Abstract
A new monomer, (E)-4-acetylpyridine oxime (APO), was prepared by reaction of 4-acetylpyridine and hydroxylamine hydrochloride. Its terpolymers with 4-hydroxyacetophenone (HA)/4-hydroxybenzaldehyde (HB) and formaldehyde (F) were synthesized by using an acid catalyst in 1:1:5 M proportions. The synthesized monomer (APO) and its terpolymers were identified by FT-IR and 1H NMR spectroscopic data. The apparent number average molecular weight and viscosities were determined by gel permeation chromatography and Ubbelohde viscometer, respectively. Thermal stability of these resins was determined by differential scanning calorimetry (DSC). The activation energy (E a) values of the thermal decompsition were investigated with thermogravimetric analysis (TGA) by isoconversional Flynn–Wall–Ozawa method. Empirical kinetic models, as well as generalized master plots, were applied to explain the degradation mechanisms of terpolymers. The terpolymers strongly inhibited the growth of a wide variety of microorganisms, including Gram-positive, Gram-negative bacteria and fungi.
Introduction
Recently, functional polymers endow countless products with properties enhancing the functionality that plays a crucial role in quality as well as they have virtually unlimited potential for innovations. Advantages of functional polymers are low cost, ease of processing, and a range of attractive mechanical characteristics for functional organic molecules. The use of functional terpolymers in all sphere of life has increased abundantly increased in recent years because of their large applications like packaging, adhesives and coatings in electrical sensor, electroluminescent devices, hardener for epoxy resin, ion exchangers, thermal stable, and antimicrobial materials.Citation[1–9] Singru and co-workers Citation[10] studied the electrical property, thermal stability, morphology, photoluminescence, and ion-exchange properties of p-cresol-formaldehyde-melamine terpolymers resin. A polyacrlonitile (PAN) has been prepared with amidoxime-nitrile-3 hydroxyphenyl-1,2,4-oxadiazole (ANHOT) as pendant group has been prepared. Soykan and Erol Citation[11] synthesized oxime, carbazone, and thiosemicarbazone derivatives of N-(4-acetylphenyl) maleimide and their homopolymers by free radical polymerization having antimicrobial activities. polyethylene glycol (PEG) is produced by the interaction of ethylene oxide with water. Zhao et al. Citation[12] prepared a series of amphiphilic poly (ether-anhydrides) terpolymers composed of sebacic acid, 1,3-bis(carboxyphenoxy)propane and poly(ethylene glycol) via melt-condensation polymerization. These PEG-based ether-anhydride terpolymers may be of great potential as nanoscaled carriers for drug delivery system. Similarly, one of the most frequently used peptides for surface coating is peptide that contains Arg–Gly–Asp (RGD) amino acid sequence. RGD sequence is recognized by cell-surface receptors, such as integrins, which also known to mediate cell adhesion. Fussel and Cooper Citation[13] synthesized acrylic terpolymers with RGD peptides for biomedical applications. Madkour et al. Citation[14] prepared end functionalize living polymer chains of allyl-based terminating agents by ring-opening metathesis polymerization (ROMP), which are attractive in the biomedical field. Murthy et al. Citation[15] synthesized terpolyperoxide (TPPE) by the oxidative terpolymerization of styrene, methyl methacrylate, and α-methylstyrene. TPPE is tetrapolymer of constituents with peroxide linkage. Yang and Sun Citation[16] prepared terpolymers of N-cyclohexylmaleimide, methyl methacrylate, and acrylonitrile by suspension polymerization. Samal and co-workers Citation[17] synthesized terpolymers of phenolic Schiff bases derived from o-, m-, and p-hydroxybenzaldehydes and 4,4-diaminodiphenyl ether with formaldehyde by polycondensation technique.
The disadvantage of a surface attachment/grafting approach is that antimicrobial activity is lost after the surface layer is worn off. This disadvantage can be eliminated by incorporating functional groups like hydroxyl, acetyl, formyl, and oxime groups into the bulk resin rather than using it as a coating.
