Abstract
Chalcone derivative and their polymers are synthesized, characterized, tested for their antimicrobial activity against four different micro-organisms. In vitro cumulative drug release studies are done using UV–visible spectroscopic technique. 2,4-Dichloro-1-ene(4-hydroxyphenyl)phenone (DHP) is synthesized using 4-hydroxy benzaldehyde and 2,4-dichloro acetophenone. 4-[3-(2,4-Dichloro-phenyl)3-oxoprop-1-en-1-yl]phenylacrylate (DCP) is prepared by reacting DHP with acryloyl chloride. Copolymer of DCP is prepared using acrylic acid and hydroxyl ethyl acrylate. The drugs are characterized by IR, 1H NMR, and UV–visible spectroscopic techniques. Molecular weight of the polymer is 4000 g/mol. First decomposition temperature of the polymers is around 320 °C. MIC value of the drug is very high for gram-negative bacteria (1.95 μg/mL). In vitro drug release rate is dependent on the comonomer, pH, and temperature.
1. Introduction
The use of polymeric systems with pharmacological activity provides very good local activities reducing the toxicological risks and in addition could act as a release system of the pharmacological active residue, controlled by chemical reactions, mainly hydrolytic under enzymatic and non-enzymatic processes. Generally, drug conjugates are usually prepared by esterification, acrylation, or alkylation. By attaching bioactive substrates to the synthetic or naturally occurring macromolecules, it is expected to increase the therapeutic efficiency while lowering their toxicity. Generally speaking, the activity of the polymeric drug is related to the functional group and the nature of the polymeric substance. According to Vogl et al. [Citation1], the introduction of hydrophilic, hydrophobic, or polyelectrolytic moieties will enhance the activity of the drug on micro-organisms. Also, it is observed that poly(methacrylic acid) has no effect on the micro-organisms like Escherichia coli. Incorporating hydroxyl or carboxylic groups in the polymer backbone could infact increase the hydrophilic character of the polymeric drug. Most of the synthesized polymeric drugs having high hydrophilic character are produced by copolymerizing the drug conjugates with acrylic acid as a hydrophilic part. The advantage of acrylic acid as a comonomer in a polymer chain bearing active drug moiety is its ability to assist the cleavage of amide or ester linkage through neighboring group participation mechanism. These properties make such systems quite interesting and provide longer delivery times with lower dosages.
Chalcones are condensed products of the substituted aromatic aldehydes with simple or various substituted acetophenones in the presence of alkali. Compounds with the backbone of chalcone possess various biological activities such as anti-microbial,[Citation2] anti-inflammatory,[Citation3] analgesic,[Citation4] anti-ulcerative,[Citation5] immune-modulatory,[Citation6] antimalarial,[Citation7] anti-leishmanial,[Citation8] anti-oxidant,[Citation9] antiplatelet,[Citation10] etc. Presence of the reactive keto group and the vinylenic group in the chalcone and their analogues possesses the antioxidant activity.[Citation11]
Antimicrobial activity: Nagaini et al., successfully have synthesized a new series of chalcone derivatives as antimicrobial agents and have found that the position of functional group would have a pronounced effect on the anti-bacterial effect against E. coli.[Citation12] Swamy and Agasimundin have reported the antimicrobial activity of 3-hydroxy benzofuran-substituted chalcones. It is evident that most of the compounds are very weakly active and few are moderately active against Staphylococcus aureus and E. coli but they possess very good activity against fungi Aspergillus flavus.[Citation13] Similarly, Mayekar et al. have reported a series of chalcones and its cyclohexanone derivatives derived from 6-methoxy-2-naphthaldehyde. They show comparatively good activity against all the bacterial and fungal strains like E. coli, S. aureus, Pseudomonas aeruginosa, when compared to standard drugs like Ampicillin.[Citation14] de Carvalho Tavares et al. have tested a series of new 6-quinolinyl and quinolinyl N-oxide chalcones on the survival and growth of the human cancer cell lines UACC-62 (melanoma), MCF-7 (breast), TK-10 (renal), and leukemic cells, Jurkat and HL60.[Citation15] Similarly, Nakagawa-Goto et al. have reported the synthesis of a chalcone from commercially available 2, 4, 6-trihydroxy toluene, which shows a unique highly potent anti-HIV activity.[Citation16] With a view to develop an efficient antifungal/antibacterial active polymer conjugates, the present work investigates the biological activity of the chalcone polymers containing chlorine as biologically active sites. Also these compounds can be associated with the antioxidant properties due to hydroxyl and phenyl group in the polymer backbone. Polymeric drug based on acrylates has a higher activity against bacterial strains.[Citation17] This work discusses the synthesis of a chalcone and acrylate derivative of DHP using 4-hydroxy benzaldehyde and DCA. Antimicrobial activities of DHP, DCP, poly(DCP), poly(DCP-co-AA), and poly(DCP-co-HEA) are tested on four different bacterial strains. The drug release studies are carried out using UV–visible spectrophotometer.
