Abstract
A series of thiophene-based chiral small bandgap π-conjugated polymers was successfully synthesized. Optical properties of the polymers were investigated by using UV–vis, photoluminescence (PL), circular dichroism (CD) spectroscopies, and specific rotation measurements. Especially, the isothianaphthene–thiophene based copolymers display 10–20 nm longer absorption maximum than those of thiophene–thiophene copolymers in the UV–vis. PL measurements demonstrate that the polymer exhibit light emission. CD spectroscopy measurements reveal helical π-stacking structure of the polymers.
1. Introduction
Half a century ago, Rosalind Franklin first obtained quite a beautiful X-ray diffraction pattern of DNA.[Citation1] The double helical structure of DNA was evaluated.[Citation2] The discovery opened avenues to researches on molecular genetics and biological chemistry of helical natural polymers, such as RNA, sugars, and proteins. Synthetic macromolecules also show helical structures. Isotactic polyethylene produced by using the Ziegler–Natta catalyst forms 3/1 turn helical structures.[Citation3] Especially, chiral π-conjugated polymers form helical structure. Chiroptical activities derived from π-conjugated chromophores are observed for the chiral π-conjugated polymers. We have synthesized chiroptically active polymers based on consideration for biomimetic technologies to produce structure organizing polymers having higher order forms.[Citation4]
Small-bandgap polymers have been paid attention from viewpoint of applications for polymer light emitting diodes, photovoltaics, and optical modulators because high sensitivity for light and electric field. Fused-type aromatic groups have been employed for construction of low-bandgap polymers. Especially, isothianaphthene (ITN) is one of the most simple and promising fused-type block for conjugated polymers.[Citation5] To synthesize aromatic type conjugated polymers, polycondensation reactions have been performed, such as Miyaura–Suzuki coupling reaction, Migita–Kosugi–Stille reaction, and Mizoroki–Heck reaction. Recently, an effective direct polyarylation method was developed.[Citation6] Synthesis of achiral aromatic conjugated polymers with achiral flexible alkyl chain showing amphotropic liquid crystallinity (both thermotropic and Iyotropic liquid crystallinity) was reported by Kaeriyama et al. [Citation7]. Main chain type chiral aromatic polymers have been considered after discussion of one of the authors (Goto) with him in Nagoya, Japan (1998).
In this study, we synthesized thiophene–thiophene (Th–Th) and isothianaphthene-thiophene (ITN–Th)-based chiral π-conjugated copolymers as synthetic chiral polymers. The authors had previously synthesized chiral aromatic compounds using the Mitsunobu reactions.[Citation8–10] The polymers thus obtained exhibit light emission. Circular dichroism (CD) absorption spectroscopy measurements demonstrated helical π-stacking structure of the polymers.
2. Synthesis and methods
Dibromothiophene acetic acid and (S)-1,1,1-trifuluoro-undecan-2-ol were coupled in CH2Cl2 by the esterification with an aid of N,N-dimethylaminopyridine (DMAP) and dicyclohexylcarbodiimide (DCC) to obtain the chiral monomer (Mono 2), as shown in Scheme . Molecular structures of the chiral monomers were confirmed by nuclear magnetic resonance (NMR) spectroscopy measurements in CDCl3 solution. 2,5-Di(trimethylstannyl)thiophene (TMSn2Th),[Citation11] 5,5′-bis(trimethylstannyl)-2,2′-bithiophene (TMSn2BTh), and 1,3-di(trimethylstannyl)isothianaphthene (TMSn2ITN) [Citation12] were prepared by lithiation followed by a reaction with trimethylstannyl chloride.
Migita–Kosugi–Stille type polycondensation with an aid of [Pd(0)] catalyst was carried out between the chiral monomer (Mono 1 or Mono 2) and TMSn2Th, TMSn2BTh, or TMSn2ITN in dimethylformamide (DMF) to yield polymers. The polymers were obtained with good yields. Optical rotation of monomers and polymers was measured in tetrahydrofuran (THF) solution. All the monomers and polymers showed negative optical rotation values at sodium D-line (589.29 nm). Mono 1: [α]D = −0.95° (Scheme ).
