Abstract
The monomer 2-{5-[4-((4-nitrophenyl)diazenyl)phenyl]-1,3,4-oxadiazol-2-ylthio}ethyl acrylate (NDPOE-acrylate) was synthesized in five steps and then copolymerized with N-isopropylacrylamide, leading to a dual pH and temperature responsive polymeric sensor. Obtained copolymer exhibited the lower critical solution temperature only in acidic medium (pH 2) as 32 °C. The UV–vis and fluorescence of copolymer was investigated at different pH values in various temperatures. The UV–vis of copolymer are independent to temperature above pH 4. The copolymer exhibited sensitive fluorescence change towards pH between 2 and 10 at different temperatures.

1. Introduction
The design and development of ‘smart’ functional materials, which have a wide range of applications in various fields, such as DNA-sequence detection,[Citation1,2] biotechnology,[Citation3,4] drug delivery,[Citation5–7] organic analysis,[Citation8] particle transport,[Citation9] thermometers,[Citation10–12] bar codes [Citation13] and optical imaging,[Citation14–16] has gained a great deal of attention in the last years.
Recently, dual or multiple stimuli sensors have been reported, that respond simultaneously and independently to different stimuli, such as oxygen and temperature,[Citation17–19] oxygen and carbon dioxide,[Citation20,21] oxygen and pH,[Citation22–25] pressure and temperature,[Citation26] oxygen, temperature and pH,[Citation27] glucose and temperature,[Citation28] pH and temperature,[Citation29–36] and metal ions and temperature.[Citation37] Among these, stimuli-responsive polymers play an important role by changing their structure and physical properties in response to changes in the environment. These stimuli-responsive polymers are prepared by combination of a responsive polymer phase transition (coil-to-globule) with a solvatochromic dye.[Citation38] It is known that poly(N-isopropylacrylamide) (PNIPAM) is one of the most important water-soluble polymers, which exhibits a heat-induced phase transition from coiled to globular state in an aqueous solution in response to temperature changes across a lower critical solution temperature (LCST) at 32 °C,[Citation39] by expanded conformation in water below the LCST due to strong hydration, and changes to compact forms above the LCST by dehydration.[Citation40,41]
Continuing our works on heterocyclic chemistry,[Citation42–45] herein, we wish to report a new heterocyclic pH-responsive dye based on diazenylphenyl-1,3,4-oxadiazol moiety and its use in a novel water-soluble polymeric sensor, which responds to both pH and temperature.
2. Experimental
2.1. Material and methods
All chemicals were purchased from Merck and Sigma–Aldrich and used without any further purification. Solvents were used as received from commercial suppliers. Used buffers were prepared as follows: pH 2 (13 mL of 0.2 M HCl + 50 mL of 0.2 M KCl), pH 4 (0.1 M AcOH), pH (58.2 mL of 0.1 M NaOH + 100 mL of 0.1 M KH2PO4), pH 10 (21.4 mL of 0.1 M NaOH + 100 mL of 0.05 M NaHCO3), and pH 12 (12 mL of 0.2 M NaOH + 50 mL of 0.2 M KCl). NMR spectra were recorded using a Bruker instrument at 400 MHz for proton and at 100 MHz for carbon nuclei in CDCl3 or DMSO-d6. FT-IR spectra were measured using a Perkin–Elmer 781 spectrometer. UV–vis spectra were recorded using Shimadzu UV-1800 spectrophotometer, and fluorescence spectra were measured using JASCO-FP-750, Rev. 1.00 fluorometer.