2. Experimental section
2.1. Materials and methods
4-Hydroxy benzaldehyde and acrylic acid were used as received from Aldrich Chemicals. 2,4-Dichloro acetophenone (DCA) and triethyl amine (TEA) were received from SD Fine Chemicals. Ethyl methyl ketone (MEK), acrylic acid (AA), hydroxy ethyl acrylate (HEA), and acroloyl chloride were used as received from Merck. ALPHA BRUKER FT-IR spectrophotometer was used for recording IR spectrum and the spectra were recorded using KBr pellet method. 1H NMR spectra of the samples were run on a Bruker FT-NMR spectrophotometer operating at 500 MHz’s using CDCl3 as a solvent and tetramethyl silane as an internal reference. UV absorption measurements were recorded using LABINDIA model UV 320 instrument by dissolving polymer samples in tetrahydrofuran (THF).
Thermal analyses of the polymers were done with a Mettler 3000 thermal analyzer at the heating rate of 10 °C/min in air. The molecular weight of the polymer was determined using shimadzu instrument with THF as an eluent at a flow rate of 0.3 mL/min. Muller-Hinton broth and Muller-Hinton agar were obtained from Himedia and used as such. MTCC stains of gram-negative bacteria (E. coli (739), P. aeruginosa (424)) and gram-positive bacteria ((S. aureus (3381), Bacillus cereus (430)) were obtained from the Christian Medical College (CMC), Vellore, India and used for culturing the bacteria.
2.2. Synthesis of 1-(2,4-dichlorophenyl)-3-(4-hydroxyphenyl)prop-2-en-1-one (DHP)
Into a conical flask, containing 30 mL of ethanol were added 0.025 mol (4.73 g) of DCA and 0.025 mol (3.05 g) of 4-hydroxy benzaldehyde. To this solution, 4 g of sodium hydroxide dissolved in 20 mL of distilled water was added dropwise at room temperature. The contents of the flask were stirred for 20 h at room temperature. The mixture was neutralized using dilute hydrochloric acid and the precipitate formed was filtered, washed with cold water, and dried in vacuum at 40 °C. Yield: 6.9 g (89%) and the m.p. is 110–112 °C. 1H NMR (500 MHz, DMSO-d6, δ): 7.4–7.7 (m, 7H aromatic H) and 7 (d, 1H vinylic CH) and 6.9(d, 1H vinylic CH). FT-IR (cm−1) 3184 (–OH), 3030 (aromatic CH-stretching), 2985 (aliphatic CH-stretching), 1695 and 1646 (cis & trans C=O) and 1588 (aliphatic CH=CH).
2.3. Synthesis of 4-(3-(2,4-dichlorophenyl)-3-oxoprop-1-enyl)phenylacrylate (DCP)
Into a 500 mL round-bottom flask, fitted with a mechanical stirrer, 150 mL of MEK 0.01 mol (2.92 g) of EHP and 0.012 mol (1.67 mL) of TEA were added. Using a pressure equalizing addition funnel, 0.012 mol (1.20 mL) of acryloyl chloride dissolved in 30 mL of MEK was added dropwise with constant stirring and the temperature was maintained at 0–5 °C. After the addition, the contents of the flask were stirred for 3 h at room temperature and the solid material formed was removed by filtration. The filtrate was washed with water and the organic layer was dried with anhydrous sodium sulfate. The product obtained by evaporating the solvent. Yield = 2.9 g (79%) and the m.p. is 84–86 °C. 1H NMR (500 MHz, DMSO-d6, δ): 7.4–7.7 (m, 7H Ar H), 7.1 (d, 1H vinylic CH), 7.0 (d, 1H vinylic CH),6.6 (t, 1H vinylic CH) and 6.3 (d, 2H vinylic CH2). FT-IR (cm−1): 3036 (aromatic CH-stretching), 2985 (aliphatic CH-stretching), 1748 (−C=O of ester), 1697 and 1646 (cis & trans C=O), 1591 (vinylic) and 1583 (aliphatic CH=CH).
