Abstract
A quartz crystal microbalance (QCM) electrode coated with Chitosan (Cs) film was used as a sensor for some volatile organic compounds (VOCs) such as aliphatic amines and alcohols. A homogeneous smooth thin films of Cs coating on the QCM electrode was prepared by drop casting method. The frequency shifts (Δf) of the QCM, due to adsorption of the VOCs under investigation, onto the Cs film were measured and were related to the analytes’ concentrations. Calibration curves were obtained with linear relationships between the Δf (Hz) and the analyte concentrations (mg L−1). The sensor showed good sensitivity, reproducibility, and reversibility. The influence of different temperatures on the vapor adsorption, of the VOCs onto the Cs film, was studied.
1. Introduction
Chemically modified quartz crystal microbalance (QCM) has been widely used in toxic gas and organic vapors detection owing to its extreme sensitivity and simplicity. Macrocyclics,[Citation1–4] mono- and multi-layers [Citation5–7], and bio-organic molecules [Citation8,9] have been used as coatings on the QCM electrode to improve its sensitivity and selectivity. It has been reported that in the gas systems, the simple dispersion forces, hydrogen bonding, or dipole–dipole interactions between the sensor coating and the analytes result in an increased signal intensity.
The QCM operation principle is based on the resonant frequency shift (Δf) caused by the adsorption of gas molecules onto the coated-QCM according to Sarerbrey equation.[Citation10](1)
where f0 (Hz) is the natural frequency of the quartz crystal, ρQ is the quartz density (2.649 g cm−3), μQ is the shear modulus (2.947 × 1011 dyne/cm2), and m′ is the mass change per unit area.
Appropriate coating materials on QCM electrode surface have played an important role in the detection of different analytes. The coating of QCM electrode with thin layers of polymers can be carried out by various coating methods e.g. spray coating, spin coating, and drop cast from solution.[Citation11] Recently, we have exploited polyaniline (PANI) coating on the electrode of the QCM as pH,[Citation12] phosphoric acid,[Citation13] chlorinated hydrocarbons, aliphatic amines, and alcohols vapor [Citation14–16] sensors. The sensors showed good reproducibility and reversibility. It has been concluded that the hydrophilicity, dispersion forces, hydrogen bonding, and/or dipole–dipole interactions play an important role to enhance the signal intensity of the sensor.
Chitosan (Cs) is a poly (amino-saccharide) [2-amino-2-deoxy-(1→4)-β-D-glucopyranan; poly (1,4)-β-D glucopyranos-amine]. It bears two types of reactive groups first, the free amino groups on deacetylated units and secondly, the hydroxyl groups on the C3 and C6 carbons of acetylated or deacetylated units. It is soluble in acidic aqueous solution, and forms strong film from solution containing as little as 1 wt.% of acetic acid. Furthermore, Cs has many interesting characteristics including good mechanical strength, good film-forming properties, low cost, and hydrophilicity.[Citation17,18] These properties have led to the diverse applications of Cs in many fields such as the sensor area. Recently, Cs film sensor has been fabricated to detect acetone concentrations in human breath, in order to precisely diagnose diabetes mellitus in patients. Acetone vapor concentration in the breath varies from 0.3 to 0.9 ppm at room temperature (25–30 °C) in normal air. The acetone-based gas sensor has been operated with a high performance demonstrated by good response, recovery, stability and repeatability.[Citation19] Cs has also been used to coat Fe3O4 nanoparticles to prepare iron oxide-based gas sensors.[Citation20] The gas sensing measurements revealed that the Fe3O4/Cs nanocomposite had a better response to H2, CO, C2H5OH, and NH3 as compared to the pristine Fe3O4.[Citation20] The same nanocomposite film deposited onto indium-tin-oxide has been prepared for urea detection and the sensor has shown good sensitivity.[Citation21] The dispersion of carbon nanoparticles into Cs matrix has been successfully used to develop water, toluene, and methyl alcohol vapors sensor. The response of the sensor to different analytes has followed the order: water > methyl alcohol > toluene.[Citation22] An optical micro-electromechanical systems sensor has been fabricated for the detection of catechol using the UV and near UV range.[Citation23] The detection limit of the device is found to be about 0.2 mM for a 10 min reaction time. Dubas et al. [Citation24] have used the layer-by-layer technique to assemble Cs–Nylosan dye films to detect ethyl alcohol content in water. The reported ethyl alcohol content was found to be linear from 10 to 45%. Wen et al. [Citation25] developed an alcohol biosensor which comprises an alcohol oxidase/Cs immobilized membrane. The response of the sensor was defined as the decrease in dissolved O2 concentration and depends linearly on ethyl alcohol concentration between 60 μM and 0.80 mM. Due to the hydrophilicity of Cs, it is intended to coat the QCM electrode with Cs to be used as a gas sensor.