2.2. Synthesis of NDPOE-acrylate monomer
2.2.1. Synthesis of 5-phenyl-1,3,4-oxadiazol-2-thiol
To a solution of benzoic acid hydrazide (1.34 mmol) in 6–7 mL EtOH, 0.1 g of KOH in 2 mL of water and 0.3 mL of CS2 were added, and the resulting mixture was refluxed for 4 h. After completion of the reaction, monitored by TLC using EtOAc/n-hexane (3/7) as eluent, EtOH was evaporated under reduced pressure, and then, water (4 mL) was added and acidified with a dilute aq. HCl to pH 5. The white precipitate was collected by filtration. Yield of 5-phenyl-1,3,4-oxadiazol-2-thiol (POT) was 76%, and the mp was measured as 211–213 °C.[Citation46]
2.2.2. Synthesis of 5-{4-[(4-nitrophenyl)diazenyl]phenyl}-1,3,4-oxadiazole-2-thiol
Cooled solution of sodium nitrite (2 mmol) in 1.5 mL water was slowly added to ice bath-cooled solution of 4-nitroaniline (2.2 mmol) in 9 mL water and 4 mL HCl (Conc.) and stirred for 5 min at the same temperature. Then, POT (2 mmol) was dissolved in a solution of 0.6 g NaOH in 6 mL water and cooled to 0–5 °C using ice bath. The POT solution was slowly added to above solution during 5 min and then stirred for additional 10 min in an ice bath. The mixture was filtered for removal of dirty solid, and the filtrate was left to precipitate a yellow solid. 48% yield; mp: 223–224.5 °C; FT-IR (KBr) ν = 3269 (C–H), 2401 (S–H), 1596 (C=C), 1517, 1334 (NO2), 1404 (N=N), 1254 (C–O) cm−1; 1H NMR (DMSO-d6) δ = 13.54 (s, 1H, SH), 8.32 (m, 4H, CHAr), 7.84 (d, J = 7.6 Hz, 2H, CHAr), 7.32 (d, J = 7.6 Hz, 2H, CHAr) ppm.
2.2.3. Synthesis of 2-{5-[4-((4-nitrophenyl)diazenyl)phenyl]-1,3,4-oxadiazol-2-ylthio}ethyl acrylate (NDPOE-acrylate)
2-Chloroethanol (4.5 mmol) and 0.63 g KOH were added to a solution of 5-{4-[(4-nitrophenyl)diazenyl]phenyl}-1,3,4-oxadiazole-2-thiol (NDPOT) (0.56 mmol) in 3.5 mL EtOH and refluxed for 12 h. Reaction mixture was solidified, and a green solid was obtained by simple filtration and used in the next step without further purification. Then, acryloyl chloride (0.28 mmol) was slowly added to a solution of obtained solid (0.2 mmol) and Et3 N (0.3 mL) in 35 mL CHCl3 at 0 °C, and the reaction mixture was stirred for 12 h at the same temperature. Then, brine solution was added and extracted using CH2Cl2 for three times (3 × 10 mL). Organics were combined and dried using anhydrous sodium sulphate and concentrated under vacuum using a rotatory evaporator. Further purification was carried out by recrystallization in EtOH. NDPOE-acrylate was obtained as a yellow solid in 71% yield, with mp 121 °C. FT-IR (KBr) ν = 3258, 2931 (C–H), 1721 (C=O), 1597 (C=C), 1527, 1334 (NO2), 1406 (N=N), 1187 (C–O) cm−1; 1H NMR (DMSO-d6) δ = 8.32–8.34 (m, 4H, CHAr), 7.71–7.79 (m, 4H, CHAr), 6.38 (dd, J = 17.2, 1.2 Hz, 1H, CHvinyl), 6.11 (dd, J = 17.2, 10.4 Hz, 1H, CHvinyl), 5.88 (dd, J = 10.4, 1.2 Hz, 1H, CHvinyl), 4.74 (t, J = 6.0 Hz, 2H, CH2–O), 4.58 (t, J = 6.0 Hz, 2H, CH–S) ppm; 13C NMR (DMSO-d6) δ = 165.9 (C=O), 153.5, 148.9, 146.9, 143.9, 132.1, 127.5, 125.4, 125.0, 122.4, 116.5, 59.9 (C–O), 44.1 (C–S) ppm; Anal. Calcd for C19H15N5O5S (425.42): C, 53.64; H, 3.55; N, 16.46. Found: C, 53.59; H, 3.54; N, 16.09.