2.4. Synthesis of poly(DCP)
DCP (1.0 g) and 0.05 g of BPO (5 wt.%) were placed in a polymerization tube containing 10 mL of MEK. The polymerization tube was degassed and heated at 70 ± 1 °C in a thermostatic water bath for 24 h. Poly(DCP) was precipitated by adding the reaction mixture to a large excess of methanol with rapid stirring. Yield = 0.9 g (90%). 1H NMR (500 MHz, DMSO-d6, δ): 7.4–7.7 (m, 7H Ar H), 7.1 (d, 1H vinylic CH), 7.0 (d, 1H vinylic CH) and 0.9–1.3 (m, 3H alkane). FT-IR (cm−1): 3040 (aromatic CH-stretching), 2986 (aliphatic CH-stretching), 1744 (–C=O of ester), 1693 and 1642 (cis & trans C=O) and 1583 (aliphatic CH=CH).
2.5. General procedure for the preparation of copolymer
Copolymerization was done using solution polymerization technique. Monomer 1 and monomer 2 were taken in a polymerization tube containing MEK as a solvent and BPO (5 wt.%) as a free radical initiator. The reaction medium was made inert by passing the nitrogen gas through the inlet of the polymerization tube. The polymerization was carried out at 70 ± 1 °C for 24 h. The polymer solution was then precipitated in methanol and the precipitate obtained was then filtered in sintered crucible, washed with methanol, and weighed. This method was used for the preparation of the following compounds.
2.5.1. Synthesis of poly(DCP-co-AA)
(0.004 mol) 1.38 g of DCP and (0.004 mol) 0.28 g of AA, 10 mL of MEK and 0.08 g (5 wt.%) of BPO were used for the synthesis of poly (DCP-co-AA). Yield = 0.88 g. 1H NMR (500 MHz, DMSO-d6, δ): 7.4–7.7 (m,7H Ar H) 7.1 (d,1H vinylic CH), 7.0 (d,1H vinylic CH) and 1–1.5 (m, 6H alkane). FT-IR (cm−1): 3040 (aromatic CH-stretching), 2986 (aliphatic CH-stretching), 1743 (–C=O of ester), 1664 (C=O) and 1585 (aliphatic CH=CH).
2.5.2. Synthesis of poly(DCP-co-HEA)
(0.004 mol) 1.38 g of DCP and (0.004 mol) 0.46 g of HEA, 10 mL of MEK and 0.08 g (5 wt.%) BPO were used for the synthesis of poly(DCP-co-HEA). Yield = 1.0 g. 1H NMR (500 MHz, DMSO-d6, δ): 7.4–7.7 (m,7H Ar H), 7.1 (d,1H vinylic CH), 7.0 (d,1H vinylic CH) 4.6 (b, 1H hydroxyl) 3.6 (d, 2H CH2), 3.4 (d, 2H CH2) and 1–1.5 (m, 6H alkane). FT-IR (cm−1): 3336 (–OH), 3036 (aromatic CH-stretching), 2986 (aliphatic CH-stretching), 1740 (–C=O of ester), 1670 (C=O) and 1582 (aliphatic CH=CH).
2.6. Drug susceptibility test
The drugs were tested by disk-diffusion method. Diluted bacterial cultures (100 mL) were spread on sterile Mueller-Hinton agar plates, on which 8-mm diameter disks (sterile blank) impregnated with drug to be tested were placed. The plates were incubated for 24 h at 37 °C under aerobic conditions and the diameter of the inhibition zone around each disk was then measured and recorded. If the drugs were found to be active in the disk-diffusion test (inhibition zone > 10 mm), they were further evaluated for determining minimum inhibitory concentration (MIC) values.
2.7. Minimum inhibitory concentration
The drugs were screened for their anti-bacterial activities against S. aureus (MTCC3381), B. cereus (MTCC430), E. coli (MTCC739), and P. aeruginosa (MTCC424). MIC was evaluated by turbidity method. A loopful of bacteria was inoculated in 100 mL of nutrient broth at 37 °C for 20 h in a test tube shaker at 150 rpm. The test compounds were prepared by dissolving in minimal volume of DMSO and were serially diluted in Muller-Hinton broth at concentration ranging from 1 to 100 μg/mL. The 24-h bacterial cultures were then transferred into 10 mL of Muller-Hinton broth and incubated at 37 °C for 24 h. The growth of the bacteria was determined by measuring the turbidity by optical density reading at 600 nm after 24 h. Thus, MIC was generally read as the smallest concentration of drug in the series that prevents the development of growth of test organism. All the experiments were done in triplicate.