Sensing low concentrations of chemical vapors and volatile organic compounds (VOCs) is an area of great interest in many various applications. Amines vapors are of particular interest since low concentrations of both aliphatic and aromatic amines have toxicological effects.[Citation26,27] In addition, alcohols such as methyl alcohol and ethyl alcohol are volatile organic solvents that are commonly used in many workplaces, laboratories, medicine, food industry, and chemicals industry. The exposure to methyl alcohol vapor for a long time causes some diseases such as eyesight disturbance, nasal mucous membrane, conjunctiva inflammation, nerve disease, and even death.
The present work reports the using of thin Cs films-coated QCM electrode as a sensor for the detection of methylamine, dimethylamine, diethylamine, methyl alcohol, ethyl alcohol, and isopropyl alcohol vapors in air. A simple, rapid, sensitive, and reproducible sensor was developed. The influence of different temperatures on the vapor adsorption onto the Cs film was studied.
2. Experimental
2.1. Reagents and materials
Cs of high M.wt. (~310.000 to ˃375.000 Da) was obtained from (Sigma Aldrich, USA). Methylamine 40% solution (LOBA Chemie, India), dimethylamine 40% solution (Oxford, India), diethylamine 99% (MERCK, Germany), glacial acetic acid 99% analytical grad, methyl alcohol, ethyl alcohol, and isopropyl alcohol (ADWIC, Egypt) were used without further purification.
2.2. QCM measurements
A 5 MHz AT-cut quartz crystal with gold electrodes in both sides was used. The QCM apparatus used for the frequency measurements was described elsewhere.[Citation14] The resonance frequency of the crystal was determined by GW frequency counter, Model GFC-8055G. A schematic illustration of the system is shown in Figure .
The gold-coated QCM electrode was cleaned via its soaking in piranha solution (3:1, H2SO4:30% H2O2) for 5 min and rinsing thoroughly with distilled water. The surface modification of the gold-coated QCM plate was carried out by dropping 0.1 mL Cs solution (4 mg mL−1 of Cs in 2% v/v acetic acid) on the surface of gold electrode of QCM by micro-syringe). The droplet was spread with the tip of the syringe needle to cover the entire gold electrode. The quartz crystal was then put into vacuum desiccator to remove all the entrapped gas bubbles and to form a film. Then the Cs-coated QCM was transferred into an air oven to be dried at 60 °C. The scanning electron microscopy showed that a homogenous smooth film of Cs was formed (Figure ).
The thickness of the film, L (cm) can be determined from the density (ρ) of the Cs films, ρ ≈ 1.37 (g cm−3) [Citation28] using the relation:
(2)
2.3. Procedures
In order to obtain extremely high oscillation capabilities with very low noise, all the experiments were carried out in a hermetical condition with the usage of a shielding box for impeding the magnetic field, and a set of constant potential was supplied for keeping stable voltage.
All measurements were carried out in a double wall vessel with an internal volume of 110 mL at room temperature (25 ± 1 °C). PerkinElmer microliter syringe (PerkinElmer, Australia) was used for analyte injections. The concentration of the injected VOCs in the cell was calculated in mg L−1 using its density, purity percentage, and volume.