Nevertheless, up to date only few efforts have been made to study terpolymers with excellent biocidal and thermal properties, and much more investigations are thus highly required for obtaining deep understanding of them. More important, unique properties and large potential applications are expected from such novel terpolymer architectures. In view of the above, in this study, I thought the functional groups like hydroxyl, oxime, pyridinium, and acetyl groups, as a technicophore that can be responsible to tune the antimicrobial properties of the terpolymers. The antibacterial activity of the quaternary N+ polymers is largely driven by the alkyl chain branching and hydrophobicity of the cation.Citation[18–20]
Polymeric biocides can be made by grafting an antimicrobial agent to their surfaces or blending with a non-leaching biocide. A partially hydrolyzed resin formulation containing 4-acetylpyridine oxime, formaldehyde, and acetophenone retains kill-on-contact microbiocidal activities, which should contribute to hospital associated infections. Apart from the potential to prevent against microorganism for long time, incorporation of more biocidal functional groups in terpolymers may impart additional benefits, such as improved polymerization characteristics and the ability to self-repair damage caused by water sorption, and mechanical stress over time.
In this research, a monomer (E)-4-acetylpyridine oxime and its terpolymers with 4-hydroxyacetophenone/4-hydroxybenzaldehyde and formaldehyde were synthesized. The structures and physicochemical characteristics were elucidated by FT-IR, 1H NMR, and non-aqueous conductometric titrations. Furthermore, thermal stability and degradation kinetics of these terpolymers were also investigated by DSC and thermogravimetry-derivative of thermogravimetry analysis. Additionally, thermal degradation mechanisms of terpolymers were also evaluated using Craido method. The antimicrobial activities of monomer and its terpolymers against different microorganism species were also studied. I hope that such novel terpolymers will find new potential applications for instance as smart materials, antimicrobial materials, etc.
Experimental
Materials
4-Acetylpyridine (Sigma–Aldrich, Mumbai) and hydroxylamine hydrochloride (Thomas Baker, Mumbai) were analytical grade and were used as received. 4-Hydroxyacetophenone (Merck, India) and 4-hydroxybenzaldehyde (SRL, India) were purified by rectified spirit. Formaldehyde (37%) was used as received from Merck, India.
All the other chemicals, solvents, and indicators were analytical grade procured from SD Fine chemicals, Mumbai, India. All the solvents were distilled before use.
Preparation
Synthesis of (E)-4-acetylpyridine oxime (APO)
Hydroxylamine hydrochloride (25.0 g, 0.36 mol) was dissolved in 50 mL of water, and the solution was added to 70 mL of 20% aqueous sodium hydroxide in a 500-mL Erlnmeyer flask. It was then magnetically stirred, and at one time, 4-acetylpyridine (36.3 g, 0.30 mol) was added, where precipitate formed rapidly. The reaction mixture was stirred at 0–5 °C for two hours, and then, the precipitate was collected by suction filtration and washed with 500 mL of cold water.
The E- and Z-isomers of these oximes are distinguished by their melting points (E-isomer at 157 and 147 °C for Z-isomer). Therefore, the product, (mp. 146 °C, 33–36 g, yield 81–88%), was recrystallized twice to obtain pure E-isomer. The crude product was dissolved in 600 mL of hot water in a 2-L Erlenmeyer flask, and the hot solution was decanted to remove any undissolved residue. Then, the supernatant liquid was allowed to cool slowly to 30 °C during 2–3 h by placing the flask on a cork ring. The precipitate was collected at this temperature by suction filtration. Second crystallization by the same procedure yields pure E-oxime, which was dried under reduced pressure over Drierite to constant weight. The yield of (E) 4-acetylpyridine oxime, mp 157 °C, is 27.1 g (Yield 66%). In dimethyl sulfoxide-d6, the E and Z isomers show OH proton resonance at δ 11.65 and 10.97 ppm, respectively.Citation[21]
The structure of monomer, APO, was identified by FT-IR and 1H NMR spectra and it was found to be consistent with the assigned structure of (E)-4-Acetylpyridine oxime.