2.8. Statistical analysis
MIC values were measured in triplicate. Statistical analysis of the MIC value was performed using the unpaired student’s t test. Differences were considered significant for the p values of <0.01.
2.9. Preparation of film
200 mg of the drug sample was dissolved in a minimum quantity of the DMSO. The resulting solution was placed into a film forming glass plates. This glass plate was dried in vacuum at 30 °C for 24 h. The film was peeled out from the glass plate and the thickness of the film was measured using electronic screw gage. The thickness of the film was 100–200 μm. This film was used for the controlled drug delivery studies.
2.10. In vitro drug release study
In vitro drug release pattern was studied at different temperatures (37 and 40 °C) and different pH values (7.4 and 9.2) of the medium. Pieces of 1–1.5 cm2 of copolymer films (100–200 μm thickness) were taken and soaked in 10 mL of phosphate-buffered solutions having pH values of 7.4 and 9.2. 5 mL of the solution were periodically collected for analysis and replaced by fresh medium (the same volume). The amount of APA in the medium was determined using UV spectroscopy absorption at λmax = 310 nm. These measurements were performed by an UV–visible detector systronic system. The cumulative drug release was plotted against the time intervals. All the experiments were done in triplicate.
3. Results and discussion
The parent compound chalcone 2,4-dichloro-1-ene(4-hydroxyphenyl)phenone (DHP) and the monomer 4-[3-(2,4-dichloro-phenyl)3-oxoprop-1-en-1-yl]phenyl acrylate (DCP) were prepared according to Schemes and , respectively and characterized by IR and 1H NMR techniques. Poly(DCP) was synthesized using novel DCP monomer according to Scheme . The IR spectrum of DCP is presented in the Figure . The poly(DCP-co-AA) and poly(DCP-co-HEA) (Scheme ) were prepared in EMK by a free radical polymerization technique and were characterized by FT-IR, 1H NMR, and UV to confirm the structure. The thermal stability of the polymers is reported in this paper. The molecular weights of the polymers were determined by GPC. The weight average molecular weights of the polymers were around 4000 g/mol. The polydispersity values of the polymers are less than 2, quantitatively suggesting that the polymer is terminated by disproportion method, which is typical of acrylates. The antimicrobial activities of the polymers were evaluated using MIC method. The drug release studies were carried out using UV–visible spectroscopic technique.
Figure 4. Comparative MIC graph of DCP, poly(DCP), poly(DCP-co-AA), and poly(DCP-co-HEA) on gram-negative bacteria (cylinder = E. coli and cone = P. aeruginosa).
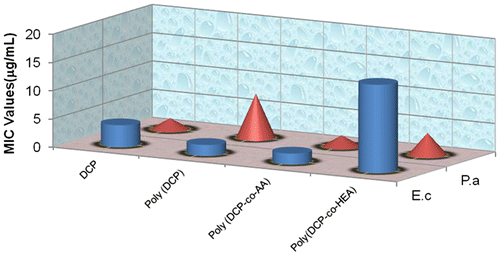
Figure 5. Effect of pH and temperature (■ = pH 9.2 at 37 °C, ♦ = pH 7.4 at 40 °C and ▲ = pH 7.4 at 37 °C) on the drug releasing rate of copoly(APA-AA) film (average ± SD, n = 3).
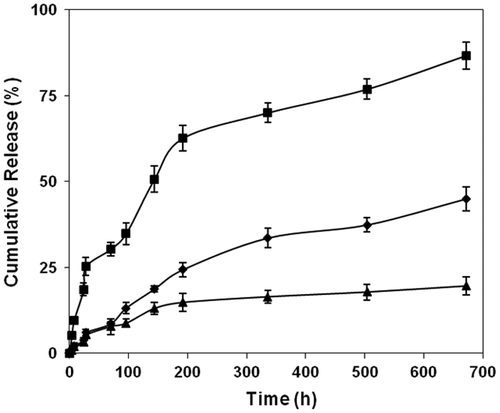
Figure 6. Effect of pH and temperature (■ = pH 9.2 at 37 °C, ♦ = pH 7.4 at 40 °C and ▲ = pH 7.4 at 37 °C) on the drug releasing rate of copoly(APA-HEA) film (average ± SD, n = 3).