2.1. Synthesis of (−)-2,5-dibromo-thiophene-3-carboxylic acid 1-trifluoromethyl-decyl ester (Mono 2)
A solution of 2,5-dibromothiophene-3-acetic acid (0.840 g, 3.13 mmol), (S)-1,1,1-trifuluoro-undecan-2-ol (0.776 g, 3.43 mmol), and DMAP (0.639 g, 5.23 mmol) in CH2Cl2 (10 mL). The solution was cooled to 0 °C and stirred under N2 flow for 20 min prior to addition of DCC. Then, DCC (0.661 g, 3.20 mmol) was dissolved in 2 mL of CH2Cl2, the solution was added to the stirring mixture at 0 °C. The reaction was conducted at room temperature under N2 flow for 24 h. After evaporation of the solvent, the reactant was washed with water thoroughly. The organic layer was extracted with dichloromethane. After the evaporation, the crude product was purified with column chromatography (silica gel, dichloromethane/hexane = 1/1) to afford mono 2 as a pale-yellow oil (1.10 g, Y = 74%). 1H NMR of (−)-Mono 2 in CDCl3 is displayed in Figure . The sextet signal is observed at δ 5.43−5.49 ppm from TMS due to a proton at the stereogenic center. 1H NMR (400 MHz, δ from TMS(ppm), CDCl3): δ 0.87 (t, 3H, –(CH2)7–CH3–, J = 11 Hz), 1.25–1.38 (m, 14H, –(CH2)7–CH3–), 1.83–1.87 (m, 2H, –CH2–(CH2)7–), 5.43–5.49 (sext, 1H, –CH–CF3–), 7.37 (s, 1H, 3H(thiophene)). [α]D = 2.3°.
2.2. Synthesis of (−)-P(ITN-CH3)
A solution of Mono 1 (45 mg, 0.115 mmol), and Pd(PPh3)4 (10 mg, 0.009 mmol) in DMF (0.5 mL) was stirred. Then, TMSn2ITN (42.3 mg, 0.0915 mmol) was added to the solution, and refluxed under argon atmosphere for 24 h. The crude polymer was washed with a large volume of methanol. Filtration followed by drying in vacuum to afford a desired polymer abbreviated as (−)-P(ITN-CH3) (dark-red solid, 36.1 mg, Y ≈ 100%).
2.3. Synthesis of (−)-P(ITN-CF3)
A solution of Mono 2 (16.3 mg, 0.033 mmol), TMSn2ITN (12 mg, 0.026 mmol), and [Pd(PPh3)4] (3.6 mg, 0.003 mmol) in DMF (1 mL) was refluxed under an argon atmosphere. After 10 days, the solution was poured into a large volume of methanol, and filtered followed by drying in vacuum to yield dark-red solid (11.7 mg, Y = 92%). The polymer thus obtained is abbreviated as (−)-P(ITN-CF3).
2.4. Synthesis of (−)-P(1T-CF3)
A solution of Mono 2 (76 mg, 0.153 mmol), TMSn2Th (55 mg, 0.133 mmol), and Pd(PPh3)4 (12 mg, 0.009 mmol) in DMF (0.5 mL) was refluxed. After 10 days, the solution was poured into a large volume of methanol and filtered, followed by drying in vacuum to yield a dark-red solid (69 mg, Y ≈ 100%). The polymer thus obtained is abbreviated as (−)-P(1T-CF3).
2.5. Synthesis of (−)-P(2T-CF3)
A solution of Mono 2 (108 mg, 0.219 mmol), TMSn2BTh (57.3 mg, 0.112 mmol), and Pd(PPh3)4 (19 mg, 0.013 mmol) in DMF (1.5 mL) solution was refluxed. After 10 days, the solution was poured into a large volume of methanol and filtered, followed by drying in vacuum to yield a dark-red solid (63 mg, Y ≈ 100%). The polymer thus obtained is abbreviated as (−)-P(2T-CF3).