IR (KBr, cm−1): υ = 3413 and 3425 (oxime > NOH), 3021(C–H stretching of acetyloxime group), 1516 and 1417 (aromatic C=C stretching) and 931 (N–O stretching).
1H NMR (CDCl3, ppm): δ = 11.72 (H; –C=N–OH), 8.59–7.59 (4H, Pyridine ring-H), and 2.58–2.1 (3H, CH3).
Synthesis of terpolymers
The terpolymers were synthesized by condensation reaction of (E)-4-acetylpyridine oxime (0.01 mol), condensing reagent formaldehyde (0.05 mol) and 4-hydroxyacetophenone/4-hydroxybenzaldehyde (0.01 mol) using 6 M HCl as the reaction medium and refluxing with occasional shaking at 100–120 °C for 8–10 h in an oil bath to ensure thorough mixing. The solid resinous product was removed immediately from flask. It was washed with cold water and dried. The shinning black colored resins separated out. It was washed with warm water and methanol to remove unreacted monomers. Then, it was extracted with diethyl ether to remove excess of copolymers (4-acetylpyridine oxime-formaldehyde (APOF), 4-Hydroxyacetophenone-formaldehyde (HAF) or 4-Hydroxybenzaldehyde-formaldehyde (HBF)), which might be present along with 4-acetylpyridine oxime-formaldehyde-hydroxyacetophenone (APOFHA)/4-acetylpyridine oxime-formaldehyde-hydroxybenzaldehyde (APOFHB) terpolymer resin. Finally, the terpolymers were air-dried. The dried sample was then dissolved in 10% NaOH and regenerated using 1:1 (v/v) HCl/water. It was filtered off and cured in an air oven at 60 °C for 48 h. The yield was APOFHA (41%) and APOFHB (56%) [Scheme ].
Measurements
Spectral analysis
The FT-IR spectra of the synthesized terpolymers have been scanned in KBr pellets on a Perkin-Elmer (model 2005) spectrophotometer to identify the linkage and the functional groups.1H NMR spectra of polymer samples were taken in CDCl3 on Bruker DPX – 300 MHz NMR spectrometer. Tetramethylsilane (TMS) was used as internal reference.
Viscosity and molecular weight measurements
Intrinsic viscosity [η] was measured in DMSO at 30 °C using Ubbelohde suspended level capillary viscometer. The relative and specific viscosities were measured at five concentrations in the range (0.002–0.010 g/mL). The value of [η] was determined by extrapolating the reduced and inherent viscosities to infinite dilution.
The average molecular weight and its polydispersity index were determined with GPC analysis. It was performed with a setup consisting of a Waters 600 pumps and 2 ultra styragel columns (104, 500Ao) with THF as the eluent at a flow rate of 1 mL/min equipped with Waters differential refractometer and calibrated with the standard linear polystyrene (PSt).
Thermal analysis
DSC analysis with a Mettler Toledo (Model AG 2007) equipped with a thermal analysis data station enabled the curing exotherms of terpolymer resins to be determined. This study was performed with a sealed aluminum capsule pan, under a nitrogen atmosphere, at four different heating rates (10, 20, 30, and 40 °C/min) between 30 and 250 °C. Terpolymers were subjected to TGA in air atmosphere at four different heating rates (10, 20, 30, and 40 °C/min) from 30 to 600 °C with a thermogravimetric analyzer (Mettler Toledo AG 2007). The flow of purging air was kept at 80 mL/min. For the removal of moisture from the resins, the resins were mounted in a vacuum-dried oven at 30 °C for 48 h.
Assay for antimicrobial activities against microorganisms
Microbial tests were performed to examine antimicrobial properties of the monomer and terpolymers against Escherichia coli, Staphylococus aureus, Pseudomonas aeuroginosa, Bacillus subtilis, Alternaria solani, and Fusarium oseysporum. In antimicrobial experiments, zone of inhibition and activity index was determined with respect to standard drugs (Ciprofloxacin for antibacterial and Amphotericin-B for antifungal activities). Cup or well method was used for antibacterial studies, whereas poisoned food method was used for antifungal scanning.Citation[22] Concentrations of samples for antibacterial and antifungal activity were taken as 500 μg/mL.