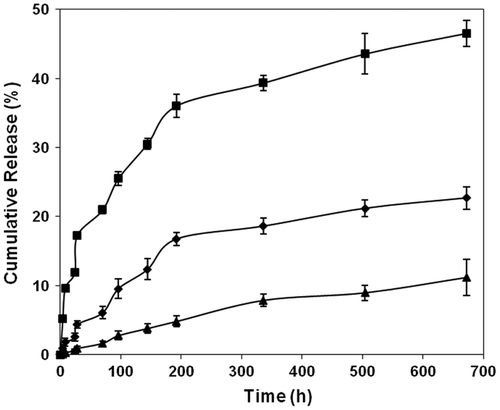
Figure 7. Effect of comonomer type (■ = AA, ▲ = HEA) on the drug releasing rate from the polymer film (pH 9.2 at 37 °C) (average ± SD, n = 3).
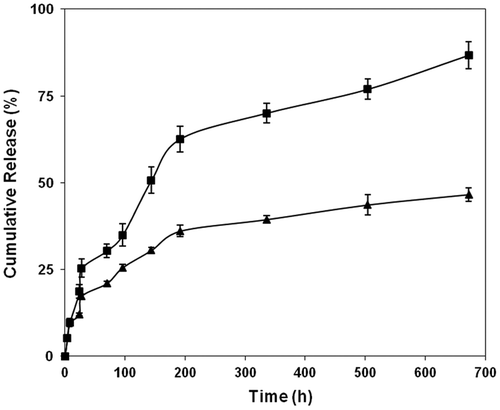
3.1. Solubility
The solubility data of the polymer samples are presented in the Table . It shows that all the copolymers are soluble in DMSO, dimethylformamide, acetone, and tetrahydrofuran, but insoluble in polar solvents like chloroform, water, methanol, and partially soluble in ethanol. The copolymer is not soluble in non-polar solvents like benzene, n-hexane, and carbon tetrachloride.
Table 1. Solubility data of monomer and polymers at 30 °C.
3.2. UV studies
UV–visible spectrum of the poly(DCP) is depicted in Figure as a representative of the polymer series. The Figure reveals two characteristic peaks of absorptions at 243 nm is due to aromatic double bond absorption and at 310 nm is due to π–π* transition of >CH=CH< in the DPA system. Similar to poly(DCP), UV–visible spectra of poly(DCP-co-AA) and poly(DCP-co-HEA) show the peaks of absorptions for aromatic double bond at 230 and 246 nm and >CH=CH< peaks at 315 and 320 nm, respectively. The absorption values of the polymers are presented in the Table .
Table 2. TGA, molecular weight, UV data of the synthesized materials.
3.3. Molecular weights
Molecular weights of the polymer samples were determined using GPC method. For poly(DCP), the weight average molecular weight is Mw = 2.85 × 103, the number average molecular weight is Mn = 1.88 × 103 and the polydispersity index is Mw/Mn = 1.80. Similar trend is observed for other polymer samples in this series and is presented in Table . The observed polydispersity values confirm that the termination is by disproportion (Mw/Mn < 2). Acrylates generally undergo termination by disproportion method.
3.4. Thermal analysis
Thermogravimetric analysis (TGA) was used to estimate the percentage weight loss of the copolymer against temperature. The TGA and DTA curve of poly(DCP) is shown in Figure and the decomposition temperatures are presented in Table . These results show that the poly(DCP) undergoes decomposition in two stages. The first decomposition temperature found around 315 °C and the second decomposition temperature centered around 428 °C. These two stage decomposition temperatures of the copolymer clearly show that the copolymer has higher thermal stability. Similar trends are observed for other polymer samples and the values are presented in the Table . This type of two stage decompositions are reported for similar type of polymeric systems.[Citation18,19]
3.5. Antimicrobial activity
The MIC values (average of triplicates) of DHP, DCP monomer, poly(DCP), poly(DCP-co-AA), and poly(DCP-co-HEA) are shown in Table . The effect of comonomer in the polymer chain on the antimicrobial activity was investigated by comparing the MIC values of DCP, poly(DCP), poly(DCP-co-AA), and poly(DCP-co-HEA). The result is presented as a bar diagram (cylinder = E. coli and cone = P. aeruginosa) in Figure . The results show that the presence of hydrophilic comonomer in the polymer chain enhanced the antimicrobial activity on the gram-negative bacteria. The antimicrobial activities of poly(DCP-co-AA) on the E. coli and P. aeruginosa is found to be very high. The MIC value (1.95 μg/mL) is higher than the MIC values of linezolid (a new oxazolidinone class of compound) which is in the early phase III of human clinical trial. Besides having a higher MIC value, the synthetic procedure of poly(DCP-co-AA) is very simple to become a good competitor for oxazolidinone class of compound. But, in the case of gram-positive bacteria, the antimicrobial activity is found to be decreased (Table ) when active drug moiety is copolymerized with AA or HEA. Differences in the cell wall constituents of microbes might have brought about such a variation in the comonomer effects between the two types of stains. In the case of gram-negative bacteria, the remarkable observation lies in the MIC value of P. aeruginosa. The P. aeruginosa considered to be a highly resistant species among the gram-negative bacteria. Several studies have demonstrated a low susceptibility of P. aeruginosa towards both hydrophobic and hydrophilic antibiotics. The MIC value of poly(DCP-co-AA) over P. aeruginosa was found to be high (1.9 μg/mL), considering the intrinsic resistance capability of the bacterium.