3. Measurements results and discussion
3.1. GPC measurements
Polymers were thus successfully synthesized in good yields (~100%). Gel permeation chromatography (GPC) measurement results are summarized in Table . Number-average molecular (Mn) weights of the polymers are in the range of 1800−2200 against the polystyrene standard. Weight-average-molecular (Mw) weights of the polymers are in the range of 2400−3800.
Table 1. Copolymerization results.
3.2. Infrared measurements
Infrared (IR) absorption spectra of Mono 2 and the polymers were obtained with the KBr pellet method (Figures and ). (−)-Mono 2 shows absorption bands at 2963–2864 cm−1 attributable to νCH2 and νCH3 of the methylene groups. An intense absorption band corresponding to νC=O as an ester stretching is observed at 1738 cm−1. The absorption bands at 1520 and 1426 cm−1 are due to C=C stretching of the thiophene ring. Characteristic absorption at 1226 cm−1 is C–F stretching. The absorption due to CH out-of-plane bending of thiophene is observed at 766 cm−1.
3.3. UV–vis absorption spectroscopy
UV–vis absorption spectroscopy measurements of the polymers were carried out in chloroform solution and film state (Figures ). Results are summarized in Table . The polymers show absorption maximum in the visible range due to π−π* transition of the main chain. The ITN–Th-based polymers ((−)-P(ITN-CH3), (−)-P(ITN-CF3)) display 10−20 nm longer absorption maximum than those of thiophene-based polymers ((−)-P(1T-CH3), (−)-P(2T-CF3)). This red shift is obviously due to the planar structure of ITN skeleton. The fused ring attached to the thiophene ring provides an expanded conjugated system which means ITN–Th-based polymers can absorb lights at longer wavelength. Meanwhile, the absorption onset of the ITN–Th-based polymers also appears at longer wavelength than those of thiophene-based polymers (Figure ). Figures and show the absorption spectra of P(ITN-CF3) and P(2T-CF3), respectively, in film state. Compared with ITN–Th-based polymers, thiophene-based polymers showed a larger red shift of maximum absorption wavelength in the film state than in solution. This can be attributed to the good film forming properties of linear-shaped thiophene-based polymers. Bandgap of polymers calculated from the onset of UV–vis spectra are summarized in Table . This series of polymers show bandgap value around 2 eV.
Figure 5. UV–vis spectra of P(ITN-CF3) (cast film and solution in chloroform solution) and PL spectra in chloroform solution (UV–vis absorption onset: in CH3Cl(614 nm); Film: (620 nm). Excitation wavelength: 449 nm).
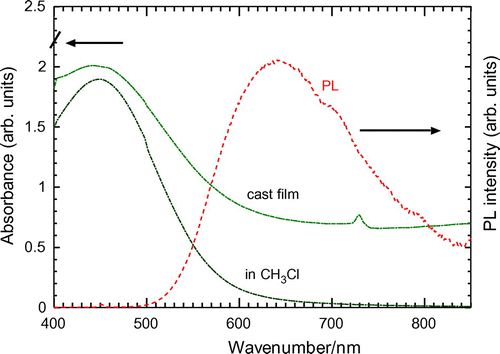
Figure 6. UV–vis spectra of P(2T-CF3) (cast film and solution in chloroform solution) and PL spectra in chloroform solution. (UV–vis absorption onset: in CH3Cl(585 nm); Film: (636 nm). Excitation wavelength: in CH3Cl(437 nm); Film: (481 nm)).
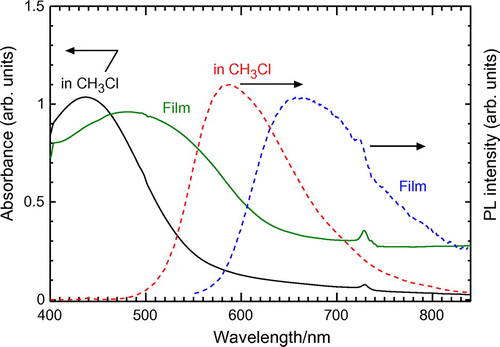
Table 2. Maximum absorption wavelengths (λmax), absorption onset wavelengths (λonset), and maximum emission wavelengths (λem) of the polymers in chloroform solution and in the film state.