After the adsorption of the analyte, the Cs thin film coated the gold electrode of the QCM was exposed to hot dry air (50 °C) to desorb the analyte and recover the electrode. The backshift of the crystal frequency to its initial value was taken as an indication of full desorption.
3. Results and discussion
3.1. The QCM measurements for determination of amines vapors
The Cs film coating on the electrode of QCM was exposed to different concentrations of amines’ vapors. The frequency changes due to the adsorption of amine vapors onto the film as a function of time were recorded.
Figure (a) shows the exposure of Cs film (thickness 2.68 μm) to different concentrations of methylamine vapors. The frequency of the quartz crystal decreased due to the adsorption of the vapors onto the polymer film according to Equation (Equation1(1) ). Δf increased linearly with increasing the concentration of the tested methylamine vapor. Since more methylamine molecules were provided in the test atmosphere, more molecules were expected to be adsorbed onto the Cs film coating on the QCM electrode.
Figure 2. (a) Δf of QCM electrode coated with Cs film exposed to different concentrations of methylamine vapors, (b) calibration curve for the methylamine, (c) Δf of different concentrations of dimethylamine vapors, (d) calibration curves for the dimethylamines, (e) Δf of different concentrations of diethylamine vapors, (f) calibration curve for the diethylamines.
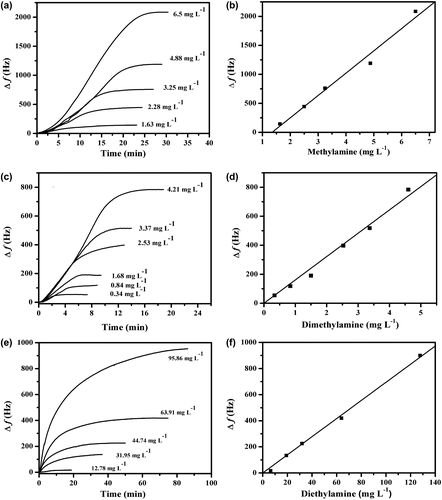
After the equilibrium has been attained, the sensor can be recovered by drying the electrode using hot dry air. The response obtained from the sensor should be linear against the concentration of analyte. Thus, a calibration curve of Δf vs. different concentrations of methylamine vapors ranged from 1.63 to 6.5 mg L−1 was plotted as shown in Figure (b). A linear relationship was obtained with correlation coefficient (R2) and slope (sensitivity) of 0.99 and 384.7 Hz mg−1 L, respectively.
The Cs film coating on QCM electrode was also exposed to different concentrations of dimethylamine and diethylamine vapors. The time dependence of Δf was obtained for different concentrations of vapors as shown in Figure (c) and (e). The same profiles were obtained as reported in the case of methylamine. It is clear from the graphs that as the concentration of the vapor increases, the magnitude of the response increases as well. Calibration curves were constructed by plotting Δf against the concentration of the vapors as shown in Figure (d) and (f) and linear relationships were obtained. The sensitivities and R2 of the Cs film coating on the QCM electrode toward the tested amines vapors were calculated and given in Table . Our results show a higher sensitivity when compared to results of Mirmohseni and Oladegaragoze [Citation29] who used a thin layer of polyvinylpyrrolidone modified QCM for the detection of ammonia and number of short chain aliphatic amines vapors with a sensitivity of 7.5–48.2 Hz mg−1 L. The sensitivity also higher than the results of Ayad and Torad [Citation15] who used PANI base film coating on QCM electrode, as a sensor for a primary aliphatic amines vapors. The sensitivity was found to be 6.4–38 Hz mg−1L.
Table 1. Analytical characteristic parameters for the amine and alcohol vapors using the Cs film coating on the QCM electrode at room temperature (25 ± 1 °C).
The differences in sensitivity of the Cs films toward different analytes can be explained because of the difference of the adsorption sensing mechanism of the amines’ vapors with the protonated amines sites of Cs chains. The adsorption mechanism could be based on the hydrogen-bonding and dipolar interactions [Citation30] between the amines vapor with hydroxyl and protonated amines sites of Cs chains.