Table 3. MIC values of DCP, poly(DCP), poly (DCP-co-AA) and poly(DCP-co-HEA).
The antimicrobial property of comonomer AA in poly(DCP-co-AA) is not apparent and can be ruled out as poly(AA) showed absence of any antimicrobial activity in similar systems. This provides a clear picture that the antimicrobial activity enhancement of poly(DCP-co-AA) over the poly(DCP) on P. aeruginosa and E. coli was due to the availability of active drug moiety (DHP) adjacent to the cell wall of the micro-organism and/or the opening up of the cell wall to allow the active drug moiety to penetrate through the micro-organism. With regard to the first condition, it is felt that the availability of the drug adjacent to the cell wall was increased due to the polymeric chain forming a cage-like structure around the micro-organism. Such structures could be stabilized by both ionic and hydrogen bonding formed between the polymeric chain and the cell wall of the micro-organism.[Citation20] As for the second possibility, cell wall opening can be triggered by the ionic strength and the hydrophilic nature of the medium adjacent to the cell wall of the micro-organism. In poly(DCP-co-AA), the comonomer AA is involved in increasing the ionic strength of the medium adjacent to the cell wall of the micro-organism. It appears from the results that these two factors operate simultaneously to increase the activity of poly(DCP-co-AA) over poly(DCP) and poly(DCP-co-HEA). The comonomer AA acts as an anchor to deliver the active drug moiety through the above-said two mechanisms.
3.5.1. Effect of comonomer on gram-negative bacteria
When the activity of the poly(DCP-co-AA) is compared with that of poly(DCP-co-HEA) on gram-negative bacteria, the former is found to be much more active than the latter. This might be attributed to a greater ionic strength of AA than HEA which enhances the antimicrobial activity considerably. Moreover, the AA present in poly(DCP-co-AA) can engage in neighboring group participation mechanism for the detachment of active drug moiety from that of the polymeric chain. This also will add on to show that poly(DCP-co-AA) is a very active polymeric drug when compared with poly(DCP) and poly(DCP-co-HEA) toward the gram-negative bacteria.
3.6. Control release studies of copolymers
In vitro drug release pattern for the polymer carrying AA and HEA as a comonomer is shown in the Figures and . The release graph clearly shows that the release rate depends on the pH of the medium. As the pH of the medium is changed from 7.4 to 9.2, the releasing rate is enhanced.[Citation21] This is due to the fact that at blood pH (pH 7.4) medium, the ester linkage that connects the drug to the polymer backbone shows resistance to hydrolysis. The irregular drug releasing pattern may be due to uneven distribution of drug in the polymeric film. Earlier studies have reported a similar type of irregularity in the release of the drug and this can overcome by changing the type of testing sample from the film type to granular type.[Citation22] The in vitro drug release pattern in the acidic medium is not studied here since the ester linkage shows more stability towards hydrolysis [Citation23] in acidic condition. At higher basic medium, the ester linkage is more pronounced to hydrolysis and releases the active drug moiety to the surroundings [Citation24] at a faster rate. The other factor that is responsible for the higher release rate of the drug is the presence of aromatic system of the drug over aliphatic system. This sort of aromatic systems exhibiting faster release rates than the aliphatic system has been observed by many previous research workers.[Citation23] The hydrolysis of ester bond in the basic medium has been reported earlier.[Citation24] A slight increase in the release rate of the drug when the temperature was changed from 37 to 40 °C at pH 7.4 is due to the fact that, as the temperature increases the chain mobility increases which in turn allows the water molecules to penetrate through the polymer matrix to enhance the hydrolysis rate of the system. This sort of chain mobility responsible for the enhancement of release rate and the effect may be more pronounced when the polymer sample is in film form. This type of pH response has got major advantages. One of the most important applications is sustained oral drug delivery system providing from the pH difference between the stomach and the intestine.[Citation25]
A comparative graph between AA and HEA is presented in Figure and it is evident that the polymer sample made of AA releases the drug at a faster rate than the HEA. This is due to the fact that the AA in the polymer backbone assists the hydrolysis through the neighboring group participating mechanism [Citation26] and this type of assistance is absent in the case of polymer-bearing HEA as a comonomer. No doubt the comonomer HEA presents in the polymer chain is involved in increasing the swelling of the polymer matrix thereby allowing the water particles to participate in the hydrolysis of the ester bond, but the effect is comparatively lower than that of AA which effectively participates in the neighboring group participation mechanism.