2.3. Preparation of copolymer
N-Isopropyl acrylamide (NIPAM, 1.33 mmol), N,N′-methylenebisacrylamide (MBAM, 13.3 μmol) and N,N,N′,N′-tetramethylethylenediamine (38.67 μmol) were dissolved in a solution of SDS (12.6 mmol) in 20 mL water and stirred at 70 °C for 0.5 h under N2 atmosphere. Then, a solution of NDPOE-acrylate (185.0 μmol) in 4 mL CHCl3 was added to above solution dropwise. After evaporation of CHCl3 under reaction conditions, 0.15 g of potassium persulfate was added, and the reaction mixture was stirred at 70 °C for additional 4 h under N2 atmosphere. Then, the reaction mixture was cooled to 0–4 °C using ice bath and then filtered for removing of unreacted NDPOE-acrylate. Then, the filtrate was poured into 80 mL water, and ammonium chloride was added to make the precipitated by a salting out technique. Copolymer was obtained as orange–yellow floc-like material. FT-IR (KBr) ν = 3412 (N–H), 3096, 2968, 2922 (C–H), 1712 (C=Oacrylate), 1644 (C=Oacrylamide), 1545 (C=C and NO2), 1391 (N=N), 1335 (NO2), 1186, 1057 (C–O and C–N) cm−1; 1H NMR (DMSO-d6) (all peaks are broad.) δ = 7.26 (CHAr), 3.96, 2.16, 1.62, 1.24, 1.13 (MeNIPAM), 0.87 ppm.
3. Results and discussion
3.1. Synthesis
The pH-sensitive monomer, 2-{5-[4-((4-nitrophenyl)diazenyl)phenyl]-1,3,4-oxadiazol-2-ylthio}ethyl acrylate (NDPOE-acrylate), was prepared starting from ethyl benzoate in five steps. Benzoic acid hydrazide was prepared by heating a solution of ethyl benzoate and hydrazine hydrate in MeOH under reflux conditions for 3 h,[Citation47] which was converted to POT by refluxing with CS2 in the presence of KOH in ethanolic solution for 4 h. NDPOT was synthesized via diazo coupling reaction of POT with 4-nitrophenyl diazonium salt in water at 0–5 °C, in 48% yield, which was transformed into NDPOE-acrylate in two steps by reacting with 2-chloroethanol in ethanolic KOH solution under reflux condition for 12 h, followed by reaction with acryloyl chloride in CHCl3 in the presence of Et3 N at 0 °C, for 12 h in 71% yield (Scheme ). The overall yield of the NDPOE-acrylate monomer is 26% for last four steps.
Obtained NDPOE-acrylate was copolymerized with NIPAM by an emulsion polymerization technique using a cross-linker N,N′-methylene-bis-acrylamide (MBAM) and an initiator potassium persulfate (KPS). Due to the insolubility in water, NDPOE-acrylate was injected in the reaction medium as solution in CHCl3, in which CHCl3 was evaporated at 70 °C, leading to dispersion of NDPOE-acrylate in water in the presence of SDS. The structure of the copolymer is shown in Figure .
3.2. Characterization
3.2.1. FT-IR
In the FT-IR spectrum of the NDPOE-acrylate, a distinguished weak band corresponding to the S–H starching mode, which generally appeared at about 2401 cm−1 in the FT-IR spectra of NDPOT, had disappeared, and band corresponding to the C=O of ester moiety appeared at 1721 cm−1, demonstrating the formation of ethyl acrylate moiety. Vibrations at 1527 and 1334 cm−1 are attributed to the asymmetric and symmetric stretching modes of NO2 group, respectively. Also, the diazo stretching mode appeared at 1406 cm−1. The FT-IR spectrum of the copolymer exhibited bands at about 3100 (aromatic C–H stretching mode), 1712 (C=O of ester), 1545 and 1335 (asymmetric and symmetric modes of NO2 group), and 1391 (diazo stretching mode) cm−1, which demonstrated that the NDPOE-acrylate monomer was incorporated in the structure of the copolymer. Moreover, the N–H and C–H stretching modes of the amide moiety of NIPAM, and alkyl chains were appeared at 3412 and 2968 and 2922 cm−1, respectively. The C=O stretching of amide appeared at 1644 cm−1 (Figure ).