It can be shown that methylamine records high Δf than the other amines. The sensitivity of detection is in the order, methylamine > dimethylamine > diethylamine. This is can be explained on the basis that the protonated Cs has hydrophilic properties [Citation18] and the hydrophilicity of the studied amines ranked in the order, methylamine > dimethylamine > diethylamine. Therefore, it is expected that the order of the sensitivity detection will follow the order of the variation in the hydrophilicity of the amines under investigation. Similar results were obtained for the detection of these amines based on PANI base coating on the QCM electrode.[Citation15] However, the role of the electrostatic interaction and dipolar interactions can’t be excluded.
3.2. The QCM measurements for determination of alcohol vapors
A film of Cs coating on the electrode of QCM with a thickness 2.15 μm was exposed to various concentrations of methyl, ethyl, and isopropyl alcohols’ vapors. The frequency of the quartz crystal decreased due to the adsorption of the vapors into the film according to Equation (Equation1(1) ). The equilibrium was obtained after few minutes. The relation between Δf and time was plotted and is shown in Figure (a), (c), and (e) for methyl alcohol, ethyl alcohol, and isopropyl alcohol, respectively. Calibration curves were constructed for determination of methyl, ethyl, and isopropyl alcohols vapors as shown in in Figure (b), (d), and (f). The sensitivities and R2 of different alcohols onto the Cs film coating on the QCM electrode toward the tested alcohol vapors were calculated and are given in Table . It can be seen that the sensitivity of detection are in the range of 4.06–17.3 Hz mg−1 L. It worth mentioning that Ayad and Torad [Citation16] have used PANI salt film coating on QCM electrode for the detection of methyl, ethyl, and isopropyl alcohol vapors, the sensitivity of detection in the range 20–33.5 Hz mg−1 L. Also, Ting et al. [Citation31] have employed calixarene derivatives as adsorptive coating for the QCM electrode as a sensor of ethyl alcohol and reported a sensitivity of 10.53 Hz mg−1 L.
Figure 3. (a) Δf of QCM electrode coated with Cs film exposed to different concentrations of methyl alcohol vapors, (b) calibration curve for the methyl alcohol, (c) Δf of different concentrations of ethyl alcohol vapors, (d) calibration curves for the ethyl alcohols, (e) Δf of different concentrations of isopropyl alcohol vapors, (f) calibration curve for the isopropyl alcohol.
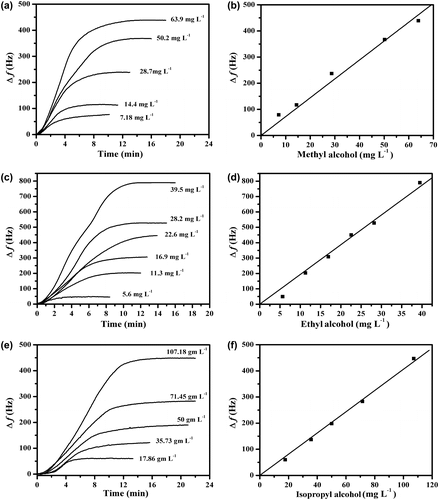
It can be shown that the sensitivity of detection is in the order, ethyl alcohol > methyl alcohol > isopropyl alcohol. The interaction between the protonated Cs film with both methyl alcohol and ethyl alcohol is electrostatic interaction through hydrogen bonding between hydroxyl and protonated amines groups of the Cs polymer chains and oxygen atoms of the alcohol.[Citation32] Since ethyl alcohol is more basic than methyl alcohol due to the differences between the inductive effect of methyl and ethyl groups, it would be expected that ethyl alcohol is adsorbed onto the Cs film more than methyl alcohol. The lowest sensitivity of isopropyl alcohol vapor can be explained on the basis of its steric hindrance which prevents isopropyl alcohol molecules from further adsorption onto the Cs film coating on the QCM electrode.