Results and discussion
Structural characterization
The IR spectra of monomer and terpolymers are shown in Figure . In the range of 3375–3425 cm−1, a strong absorption band indicates the presence of OH in oxime group of monomer moieties. At 1634–1666 cm−1, an intense band specific to carbonyl groups was present. The peak at 1439–1516 cm−1 is due to C=N stretching vibrations of oxime group in monomer moieties, whereas peak around 931 cm−1 is due to N–O stretching in oxime. Appearance of a peak ranging from 1372 to 1439 cm−1 is due to methylene (–CH2) vibration of condensing reagent, which confirm the structure of terpolymers. The peaks characteristic to para-substituted benzene exhibit specific vibrations in the range of 767–841 cm−1.
The 1H NMR spectra of monomer and its terpolymers are shown in Figure . The sharp signal 11.7 ppm was observed in monomer (APO) which may be due to protons of oxime group. Disappearance of this signal in CAOFHA and CAOFHB may be due to presence of existence of H-bonding, which makes them self-crosslinked structures. The bands that appear in the region between 7.126 and 8.587 ppm were due to pyridine and aromatic ring protons. The appearance of signals in terpolymers at 3.9–4.1 ppm is assigned for –CH2– bridges between aromatic units. The common peak situated in the range of 1.9–2.5 among APO, APOFHA and APOHB, is attributed to methyl groups.
Solubility
The solubility of the terpolymer is one of the important requirements for practical use of this polymer. The terpolymers were shinning black, easily soluble in chlorinated solvents such as chloroform, dichloromethane, chlorobenzene and in polar aprotic solvents such as dimethylformamide, dimethylsulphoxide, dioxane, N-methyl-2-pyrrolidone, dimethylene propylenurea, and tetrahydrofuran. It was insoluble in hydroxyl-group-containing solvents such as methanol, ethanol, 2-propanol, and water and in hydrocarbons like benzene, toluene, and xylenes.
Viscosity and molecular weights
The inherent viscosities of these terpolymers were found to be 0.19 dL/g for APOFHB and 0.23 dL/g for APOFHA. The number average molecular weights () and polydispersity indices (PDI) are 3496 g/mol (PDI: 1.42) and 4652 g/mol (PDI: 1.47) respectively.
Differential scanning calorimetry
An amount of 6 mg of terpolymer resins was put into a DSC sample pan and covered with an aluminum lid and closed tightly under pressure. It was heated accordingly to the programmed constant heating rate from room temperature to 250 °C. Figure shows DSC thermograms for terpolymers from −50 to 250 °C at heating rate of 10 °C. As seen from DSC curves, the intensity and position of the broad around 78.85 °C (APOFHA) and 92.49 °C (APOFHB) is attributed to the evaporation of water molecules. The heat of volatization for a number of polymers was measured by DSC where area under the endothermic peak was shown to be directly proportional to the heat of volatization. APOFHA shows maximum area under endothermic curve (1102.8 mJ) as compare to endothermic curve (968.43 mJ) for APOFHA. Only APOFHB shows a second endothermic peak at 190.76 °C, which is associated with melting point, significantly depends on the monomer unit ratios in the terpolymer especially on the 4-hydroxybenzaldehyde content. It is known that higher melting points of polymers are associated with many factors including inter- and intramolecular interactions through hydrogen-bonded functional linkages and structural regularity and rigidity of macromolecules. These results indicate that T m significantly depends on the strong acidic 4-hydroxybenzaldehyde unit content in terpolymer. This proposed that the observed characteristic melt phase is the result of formation and melting of the strong hydrogen-bonded network structure.