3.2.2. NMR spectroscopy
In the 1H NMR spectrum of NDPOE-acrylate, aromatic protons of nitro substituted phenyl ring appeared at 8.32–8.36 ppm, and the other phenyl ring protons appeared at 7.71–7.88 ppm. The three doublet of doublets (dd) at 6.38 (J = 17.2, 1.2 Hz), 6.11 (J = 17.2, 10.4 Hz) and 5.88 (J = 10.4, 1.2 Hz) ppm were assigned to the mono-substituted vinyl moiety, and two triplets at 4.74 (J = 6.0 Hz) and 4.58 (J = 6.0 Hz) ppm were attributed to the ethylene moiety of the NDPOE-acrylate. The 1H NMR spectrum of the copolymer exhibited some broad signals. The content (m/n/o) of NIPAM/ NDPOE-acrylate/MBAM units in copolymer was determined as 86.3/12.7/1% by 1H NMR analysis. As shown in 1H NMR spectra of copolymer, signal at 7.26 ppm is assigned to aromatic protons, signals at 1.13 and 1.24 ppm is assigned to the 6H of Me in i-propyl moiety of NIPAM, and signal at 3.96 ppm is assigned to CH–N of NIPAM, and CH2–O and CH2–S moieties of NDPOE-acrylate. The CH2 moiety of the MBAM appeared at 5.21 ppm as a very weak signal (Figure ).
3.2.3. Dynamic light scattering analysis studies
The size of copolymer particles dispersed in water was measured by dynamic light scattering analysis (DLS, Malvern V2.0) at different temperatures. The results are summarized in Table . The results revealed that, on increasing the temperature from 17 to 30 °C, the particle size (z average) increased from 500 to 792 nm, and then decreased to 202 nm, by further increasing the temperature until 40 °C. Particle size distribution profiles around LCST (28–34 °C) were illustrated in Figure . The higher particle size distribution, more than 1000 nm, is shown at temperatures higher than 32 °C, with low intensities.
Table 1. The size and size distribution of copolymer particles dispersed in water at different temperatures.
3.3. UV–vis spectroscopy
First, the solvatochromic properties of NDPOE-acrylate were investigated in several solvents with different polarity at room temperature. In its absorption spectra, transition bands were observed around 360, 376, 386, 388, 396 and 400 nm in CH2Cl2, CHCl3, EtOAc, dioxane and CH3CN, DMF and DMSO, respectively. As shown in Figure , polarity of solvents plays an important role in solvatochromic properties of NDPOE-acrylate that in polar solvents such as DMF and DMSO, there were significant red shifts of the absorption bands. Also, the absorbance was increased by increasing the polarity of the solvent.
Then, the pH response of NDPOE-acrylate was investigated in different pH values (pH 2–12). The absorbance of NDPOE-acrylate increased as pH values increased at λmax = 395 nm. Then, we studied the pH sensitivity of copolymer using different buffers at 20 °C. As shown in Figure , there is no considerable shift in λmax, but the intensity of the colour increased by increasing the pH, until pH 10, in that absorbance reached its maximum and kept nearly unchanged until pH 12. The absorbance of copolymer at λmax (392 nm) is plotted as function of pH values in Figure , indicating that the copolymer is sensitive to pH at pH 2–10, and at pH > 10, there is no considerable changes in the colour intensity (absorbance). Increasing the intensity can be attributed to the more hydrophobic local polarity around the dye molecules, by less hydration. Also, the more local polarity around the dye at high pHs can be deduced by a slightly red shift at λmax, from 390 nm at pH 2 to 394.5 nm at pH 12, as indicated in the case of solvatochromism of the NDPOE-acrylate monomer.
It is well known that the environmental pH may influence the polymer phase transition in the water medium and hence the LCST behaviours. The LCST of the copolymer in buffer solutions was determined by comparing their transmittances under UV–vis spectroscopy. As shown in Figure , the LCST transition curves at pH 2 and 4 are plotted at 700 nm, and the LCST of copolymer was determined by decreasing the transmittance about 50%, as 32 °C, at pH 2. In the case of pH 4, there is no considerable change in transmittance until 80 °C, but after 80 °C, the transmittance started to reduce; however, the reduced transmittance is not considerable (70% of transmittance is shown at 110 °C). In the cases of copolymer at pH ≥ 7, the LCST were not shown even above 110 °C. This can be attributed to the protonation of diazenyl moieties under acidic conditions, which underwent hydrogen bond formation with nitrogen atoms of oxadiazole heterocycle from another chain or dye molecules, providing pseudo-cross-linking in the copolymer (Figure ) and affording the globular state. But, because of the electrostatic repulsion between nitrogen atoms of oxadiazole moieties in the neutral and basic media, the changing coil to globular states did not permitted. The noteworthy proof for this statement is the low solubility of copolymer and also monomer, NDPOE-acrylate, in acidic media in comparison with neutral and basic solutions. Unlike the most reported pH and temperature responsive polymeric sensors, in which polymer phase transition (coil-to-globule) is due to the existence of thermo-responsive polymeric content only, coil-to-globule state change of this copolymer not only depend on the hydration/dehydration of PNIPAM moiety by cooling/heating, but also, pH-responsive diazenylphenyl-1,3,4-oxadiazol dye affected the polymer phase transition due to the formation of hydrogen bond between protonated diazenyl moiety and oxadiazole ring, as showed the polymer phase transition state (LCST) at pH values below 4, and LCST behaviour is not shown at pH > 4.