3.3. Effect of temperature on Cs film on the adsorption of methylamine
The influence of various temperatures on the sensitivity of the sensor is shown in Figure . Δf of the sensor was recorded at various temperatures using 3.25 mg L−1 of methylamine as presented in Figure . It is observed that there is a significant change in frequency shift with temperature change in the range of 20–50 °C. As the temperature increases, the amount of adsorbed vapor decreases. The adsorption of methylamine vapor at 20 and 30 °C are approximately the same, however as the temperatures increases, the amount of the adsorbed vapor decreases. The temperature also has an effect on the rate of adsorption.
The rates of adsorption of the vapor can then be determined at different temperatures using the pseudo-first-order rate constant (k) following the equation:(3)
where Δft and Δf∞ refer to the adsorbate weight uptake at time t and at equilibrium, respectively. A graph of ln (1 – Δft/Δf∞) against t should be a straight line of gradient k.
The plot of against response time t (sec) of Cs film for a concentration of 3.25 mg L−1 of methylamine is shown in Figure . It is clear that the vapor uptakes at different temperatures into the film followed the linear driving force mass transfer kinetic model.[Citation33] The k values were determined from the gradient of the kinetic plot and are listed in Table .
Figure 5. Variation of ln (1 – Δft/Δf∞) against time for the adsorption of 3.25 mg L−1 methylamine at different temperatures.
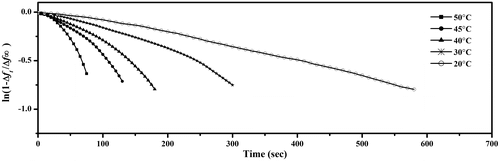
Table 2. Effect of temperature on the sensitivity, the rate of adsorption and the frequency changes of Cs film towards 3.25 mg L−1 methylamine.
3.4. Reproducibility and reversibility of the sensor
The sensor based on the Cs film showed good reproducibility and reversibility. Figure shows the Δf of the Cs film of thickness 1.06 μm when exposed to 2.47 mg L−1 of methylamine till the steady state and the full desorption of the crystal was obtained using a hot dry air. The presence of about 3–5 Hz in each analyte injection is considered to be stability in the quartz crystal electrode. The experiment was repeated three times to ensure the complete reversibility of the sensor. This behavior confirms that the sensing interaction between Cs coating and analytes is physical adsorption.[Citation34] The relative standard deviation was calculated and the value of 4.7% was obtained, which indicates that the sensor performs in a reproducible manner.
4. Conclusion
The Cs film was prepared by drop casting from its solution. The morphology of the Cs film showed smooth and homogenous film structure. The Cs film coating on QCM electrode was used to detect some VOCs. Sensitivity of protonated Cs film towards methylamine vapor among the other amines under investigation showed a large sensitivity due to the electrostatic interaction and the hydrophilicity of the amines and Cs film. Moreover, the Cs coating on the QCM electrode can be used to determine aliphatic alcohols. It was found that the electrostatic interaction and steric hindrance play an important role in the sensing process. Effect of temperature on the adsorption of methylamine into the Cs film was studied and the rate constants of adsorption process were calculated. Regeneration process of the film showed good reproducibility.