3.4. Photoluminescence measurements
Figures and show photoluminescence (PL) spectra of (−)-P(ITN-CF3) and (−)-P(2T-CF3). The PL results are summarized in Table . The ITN-Th- based polymers ((−)-P(ITN-CF3, (−)-P(ITN-CH3) exhibits no PL in the film state. This may be due to the fact that the ITN block has good aggregation property and the ITN-Th based films result in photochemical quenching in the PL measurements. Here, the emission energy from excitation can be exhausted for heat emission from the polymer. However, (−)-P(ITN-CF3) and (−)-P(ITN-CH3) in the CHCl3 solution display PL in the long wavelength compared to (−)-P(1T-CF3) and (−)-P(2T-CF3).
3.5. CD absorption spectroscopy
CD absorption spectrum for a cast film of (−)-P(1T-CF3) is displayed in Figure . This polymer shows split-type cotton effect. The signal cross point at zero (447 nm) corresponds to peak top of the absorption spectra. This can be Davydov split type spectral form in the CD. In this case, plural chromophores in chiral environment exist for showing the split-type spectral form.[Citation13] Other polymers in this series show no characteristic signs in the CD measurements because of weak optical activity, although they show negative optical rotations. The characteristic chiroptical activity of (−)-P(1T-CF3) can be derived from 3-dimensional chiral aggregation structure.[Citation14] Figure displays plausible structure of chiral π-stacking (a) and irradiation of circular polarized light. The polymer showing split-type CD forms such a chiral aggregation. The inter-chain electronic communication via one-handed helical π-stacking allows chiroptical activity of the polymer.
Figure 8. (a) Plausible structure of chiral aggregation based on π-stacking and (b) irradiation of circular polarized light.
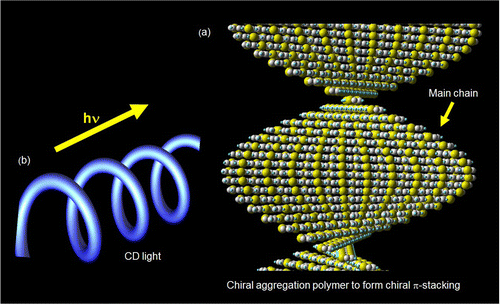
4. Conclusions
A series of aromatic type optically active conjugated polymers were prepared by Migita–Kosugi–Stille polycondensation reaction with good yield. Optical properties of the polymers were characterized by UV–vis absorption, optical PL, optical rotation, and CD spectroscopy measurements. This is the first result of production of ITN-Th-based polymer with optical active substituent.
4.1. Chemicals
DCC (Tokyo Chemicals (TCI)), DMAP (TCI), ((S)-1,1,1-trifuluoro-undecan-2-ol (Daikin, Japan), and 2-octanol (Tokyo Chemicals (TCI), Japan) were used as received. DMF and THF were purified by distillation prior to use. 2,5-dibromo-thiophene-3-carboxylic acid 1-methyl-decyl ester (Mono 1) was prepared by the method based on the coupling reaction between aromatic group and chiral alcohols.
4.2. Instrument
NMR measurements (δ from TMS (tetramethyl silane as an internal standard, ppm, in CDCl3) were carried out using a JNM-ECS 400 NMR spectrometer (JEOL, Japan). IR absorption spectra were obtained with a JASCO FTIR 550 spectrometer. Molecular weights of the polymer were estimated with GPC (5 mm PLGEL MIXED-D column, Polymer Laboratories) eluted with THF by polystyrene standard calibration. Fluorescence spectra were recorded on a Hitachi High-Technologies F-4500. UV–vis and fluorescence spectra of the polymers were obtained at room temperature. Optical rotation of the compounds was measured by using a P-1010 Polarimeter (JASCO). CD spectroscopy measurements were carried out using a J-700 spectrometer (JASCO).