Thermogravimetric analysis
TGA and DTG thermograms of terpolymers are performed at different scanning rates 10, 20, 30, and 40 °C/min. As evidenced from Figure , TGA–DTG curves have characteristic three-step decomposition regions. From Table , as the heating rate increased, the decomposition temperature (T d) increased. T d was obtained from the point at which the weight loss increased by DTG. The first weight loss region with DTG endo-peaks associated with dehydration of partially hydrolyzed 4-Acetylpyridine oxime (APO) units; secondary endo-effects can be related to scission of weak links in the terpolymer (intramolecular H-bonding between acetyloxime group of monomer and protons of methylene bridge); at last, more intensive endo-peaks indicate the main-chain degradation reaction. These terpolymers have shown high thermal stability that is probably due to the presence of more APO unit contents.
Figure 4 TGA and DTG plots obtained for APOFHA and APOFHB at (a) 10 °C/min (b) 20 °C/min (c) 30 °C/min (d) 40 °C/min.
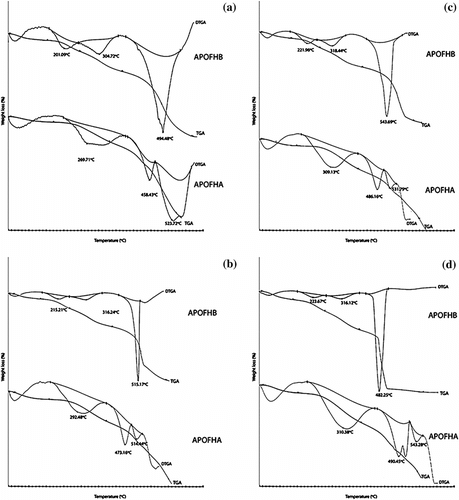
Table 1. Kinetic parameters of APOFHA and APOFHB in different regions obtained by Flynn–Ozawa–Wall analysis#.
Thermal decomposition kinetics
In the thermal decomposition process of polymers, the degree of thermal decomposition or conversion (α) can be typically calculated as follows:
Using this equation, theoretical approaches based on differential mode and integral mode were applied for the calculation of thermal decomposition kinetic parameters.
The Flynn–Wall–Ozawa method Citation[23] has been used to determine the activation energy from dynamic tests by plotting the logarithm of heating rate as a function of the inverse of the temperature at different conversions. Being integrated with the initial condition of α = 0 at T = T o, Equation (4) can be arranging as follows:
Then, using Doyle‘s approximation, Citation[23] Equation (5) can be simplified as follows:
The slope of such a line is given by −0.457E/R. Using this method, E a values for each conversion from 0.10 to 0.90 are obtained from the slope of line plotted in Figure for APOFHA and Figure for APOFHB. The E a values for each α value was calculated from the slope of each straight line, as listed in Table . The E a value of thermal decomposition curves for APOFHA and APOFHB at the degradation conversion (α) was obtained and is shown in Figure . It can be seen from these results that the E a values of thermal decomposition for the APOFHA at the initial stage were quite high compared with the APOFHB. It indicates that APOFHA has more extent of crosslinking as compared to APOFHB. The mean activation energy of decomposition for APOFHA (124.7 kJ/mol) was twice as compare to APOFHB (60.1 kJ/mol).
Table 2. E a values of the thermal decomposition for a APOFHA and APOFHB from Flynn–Wall–Ozawa expression.
Thermodegradation mechanism by Criado method
Thermodegradation mechanism was established by using Criado method.Citation[24] In order to find out the reaction mechanism, we have employed reference theoretical master curves. A master plot is a characteristic curve independent of the condition of the measurement.
Knowing previously the activation energy by some other method, (in this case with the Flynn–Wall–Ozawa method), where it is not necessary to know the reaction mechanism, and defining the function-
The fourth rational expression of P(x)
When x > 20, the error of Equation (9) is less than 10−5%.