References
- Schierbaum KD, Gerlach A, Haug M, Göpel W. Selective detection of organic molecules with polymers and supramolecular compounds: application of capacitance, quartz microbalance and calorimetric transducers. Sens. Actuators A. 1992;31:130–137.10.1016/0924-4247(92)80092-H
- Nell P, Dalcanale E, Faglia G, Sberveglieri G, Soncini P. Cavitands as selective materials for QMB sensors for nitrobenzene and other aromatic vapours. Sens. Actuators B. 1993;13:302–304.10.1016/0925-4005(93)85386-O
- Battenberg A, Breidt VF, Vahrenkamp H. Synthesis and test of organometallic materials as sensitive layers on quartz microbalance devices. Sens. Actuators B. 1996;30:29–34.10.1016/0925-4005(95)01731-A
- Dickert FL, Schuster O. Supramolecular detection of solvent vapours with calixarenes: mass-sensitive sensors, molecular mechanics and BET studies. Mikrochim. Acta. 1995;119:55–62.10.1007/BF01244854
- Ebato H, Gentry CA, Herron JN, Muller W, Okahata Y, Ringsdort H, Suci PA. Investigation of specific binding of antifluorescyl antibody and fab to fluorescein lipids in Langmuir–Blodgett deposited films using quartz crystal microbalance methodology. Anal. Chem. 1994;66:1683–1689.10.1021/ac00082a014
- Yang HC, Dermody DL, Xu C, Ricco AJ, Crooks RM. Molecular interactions between organized, surface-confined monolayers and vapor-phase probe molecules. 8. Reactions between acid-terminated self-assembled monolayers and vapor phase bases. Langmuir. 1996;12:726–735.10.1021/la9505226
- Zhou R, Hierlemann A, Schierbaum KD, Geckeler KE, Gopel W. Detection of organic solvents with reliable chemical sensors based on cellulose derivatives. Sens. Actuators B. 1995;24:443–447.10.1016/0925-4005(95)85099-6
- Yokoyama K, Ikebukuro K, Tamiya E, Karube I, Ichiki N, Arikawa Y. Highly sensitive quartz crystal immunosensors for multisample detection of herbicides. Anal. Chim. Acta. 1995;304:139–145.10.1016/0003-2670(94)00579-B
- Nicolinia C, Adami M, Dubrovskya T, Erokhinb V, Faccia P, Paschkevitsch P, Sartore M. High-sensitivity biosensor based on LB technology and on nanogravimetry. Sens. Actuators B. 1995;24:121–128.10.1016/0925-4005(95)85026-0
- Sauerbrey G. Verwendung von Schwingquarzen zur Wägung dünner. Schichten und Microwägung [The use of quartz oscillators for weighing thin layers and for microweighing]. Z. Phys. 1959;155:206–222.10.1007/BF01337937
- Komolov A, Schaumburg K, Møller PJ, Monakhov V. Characterization of conducting molecular films on Silicon: AFM, AES, XPS and surface photovoltage. Appl. Surf. Sci. 1999;142:591–597.10.1016/S0169-4332(98)00924-6
- Ayad MM, Salahuddin NA, Abou-Seif AK, Al-Ghayesh MO. pH sensor based on polyaniline and aniline-anthranilic acid copolymer films using quartz crystal microbalance and electronic absorption spectroscopy. Polym. Adv. Technol. 2008;19:1142–1148.10.1002/pat.v19:8
- Ayad MM, Salahuddin NA, Alghaysh MO, Issa RM. Phosphoric acid and pH sensors based on polyaniline films. Curr. Appl. Phys. 2010;10:235–240.10.1016/j.cap.2009.05.030
- Ayad MM, El-Hefnawey G, Torad NL. Chlorinated hydrocarbon vapors sensor using polyaniline coated quartz crystal microbalance sensor. Sens. Actuators B. 2008;134:887–894.10.1016/j.snb.2008.06.058
- Ayad MM, Torad NL. Quartz crystal microbalance sensor for detection of aliphatic amines vapours. Sens. Actuators B. 2010;147:481–487.10.1016/j.snb.2010.03.064
- Ayad MM, Torad NL. Alcohol vapours sensor based on thin polyaniline salt film and quartz crystal microbalance. Talanta. 2009;78:1280–1285.10.1016/j.talanta.2009.01.053
- Mucha M, Wańkowicz K, Balcerzak J. Analysis of water adsorption on chitosan and its blends with hydroxypropylcellulose. e-Polymers. 2007;7:181–190.