Figure 8. Hydrogen bond between nitrogen atoms of oxadiazole and protonated diazenyl moiety in acidic media.
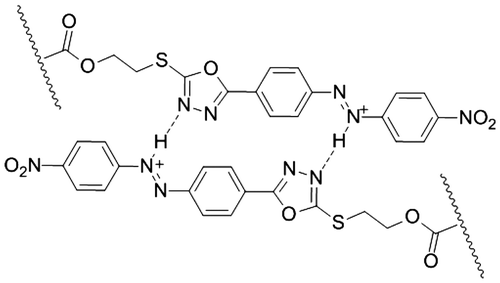
For further investigation of the temperature and pH-responsive properties of the copolymer, UV–vis absorption spectra were recorded as a function of temperature at different pH values. The absorbance of the copolymer at different pH values is plotted as function of temperature in λmax in a radar-type plot (Figure ). As shown in Figure , there is no considerable change in absorbance at the pH ≥ 7 at different temperatures. But, in the case of pH 4, a minor change was shown, and the maximum absorbance appeared at 60 °C. In the case of pH 2, considerable changes is shown in absorbance by changing the temperature, and the maximum absorbance appeared at 45 °C. These results revealed that the UV–vis properties of copolymer are independent to temperature at pH ≥ 7 and are slightly dependent to temperature at pH 4. In the case of pH 2, the UV–vis of copolymer indicated the high dependency to the temperature.
3.4. Fluorescent spectroscopy
Figure shows the fluorescence spectra of NDPOE-acrylate in solvents with different polarity such as CHCl3, THF, DMF and DMSO. The emission peaks of NDPOE-acrylate are shifted from 470 nm in CHCl3 and 484 nm in THF and DMF to 490 nm in DMSO, due to the significant interaction between the ICT excited state and the solvent molecules. Also, we investigated the fluorescence properties of NDPOE-acrylate at different pH values at 25 °C. NDPOE-acrylate exhibited two weak emissions at 471 and 683 nm; in both, there is no considerable change in the fluorescence intensity as function of pH values.
Then, the fluorescence response of copolymer was studied at different pH values and temperatures. The copolymer exhibited sensitive fluorescence change towards pH between 2 and 10 at different temperatures. Above 32 °C, the fluorescence intensity of copolymer increased as the pH values increased until pH 7, in which the fluorescence intensity attained its maximum; then, the fluorescence intensity reached its minimum at pH 10 and kept nearly unchanged until pH 12. The fluorescence intensity of copolymer at different pH values is plotted as function of temperature (Figure ). Below LCST (32 °C), the copolymer exhibited the maximum fluorescence intensity at pH < 4. The fluorescence intensity of copolymer is nearly unchanged until 38–40 °C and then decreased as the temperature increased at pH ≥ 4. The fluorescence intensity of copolymer in the acidic medium (pH 2) was stronger than that in weekly acidic, neutral and basic media (pH ≥ 4) below 32 °C, the LCST of copolymer at pH 2. The sudden decrease in the fluorescence intensity of copolymer at pH 2 between 30 and 32 °C is attributed to the polymer phase transition (coil-to-globule), in which the rigid (globule) shape of copolymer exhibited lower fluorescence intensity above 32 °C rather than coil shape below 32 °C.
4. Conclusion
In summary, we have synthesized a novel heterocyclic-based acrylate monomer and its water-soluble polymeric sensor which responds to both pH and temperature in aqueous solution. The copolymer exhibited change of colour (absorbance) towards pH between 2 and 10 at 25 °C. Also, the copolymer exhibited a sensitive fluorescent change towards pH between 2 and 10 at different temperature and showed strong fluorescence at pH 7. Meanwhile, the fluorescence intensity of copolymer decreased as the temperature increased; this copolymer could be used as a thermometer to detect the temperature range from 40 to 75 °C, and 30 to 65 °C in aqueous solutions at pH between 4 and 12, and 2, respectively.