Combining equations (2), (7) and (8), we obtain:
From Equations (3), (8), and (9), the following relationship can be derived:
The Z(α) − α master curves can be plotted using Equation (10) according to different reaction mechanism shown in Table . The experimental data at 10 °C/min obtained by Flynn–Wall–Ozawa method (Table ) were substituted into Equation (11). Figures and show the Z(α) − α master and experimental curve of the terpolymer APOFHA and APOFHB, respectively. The results for APOFHA show that the experimental curves of all three steps of degradation belong to D3 reaction mechanism (three dimension diffusion; Jander equation) to D2 reaction mechanism (two dimension diffusion; Valensi equation) whereas APOFHB exhibited D3 reaction mechanism (three dimension diffusion; Jander equation) for all three stages of degradation. This results clearly indicates that APOFHA has higher extent of crosslinking as compared to APOFHB.
Table 3. Algebraic expressions for g(α) and f(α) for the most frequently used mechanisms of solid state processes.
Antimicrobial activities
To study the broad antimicrobial spectrum of APO, APOFHA, and APOFHB, antimicrobial tests on E. coli, B. subtilis, S. aureus, P. aeruginosa, A. solani, and F. oseysporum were carried out. E. coli and P. aeruginosa are Gram-negative bacteria, while B. subtilis and S. aureus are Gram-positive bacteria. A. solani and F. oseysporum are pathogenic fungi. Table and Figure (antibacterial) and Table and Figure (antifungal) show the antimicrobial effects of monomer, terpolymers and standard drugs at a concentration of 500 μg/mL on different microorganisms. Since terpolymers show better antimicrobial properties than monomer (APO), the introduction of comonomers (4-hydroxyacetophenone/4-hydroxybenzaldehde) could greatly improve the antimicrobial activities of terpolymers.
Table 4. Antibacterial data for APO, APOFHA, and APOFHB and Ciprofloxacin.
Table 5. Antifungal data for APO, APOFHA, and APOFHB and Amphotericin -B.
Antimicrobial activity of polymers is affected by their composition, molecular weight, nature of the counter anions and length of the alkyl chain.Citation[25] In this study, the bactericidal activity of APO and its terpolymers against both the test organisms was observed to be dependent both on the nature of the test organism and on the nature of the counter ion in the terpolymer. The antimicrobial activity of the polymers is result of the disruption of the cell membranes when there is an interaction between the microbes and terpolymers. The presence of hydroxyl groups in co-monomer moieties and pyridinium ions in monomer moieties is responsible to exhibit the strongest antibacterial activity of terpolymers compared with standard drug and monomer APO. The alignment of the terpolymer chains and determines the extent of interaction between the microbes and the polymer, hence the differences in the antimicrobial activity.
The antibacterial mechanism of this polycationic disinfectant can be summarized in the following six steps. (1) Adsorption onto the bacterial cell surface; (2) Diffusion through the cell wall; (3) Binding to the cytoplasmic membrane; (4) Disruption of the cytoplasmic membrane, (5) It is possible that by the further action of the quaternary iminium groups, intracellular contents are leaked, such as K+ ions, DNA and RNA; (6) Finally, the death of bacteria cells is led to probably 4-acetylpyridine oxime itself is known to have strong antimicrobial activities.Citation[26] The proposed inhibitory mechanism of terpolymers is that the bacterial cell surfaces are negatively charged and the cationic terpolymer resin can bind to and disrupt cytoplasmic membrane of microorganism, when it is adsorbed on the cell wall.
The antifungal activity emanates from the inhibition of preparation of or direct interaction with ergosterol, which is the predominant component of the fungal cell membrane, and the result the disruption of cell membrane and prevention of the microbe biofilm formation.Citation[27] The polymer structure can induce ion exchange on the fungal cell wall, causing divalent ions from the fungal cell wall to move out of the membrane resulting in the loss of membrane integrity.Citation[28] It was observed that the antifungal activity of the terpolymers is also dependent on functional groups like hydroxyl groups.
