level of expansion was achieved. This is notorious considering the relatively low concentration of FSOC needed. This behavior can be explained considering the formation of the poly (ether-carbonate) which contains the highly voluminous fluorene groups which induce large free volume in the polymeric matrix.
4 Conclusion
FSOC was synthesized and obtained at 93% yield, with the aim to reduce shrinkage during cationic photopolymerization of the epoxy monomer DGEBA. It was demonstrated that the presence of FSOC has a beneficial effect on the cationic photopolymerization of the epoxy monomer, increasing slightly the final epoxy group conversion. The observed increase was attributed to a delay of vitrification due to the insertion of poly (ether-carbonate) flexible macromolecules, into the cured network because of DGEBA–FSOC copolymerization. This was confirmed by the very high gel content values as well as by DMTA analyses, which showed the presence of only one tan δ peak for all the cured materials. Besides, the analysis of volume change revealed that FSOC not only induced the elimination of the shrinkage of the formed polyether, but it was achieved a small level of expansion (0.19%) when 10 mol% of FSOC was used.
Acknowledgments
The authors would like to thank the Mexican National Council and Technology (CONACYT) for founding this research (project 80108). Assistance in running IR, DSC, TGA-DSC-MS, and NMR samples by Julieta Sanchez, Silvia Torres, Guadalupe Mendez, María de Lourdes Guillen, and Guadalupe Tellez, is gratefully acknowledged.
References
- May , IA . 1988 . Epoxy resins , New York , NY : Marcel Dekker .
- Takata , T and Endo , T . 1993 . Recent advances in the development of expanding monomers: synthesis, polymerization and volume change . Prog. Polym. Sci. , 18 : 839 – 870 .
- Penco , M , Donetti , R , Mendichi , R and Ferruti , P . 1998 . New poly(ester-carbonate) multi-block copolymers based on poly(lactic-glycolic acid) and poly(ϵ-caprolactone) segments . Macromol. Chem. Phys. , 199 : 1737 – 1745 .
- Bailey , WJ , Iwama , H and Tsushima , R . 1976 . Synthesis of elastomers by cationic polymerization with expansion in volume . J. Polym. Sci. , 56 : 117 – 127 .
- Bailey , WJ and Endo , T . 1978 . Radical ring-opening polymerization and copolymerization with expansion in volume . J. Polym. Sci. , 64 : 17 – 26 .
- Bailey WJ, Polycyclic ring-opened polymers. United States patent US 4,387,215. 1983.
- Ge , J , Trujillo-Lemon , M and Stansbury , JW . 2006 . A mechanistic and kinetic study of the photoinitiated cationic double ring-opening polymerization of 2-methylene-7-phenyl-1,4,6,9-tetraoxa-spiro[4.4]nonane . Macromolecules. , 39 : 8968 – 8976 .
- Nagai , D , Nishida , M , Nagasawa , T , Ochiai , B , Miyazaki , K and Endo , T . 2006 . Non-shrinking networked materials from the cross-linking copolymerization of spiroorthocarbonate with bifunctional oxetane . Macromol. Rapid Commun. , 27 : 921 – 925 .
- Sonmez , HB and Wudl , F . 2003 . Synthesis of polymers based on spiroorthocarbonates . Polym. Preprints. , 44 : 803
- Guo YM, Zou YF, Pan CY. Investigation on cationic ring-opening polymerization of 1,5,7,11-tetraoxaspiro [5,5] undecane in the presence of low molecular weight tetraols. Polymer. 2001;42:1337–1344.
- Eick , JD , Smith , RE , Pinzino , CS , Kotha , SP , Kostoritz , EL and Chapelow , CC . 2005 . Photopolymerization of developmental monomers for dental cationically initiated matrix resins . Dent. Mater. , 21 : 384 – 390 .
- Zhou , Z , Jin , B and He , P . 2002 . Copolymerization behavior and kinetics of an epoxy copolymer with zero shrinkage . J. Appl. Polym. Sci. , 84 : 1457 – 1464 .
- Smith , RE , Pinzino , CS , Chappelow , CC , Holden , AJ , Kostoryz , EC , Guthrie , JR , Yourtee , DM and Eick , JD . 2004 . Photopolymerization of an expanding monomer with an aromatic dioxirane . J. Appl. Polym. Sci. , 92 : 62 – 71 .
- Sangermano , M , Acosta Ortiz , R , Puente Urbina , BA , Berlanga Duarte , ML , Garcia Valdez , AE and Guerrero Santos , R . 2008 . Synthesis of an epoxy functionalized spiroorthocarbonate used as low shrinkage additive in cationic UV curing of an epoxy resin . Eur. Polym. J. , 44 : 1046 – 1052 .
- Sangermano , M , Gianelli , S , Acosta Ortiz , R , Berlanga Duarte , ML , Rueda Gonzalez , AK and Garcia Valdez , AE . 2009 . Synthesis of an oxetane-functionalized hemispiroorthocarbonate used as a low-shrinkage additive in the cationic ultraviolet curing of oxetane monomers . J. Appl. Polym. Sci. , 112 : 1780 – 1787 .
- Endo , T , Sato , H and Takata , T . 1987 . Synthesis and cationic polymerization of 3,9-dibenzyl-1,5,7,11-tetraoxaspiro[5.5]undecane . Macromolecules. , 20 : 1416 – 1419 .
- Crivello , JV and Lee , JL . 1989 . Alkoxy-substituted diaryliodonium salt cationic photoinitiators . J. Polym. Sci., Part A: Polym. Chem. , 27 : 3951 – 3968 .
- Acosta Ortiz R, Berlanga Duarte ML, Savage Gomez AG Sangermano M, Garcia Valdez AE Novel diol spiro orthocarbonates derived from glycerol as anti-shrinkage additives for the cationic photopolymerization of epoxy monomers Polym. Int. 2010;59:680–685.
- Acosta Ortiz , R , Savage Gomez , AG , Berlanga Duarte , ML , Sangermano , M and Garcia Valdez , AE . 2012 . The effect of hydroxyspiro-orthocarbonates on the cationic photopolymerization of an epoxy resin and on the mechanical properties of the final polymer . Polym. Int. , 61 : 587 – 595 .
- Park YJ, No KH, Kim JH, Suh IH. Synthesis and X-ray crystallographic characterization of spiro orthocarbonates. Bull. Korean Chem. Soc. 1992;13:375–381.