Acknowledgements
The work was supported by research council of the University of Maragheh. The corresponding author thanks Prof A. Jouyban (Drug Applied Research Center, Tabriz University of Medical Sciences) for fluorescent spectra, Dr A. Ziyaei Halimehjani (Kharazmi University) for NMR spectra of copolymer, and Prof G.R. Mahdavinia for his kind advisement during the work.
Disclosure statement
No potential conflict of interest was reported by the authors.
References
- Xiao Y, Pavlov V, Niazov T, Dishon A, Kotler M, Willner I. Catalytic beacons for the detection of DNA and telomerase activity. J. Am. Chem. Soc. 2004;126:7430–7431.10.1021/ja031875r
- Xu W, Xue XJ, Li TH, Zeng HQ, Liu XG. Ultrasensitive and selective colorimetric DNA detection by nicking endonuclease assisted nanoparticle amplification. Angew. Chem. Int. Ed. 2009;48:6849–6852.10.1002/anie.v48:37
- Aoshima S, Kanaoka S. Synthesis of stimuli-responsive polymers by living polymerization: poly(N-isopropylacrylamide) and poly(vinyl ether)s. Adv. Polym. Sci. 2008;210:169–208.
- Gil ES, Hudson SM. Stimuli-reponsive polymers and their bioconjugates. Prog. Polym. Sci. 2004;29:1173–1222.10.1016/j.progpolymsci.2004.08.003
- Zhang L, Guo R, Yang M, Jiang X., Liu B. Thermo and pH dual-responsive nanoparticles for anti-cancer drug delivery. Adv. Mater. 2007;19:2988–2992.10.1002/(ISSN)1521-4095
- Schmaljohann D. Thermo- and pH-responsive polymers in drug delivery. Adv. Drug Delivery Rev. 2006;58:1655–1670.10.1016/j.addr.2006.09.020
- Heffernan MJ, Murthy N. Polyketal nanoparticles: a new pH-sensitive biodegradable drug delivery vehicle. Bioconjugate Chem. 2005;16:1340–1342.10.1021/bc050176w
- Zhang C, Suslick KS. A colorimetric sensor array for organics in water. J. Am. Chem. Soc. 2005;127:11548–11549.10.1021/ja052606z
- Edwards EW, Chanana M, Wang D, Möhwald H. Stimuli-responsive reversible transport of nanoparticles across water/oil interfaces. Angew. Chem. Int. Ed. 2008;47:320–323.10.1002/(ISSN)1521-3773
- Shiraishi Y, Miyamoto R, Hirai T. A hemicyanine-conjugated copolymer as a highly sensitive fluorescent thermometer. Langmuir. 2008;24:4273–4279.10.1021/la703890n
- Guo ZQ, Zhu WH, Xiong YY, Tian H. Multiple logic fluorescent thermometer system based on N-isopropylmethacrylamide copolymer bearing dicyanomethylene-4H-pyran moiety. Macromolecules. 2009;42:1448–1453.10.1021/ma802660e
- Gota C, Okabe K, Funatsu T, Harada Y, Uchiyama S. Hydrophilic fluorescent nanogel thermometer for intracellular thermometry. J. Am. Chem. Soc. 2009;131:2766–2767.10.1021/ja807714j
- Gao XH, Cui YY, Levenson RM, Chung LWK, Nie SM. In vivo cancer targeting and imaging with semiconductor quantum dots. Nat. Biotechnol. 2004;22:969–976.10.1038/nbt994
- Koopmans C, Ritter H. Color change of N-isopropylacrylamide copolymer bearing Reichardts dye as optical sensor for lower critical solution temperature and for host–guest interaction with β-cyclodextrin. J. Am. Chem. Soc. 2007;129:3502–3503.10.1021/ja068959v
- Roth I, Jbarah AA, Holze R, Friedrich M, Spange S. 2-nitro-1,4-diaminobenzene-functionalized poly(vinyl amine)s as water-soluble UV–vis-sensitive pH sensors. Macromol. Rapid Commun. 2006;27:193–199.10.1002/(ISSN)1521-3927