Conclusions
A monomer (APO) and its terpolymer (APOFHA and APOFHB) were prepared in hydrochloric acid medium by condensation techniques. From the FT-IR and 1H NMR spectral studies, the proposed structure of the monomer and terpolymers were confirmed. Thermal reactions were also determined with differential scanning calorimetry, to assess their utility as thermal stable material for biomedical applications. Activation energies of degradation were calculated by the Flynn–wall–Ozawa method. It can be seen that APOFHA has superior thermal stability as compared to APOFHB, but APOFHA has lower peak temperatures of degradation, narrower degradation temperature ranges and higher amount of residual weights at end of degradation, which could be attributed to the chemical structure and microstructure features including the steric effect and extent of cross linking. The biocidal effect of different functional groups like hydroxyl, acetyl, pyridinium ions, and oxime groups was markedly established on the efficiency and selectivity towards different microbes as the differences in the structure of the terpolymers at the surface resulted in different extent of the antimicrobial activity.
The design of antimicrobial polymers to address healthcare issues and minimize environmental problems is an important endeavor with both fundamental and practical implications.
Acknowledgement
Author is thankful to HASETRI, JK Tyre, Rajsamand; CDRI, Lucknow and SICART, Vallabh Vidyanagar for analytical and spectral data of these samples.
References
- Ahmari , A , Mousavi , SA , Amini-Fazl , A , Amini-Fazl , MS and Ahmari , R . 2013 . Dextran-graft-poly(hydroxyethyl methacrylate) gels: a new biosorbent for fluoride removal of water . Des. Monomers Polym. , 16 : 127 – 136 .
- Saxena , S and Bajpai , SK . 2010 . Is a cationic-resin-loaded polymeric gel a better antibacterial material than a nanosilver-loaded gel? . Des. Monomers Polym. , 13 : 157 – 165 .
- Mustata , F and Bicu , I . 2006 . P-aminobenzoic acid/cyclohexanon/formaldehyde resins as hardner for epoxy resins. Synthesis and characterization . J. Optoelectron. Adv. Mater. , 8 : 871 – 875 .
- Baradie , B and Shoichet , MS . 2005 . Novel fluoro-terpolymers for coating applications . Macromolecules , 38 : 5560 – 5568 .
- Gan , LH , Kang , ET and Liau , CY . 2001 . Synthesis of intrinsically soluble terpolymers with conjugated rigid block alternating with hexane-1,6-dioxy soft block . Polymer. , 42 : 1329 – 1336 .
- Chauhan NPS, Structural and thermal characterization of macro-branched functional terpolymer containing 8-hydroxyquinoline moieties with enhancing biocidal properties. J. Ind. Eng. Chem. Forthcoming. 2012.
- Li , G and Shen , J . 2000 . A study of pyridinium-type functional polymers. IV. Behavioral features of the antibacterial activity of insoluble pyridinium-type polymers . J. Appl. Polym. Sci. , 78 : 676 – 684 .
- Kanaway , EIR , Abdel-Hay , FI , EI-Magd , AA and Mahmoud , Y . 2006 . Synthesis and antimicrobial activity of some crosslinked copolymers with quaternary ammonium and phosphonium groups . React. Funct. Polym. , 66 : 419 – 429 .
- Grisorio , R , Piliego , C , Fini , P , Cosma , P , Mastrorilli , P , Gigli , G , Suranna , GP and Nobile , CF . 2008 . Random terpolymers for electroluminescent devices: Synthesis and characterization of new cyano-containing poly(fluorenylene-vinylene) . J. Polym. Sci., Part A: Polym. Chem. , 46 : 6051 – 6063 .
- Singru , RN , Gurnule , WB , Khati , VA , Zade , AB and Dontulwar , JR . 2010 . Eco-friendly application of p-cresol-melamine-formaldehyde polymer resin as an ion-exchanger and its electrical and thermal study . Desalination. , 263 : 200 – 210 .
- Soykan , C and Erol , I . 2003 . Synthesis, characterization, and biological activity of N-(4-acetylphenyl) maleimide and its oxime, carbazone, thiosemicarbazone derivatives and their polymers . J. Polym. Sci., Part A: Polym. Chem. , 41 : 1942 – 1951 .