- Wang B, Zhang J, Cheng G, Dong S. Amperometric enzyme electrode for the determination of hydrogen peroxide based on sol–gel/hydrogel composite film. Anal. Chim. Acta. 2000;407:111–118.10.1016/S0003-2670(99)00778-3
- Nasution TI, Nainggolan I, Hutagalung SD, Ahmad KR, Ahmad ZA. The sensing mechanism and detection of low concentration acetone using chitosan-based sensors. Sens. Actuators B. 2013;177:522–528.10.1016/j.snb.2012.11.063
- Cuonga ND, Hoa TT, Khieu DQ, Lamd TD, Hoa ND, Hieu NV. Synthesis, characterization, and comparative gas-sensing properties of Fe2O3 prepared from Fe3O4 and Fe3O4-chitosan. J. Alloys Comp. 2012;523:120–126.10.1016/j.jallcom.2012.01.117
- Kaushik A, Solanki PR, Ansari AA, Sumanaa G, Ahmad S, Malhotra BD. Iron oxide-chitosan nanobiocomposite for urea sensor. Sens. Actuators B. 2009;138:572–580.10.1016/j.snb.2009.02.005
- Bouvree A. Conductive Polymer nano-bioComposites (CPC): chitosan-carbon nanoparticle a good candidate to design polar vapour sensors. Sens. Actuators B. 2009;138:138–147.10.1016/j.snb.2009.02.022
- Dykstra P, Hao J, Koev ST, Payne GF, Yu L, Ghodssi R. An optical MEMS sensor utilizing a chitosan film for catechol detection. Sens. Actuators B. 2009;138:64–70.10.1016/j.snb.2009.01.065
- Dubas ST, Iamsamai C, Potiyaraj P. Optical alcohol sensor based on dye–chitosan polyelectrolyte multilayers. Sens. Actuators B. 2006;113:370–375.10.1016/j.snb.2005.03.032
- Wen G, Zhang Y, Shuan S, Dong C, Choi MMF. Application of a biosensor for monitoring of ethanol. Biosens. Bioelectron. 2007;23:121–129.10.1016/j.bios.2007.03.024
- Page EH, Cook CK, Hater MA, Mueller CA, Grote AA, Mortimer VD. Visual and ocular changes associated with exposure to two tertiary amines. Occup. Environ. Med. 2003;60:69–75.10.1136/oem.60.1.69
- Greim H, Bury D, Klimisch H-J, Oeben-Negele M, Ziegler-Skylakakis K. Toxicity of aliphatic amines: structure-activity relationship. Chemosphere. 1998;36:271–295.10.1016/S0045-6535(97)00365-2
- Jeremy LC. Investigation of film forming properties of β-chitosan from jumbo squid pens (Dosidicus gigas) and improvement of water solubility of β-chitosan. Corvallis, OR: Oregon State University; 2012.
- Mirmohseni A, Oladegaragoze A. Construction of a sensor for determination of ammonia and aliphatic amines using polyvinylpyrrolidone coated quartz crystal microbalance. Sens. Actuators B. 2003;89:164–172.10.1016/S0925-4005(02)00459-8
- Li G, Zheng J, Ma X, Sun Y, Fu J, Wu G. Development of QCM trimethylamine sensor based on water soluble polyaniline. Sensors. 2007;7:2378–2388.10.3390/s7102378
- Ting Z, Zhong C, Yun-Lin D, Ting-Ting C, Jing-Lin H, Lei-Tao X, Shu L. Sensing mechanism and application for recognition of ethanol by calixarene supramolecules based on hydrogen bonds interaction. Chem. J. Chinese U. 2013;34:1339–1346.
- Cagri A, Ustunol Z, Ryser ET. Antimicrobial edible films and coatings: a review. J. Food Prot. 2004;67:833–848.
- Chagger HK, Nadaji FE, Sykes ML, Thomas KM. Kinetics of adsorption and diffusional characteristics of carbon molecular sieves. Carbon. 1995;33:1405–1411.10.1016/0008-6223(95)00087-T
- Mirmohseni A, Oladegaragoze A. Determination of chlorinated aliphatic hydrocarbons in air using a polymer coated quartz crystal microbalance sensor. Sens. Actuators B. 2004;102:261–270.10.1016/j.snb.2004.04.027