4. Conclusion
Novel chalcone-type monomer DCP was prepared from DHP and acryloyl chloride. Poly(DCP), poly(DCP-co-AA), and poly(DCP-co-HEA) were prepared using solution polymerization technique. The synthesized polymers were characterized by IR and NMR techniques. The weight average molecular weight of the synthesized polymers was low around 4000 g/mol. Thermal stability of the polymers were very high, first, and second decomposition temperature lies around 320 and 420 °C, respectively. The UV data showed the characteristic peaks for aromatic and vinylic absorption at 240 and 310 nm, respectively. The copolymers showed very high activity against two tested gram-negative bacteria. Remarkable low MIC value is obtained for P. aeruginosa. In vitro drug release pattern of the polymer film was monitored for four weeks using UV–visible spectroscopic technique. It is found that the rate of release increased upon increasing the pH and temperature of the medium. Also, enormous difference in drug release behavior is observed between poly(APA-co-AA) and poly(APA-co-HEA).
References
- Vogl O, Tirrell D. Functional polymers with biologically active groups. J. Macromol. Sci. Chem. 1979;13:415–439.10.1080/00222337908068110
- Ayfer B, Dizman B, Elasri MO, Mathias LJ, Avci D. Synthesis and antibacterial activities of new quaternary ammonium monomers. Des. Monomers Polym. 2005;8:437–451.10.1163/1568555054937935
- Ballesteros JF, Sanz MJ, Ubeda A, Miranda MA, Iborra S. Synthesis and pharmacological evaluation of 2′-hydroxychalcones and flavones as inhibitors of inflammatory mediators generation. J. Med. Chem. 1995;38:2794–2797.10.1021/jm00014a032
- Viana GS, Bandeira MA, Matos F. Analgesic and antiinflammatory effects of chalcones isolated from Myracrodruon urundeuva allemão. J. Phytomedicine. 2003;10:189–195.10.1078/094471103321659924
- Mukarami S, Muramatsu M, Aihara H, Otomo S. Oleic acid as an inhibitor of gastric H+, K(+)-ATPase. Biochem. Pharmacol. 1991;42:337–350. Retrieved from http://www.ncbi.nlm.nih.gov/pubmed/1646470.
- Barfod L, Kemp K, Hansen M, Kharazmi A. Chalcones from Chinese liquorice inhibit proliferation of T cells and production of cytokines' International Immunopharmacology. Int. Immunopharmacol. 2002;2:545–555.10.1016/S1567-5769(01)00202-8
- Liu M, Wilairat P, Go M-L. Antimalarial alkoxylated and hydroxylated chalcones [corrected]: structure-activity relationship analysis. J. Med. Chem. 2001;44:4443–4452.10.1021/jm0101747
- Nielsen SF, Chen M, Theander TG, Kharazmi A, Brøgger Christensen SB. Synthesis of antiparasitic licorice chalcones. Bioorg. Med. Chem. Lett. 1995;5:449–452.10.1016/0960-894X(95)00053-V
- Vogel S, Barbic M, Jürgenliemk G, Heilmann J. Synthesis, cytotoxicity, anti-oxidative and anti-inflammatory activity of chalcones and influence of A-ring modifications on the pharmacological effect. Eur. J. Med. Chem. 2010;45:2206–2213. Retrieved from http://www.ncbi.nlm.nih.gov/pubmed/20153559.10.1016/j.ejmech.2010.01.060
- Zhao LM, Jin HS, Sun LP, Piao HR, Quan ZS. Synthesis and evaluation of antiplatelet activity of trihydroxychalcone derivatives. Bioorg. Med. Chem. Lett. 2005;15:5027–5029.10.1016/j.bmcl.2005.08.039
- Nowakowska Z, Kędzia B, Schroeder G. Synthesis, physicochemical properties and antimicrobial evaluation of new (E)-chalcones. Eur. J. Med. Chem. 2008;43:707–713.10.1016/j.ejmech.2007.05.006
- Nagaini Z, Fadzillah H, Hussain H, Kamaruddin K. Synthesis and antimicrobial studies of (E)-3-(4-Alkyloxyphenyl)-1-(2-hydroxyphenyl)prop-2-ene-1-one,(E)-3-(4-Alkyloxyphenyl)-1-(4-hydroxyphenyl)prop-2-ene-1-one and their analogues. World J. Chem. 2009;4:9–14.