- Zhao , A , Zhou , Q , Chen , T , Weng , J and Zhou , S . 2010 . Amphiphilic PEG-based ether-anhydride terpolymers: synthesis, characterization, and micellization . J. Appl. Polym. Sci. , 118 : 3576 – 3585 .
- Fussell , GW and Cooper , S . 2004 . L Synthesis and characterization of acrylic terpolymers with RGD peptides for biomedical applications . Biomaterials. , 25 : 2971 – 2978 .
- Madkour , AE , Koch , AHR , Lienkamp , K and Tew , GN . 2010 . End-functionalized ROMP polymers for biomedical applications. Macromolecules , 43 : 4557 – 4561 .
- Murthy , KS , Kishore , K and Mohan , VK . 1996 . Synthesis and characterization of tetrapolymers of styrene, methyl-methacrylate, alpha-methylstyrene, and Oxygen . Macromolecule. , 29 : 4853 – 4858 .
- Yang , L and Sun , D . 2007 . Synthesis and characterization of terpolymer of n-cyclohexylmaleimide, methyl methacrylate and acrylonitrile . J. Appl. Polym. Sci. , 104 : 792 – 796 .
- Samal , S , Das , RR , Dey , RK and Acharya , S . 2000 . Chelating resins VI: Chelating resins of formaldehyde condensed phenolic Schiff bases derived from 4,4′-diaminodiphenyl ether with hydroxybenzaldehydes – synthesis, characterization, and metal ion adsorption studies . J. Appl. Polym. Sci. , 77 : 967 – 981 .
- Chauhan , NPS and Ameta , SC . 2011 . Preparation and thermal studies of self-crosslinked terpolymer derived from 4-acetylpyridine oxime, formaldehyde and acetophenone . Polym. Degrad. Stab. , 96 : 1420 – 1429 .
- Chauhan , NPS . 2012 . Terpolymerization of p-acetylpyridine oxime, p-methylacetophenone and formaldehyde, and its thermal studies . J. Therm. Anal. Calorim. , 110 : 1377 – 1388 .
- Chauhan , NPS . 2012 . Isoconversional curing and degradation kinetics study of self-assembled thermo-responsive resin system bearing oxime and iminium groups . J. Macromol. Sci., Pure Appl. Chem. , 49 : 706 – 719 .
- Aakeröy , CB , Beatty , AM and Leinen , DS . 2000 . Syntheses and crystal structures of new ‘extended’ building blocks for crystal engineering: (Pyridylmethylene) aminoacetophenone oxime ligands . Cryst. Growth Des. , 1 : 47 – 52 .
- Chauhan , NPS , Ameta , R and Ameta , SC . 2011 . Synthesis, characterization, and thermal degradation of pchloroacetophenone oxime based polymers having biological activities . J. Appl. Polym. Sci. , 122 : 573 – 585 .
- Chauhan NPS. Facile synthesis of environmental friendly halogen-free microporous terpolymer from renewable source with enhanced physical properties, Des. Monomers Polym. 2012; 15, 587–600.
- Chauhan , NPS . 2012 . Preparation and thermal investigation of renewable resource based terpolymer bearing furan rings as pendant groups . J. Macromol. Sci., Pure Appl. Chem. , 49 : 655 – 665 .
- Kenawy , AER , Worley , SD and Broughton , R . 2007 . The chemistry and applications of antimicrobial polymers: a state-of-the-art review . Biomacromolecules. , 18 : 1359 – 1384 .
- Abele E, Abele R, Lukevics E. Pyridine oxime: synthesis and biological activity. Chem. Heterocyc. Compd. 2003, 39:825–865.
- Holt RJ, The imidazoles, In: Speller DCE, editor. Antifungal Chemotherapy. Chichester, England: Wiley; 1980. P. 107–148.
- Kugler , R , Bouloussa , O and Rondelez , F . 2005 . Evidence of a charge-density threshold for optimum efficiency of biocidal cationic surfaces . Microbiology. , 151 : 1341 – 1348 .