Acknowledgments
Prof. H. Goto (Asst. Prof., at that time, in 1998) is thankful to Prof. K. Kaeriyama (Kyoto Institute of Technology) on the fruitful discussion about the aromatic type conjugated polymers on the occasion of the 47th The Society of Polymer Science, Japan (SPSJ) Symposium on Macromolecules (1998). He kindly gifted a reprint of ref. 7 to Goto, later. The analysis of the PL and the NMR was carried out with a F-4500 (Hitachi) and a JNM-ECS 400 (JEOL), respectively, at University of Tsukuba and the OPEN FACILITY, Research Facility Center for Science and Technology, University of Tsukuba. We thank Engineering Workshop of University of Tsukuba for glasswork. This work was supported by Grant-in-Aid for Scientific Research from the Ministry of Education, Culture, Sports, Science and Technology of Japan (grant #25410218, H. Goto). The early stage of this work was supported by Grant-in-Aid for Scientific Research from the Ministry of Education, Culture, Sports, Science and Technology of Japan (grant #10750642 in 1998−1999, H. Goto).
Additional information
Funding
Funding
References
- Franklin RE, Gosling RG. Molecular configuration in sodium thymonucleate. Nature. 1953;171:740–741.10.1038/171740a0
- Watson JD, Crick FHC. A structure for deoxyribose nucleic acid. Nature. 1953;171:737–738.10.1038/171737a0
- Otsu, T. Chemistry of polymer synthesis. Kyoto: Kagaku-Dojin Publishing; 1968. ( in Japanese).
- Goto H. Doping-dedoping-driven optic effect of π-conjugated polymers prepared in cholesteric-liquid-crystal electrolytes. Phy. Rev. Lett. 2007;98:253901.10.1103/PhysRevLett.98.253901
- Innami Y, Kawashima H, Kiebooms RHL, Aizawa H, Matsuishi K, Goto H. Synthesis and properties of poly(isothianaphthene methine)s with chiral alkyl chain. Materials. 2012;5:317–326.10.3390/ma5020317
- Kuwabara J, Nohara Y, Choi SJ, Fujinami Y, Lu W, Yoshimura K, Oguma J, Suenobu K, Kanbara T. Direct arylation polycondensation for the synthesis of bithiophene-based alternating copolymers. Polym. Chem. 2013;4:947–953.10.1039/c2py20917a
- Kaeriyama K, Kouyama S, Sekita M, Nakayama T, Tsukahara Y. Preparation of amphotropic liquid crystalline poly(alkoxycarbonylphenylene)s. Macro. Rapid Commun. 1999;20:50–54.10.1002/(ISSN)1521-3927
- Dong J, Kawabata K, Goto H. Synthesis and properties of aelf-alignable conjugated polymers. J. Mater. Chem. C. 2015;3:2024–2032.
- Mitsunobu O. The use of diethyl azodicarboxylate and triphenylphosphine in synthesis and transformation of natural products. Synthesis. 1981;1981:1–28.10.1055/s-1981-29317
- Ohkawa S, Yang F, Kawabata K, Goto H. Synthesis of polymers from chiral monomers in chiral liquid crystals. J. App. Polym. Sci. 2013;128:3586–3591.10.1002/app.v128.6
- Seitz D, Lee SH, Hanson RN, Bottaro JC. Synthesis and reactivity of the 2,5-bis(trimethylstannyl) derivatives of thiophene and furan. Synth. Commun. 1983;13:121–128.
- Kawabata. K; Goto, H. Synthesis and optical properties of 1,1-binaphthyl-thiophene alternating copolymers with main chain chirality. J. Mater. Chem. 2012;22:23514–23524.10.1039/c2jm35594a
- Goto H. Asymmetric polymerisation in liquid crystals and resultant electro-chiroptical effect: structure organising polymerisation and chiral charge carrier “chiralion”. IOP Conf. Ser.: Mater. Sci. Eng. 2014;54: 012013.10.1088/1757-899X/54/1/012013
- Langeveld-Voss BMW, Janssen RAJ, Meijer EW. On the origin of optical activity in polythiophenes. J. Mol. Struct. 2000;521:285–301.10.1016/S0022-2860(99)00444-5