- Swamy PMG, Agasimundin YS. Synthesis and antimicrobial activity of some novel chalcones containing 3-hydroxy benzofuran. Acta Pharm. Sciencia. 2008;50:197–202.
- Mayekar AN. Int. J. Chem. Synthesis, characterization and antimicrobial study of some new cyclohexenone derivatives. 2010;2:114–123.
- de Carvalho Tavares LC, Johann S, Maria de Almeida Alves TMA, Guerra JC, Maria de Souza-Fagundes EMS, Cisalpino PS, Bortoluzzi AJ, Caramori GF, de Mattos Piccoli RMC, Braibante HTS, Braibante MEF, Pizzolatti MG, Pizzolatti G. Quinolinyl and quinolinyl N-oxide chalcones: synthesis, antifungal and cytotoxic activities. Eur. J. Med. Chem. 2011;46:4448–4456.10.1016/j.ejmech.2011.07.019
- Nakagawa-Goto KN, Lee KH. Anti-AIDS agents 68. The first total synthesis of a unique potent anti-HIV chalcone from genus Desmos. Tetrahedron Lett. 2006;47:8263–8266.10.1016/j.tetlet.2006.09.110
- Arun A, Reddy BSR. In vitro drug release studies of 2-hydroxyethyl acrylate or 2-hydroxypropyl methacrylate-4-{(1E,4E)-5-[4-(acryloyloxy)phenyl]-3-oxopenta-1,4-dienyl}phenyl acrylate copolymer beads. J. Bioact. Compat. Polym. 2003;18:219–300.10.1177/0883911503035385
- Esquivel-Guzmán JA, Zaragoza-Galán G, Ortíz-Palacios J, Rivera E. Synthesis and characterization of novel polymers bearing fluorescein units: thermal and optical properties. Des. Monomers Polym. 2012;15:561–574.10.1080/1385772X.2012.688339
- Vijayanand PS, Kato S, Koyama M, Satokawa S, Kojima T. Co-polymerization of 4-biphenyl methacrylate with methyl methacrylate: synthesis, characterization and determination of monomer reactivity ratios. Des. Monomers Polym. 2007;10:375–388. Retrieved from http://www.jiscjournalarchives.ac.uk/openurl.html?ref=brill/1385772X/pdf/1385772x_v10n4_20070814_s7.pdf.
- Bolard J, Legrand P, Heitz F, Cybulska B. One-sided action of amphotericin B on cholesterol-containing membranes is determined by its self-association in the medium. Biochemistry. 1991;30:5707–5715.10.1021/bi00237a011
- Arun A, Reddy BSR. In vitro drug release studies from the polymeric hydrogels based on HEA and HPMA using 4-{(E)-[(3Z)-3-(4-(acryloyloxy)benzylidene)-2-hexylidene]methyl}phenyl acrylate as a crosslinker. Biomaterials. 2005;26:1185–1193. Retrieved from http://www.sciencedirect.com/science/article/pii/S0142961204003680.
- Sharkawi T, Leyni-Barbaz D, Chikh N, McMullen JN. Evaluation of the in vitro drug release from resorbable biocompatible coatings for vascular stents. J. Bioact. Compat. Polym. 2005;20:153–168.10.1177/0883911505051661
- Gallardo A, Parejo C, San Román J. NSAIDs bound to methacrylic carriers: microstructural characterization and in vitro release analysis. J. Controlled Release. 2001;71:127–140.10.1016/S0168-3659(01)00212-7
- Elvira C, Gallardo A, Lacriox N, Schacht E, Sanroman J. Incorporation of salicylic acid derivatives to hydrophilic copolymer systems with biomedical applications. J. Mater. Sci. Mater. Med. 2001;12:535–542.
- Davaran S, Hanaee J, Khosravi A. Release of 5-amino salicylic acid from acrylic type polymeric prodrugs designed for colon-specific drug delivery. J. Controlled Release. 1999;58:279–287.10.1016/S0168-3659(98)00167-9
- McCormick CL. Controlled activity polymers with pendent metribuzin: effect of structure on hydrolytic release. Ann. N.Y. Acad. Sci. 1985;446:76–92.10.1111/nyas.1985.446.issue-1