- Hasegawa T, Kondo Y, Koizumi Y, Sugiyama T, Takeda A, Ito S, Hamada F. A highly sensitive probe detecting low pH area of HeLa cells based on rhodamine B modified β-cyclodextrins. Bioorg. Med. Chem. 2009;17:6015–6019.10.1016/j.bmc.2009.06.046
- Borisov SM, Wolfbeis OS. Temperature-sensitive europium(III) probes and their use for simultaneous luminescent sensing of temperature and oxygen. Anal. Chem. 2006;78:5094–5101.10.1021/ac060311d
- Borisov SM, Vasylevska AS, Krause C, Wolfbeis OS. Composite luminescent material for dual sensing of oxygen and temperature. Adv. Funct. Mater. 2006;16:1536–1542.10.1002/(ISSN)1616-3028
- Jorge PAS, Maule C, Silva AJ, Benrashid R, Santos JL, Farahi F. Dual sensing of oxygen and temperature using quantum dots and a ruthenium complex. Anal. Chim. Acta. 2008;606:223–229.10.1016/j.aca.2007.11.008
- Borisov SM, Seifner R, Klimant I. A novel planar optical sensor for simultaneous monitoring of oxygen, carbon dioxide, pH and temperature. Anal. Bioanal. Chem. 2011;400:2463–2474.10.1007/s00216-010-4617-4
- Schroeder CR, Neurauter G, Klimant I. Luminescent dual sensor for time-resolved imaging of pCO2 and pO2 in aquatic systems. Microchim. Acta. 2007;158:205–218.10.1007/s00604-006-0696-5
- Borchert NB, Ponomarev GV, Kerry JP, Papkovsky DB. O2/pH multisensor based on one phosphorescent dye. Anal. Chem. 2011;83:18–22.10.1021/ac1025754
- Schneider K, Schütz V, John GT, Heinzle E. Optical device for parallel online measurement of dissolved oxygen and pH in shake flask cultures. Bioprocess Biosyst. Eng. 2010;33:541–547.10.1007/s00449-009-0367-0
- Mistlberger G, Koren K, Borisov SM, Klimant I. Magnetically remote-controlled optical sensor spheres for monitoring oxygen or pH. Anal. Chem. 2010;82:2124–2128.10.1021/ac902393u
- Kocincová AS, Nagl S, Arain S, Krause C, Borisov SM, Arnold M, Wolfbeis OS. Multiplex bacterial growth monitoring in 24-well microplates using a dual optical sensor for dissolved oxygen and pH. Biotechnol. Bioeng. 2008;100:430–438.10.1002/(ISSN)1097-0290
- Stich MIJ, Nagl S, Wolfbeis OS, Henne U, Schaeferling M. A dual luminescent sensor material for simultaneous imaging of pressure and temperature on surfaces. Adv. Funct. Mater. 2008;18:1399–1406.10.1002/adfm.v18:9
- Stich MIJ, Schaeferling M, Wolfbeis OS. Multicolor fluorescent and permeation-selective microbeads enable simultaneous sensing of pH, oxygen, and temperature. Adv. Mater. 2009;21:2216–2220.10.1002/adma.v21:21
- Wang D, Liu T, Yin J, Liu S. Stimuli-responsive fluorescent poly(N-isopropylacrylamide) microgels labeled with phenylboronic acid moieties as multifunctional ratiometric probes for glucose and temperatures. Macromolecules. 2011;44:2282–2290.10.1021/ma200053a
- Pietsch C, Hoogenboom R, Schubert US. Soluble polymeric dual sensor for temperature and pH value. Angew. Chem. Int. Ed. 2009;48:5653–5656.10.1002/anie.v48:31
- Li YY, Cheng H, Zhu JL, Yuan L, Dai Y, Cheng SX, Zhang XZ, Zhuo RX. Temperature- and pH-sensitive multicolored micellar complexes. Adv. Mater. 2009;21:2402–2406.10.1002/adma.v21:23
- Uchiyama S, Kawai N, de Silva AP, Iwai K. Fluorescent polymeric and logic gate with temperature and pH as inputs. J. Am. Chem. Soc. 2004;126:3032–3033.10.1021/ja039697p
- Cui K, Zhu D, Cui W, Lu X, Lu Q. Dual-responsive ionically assembled fluorescent nanoparticles from copoly(ionic liquid) for temperature sensor. J. Phys. Chem. C. 2012;116:6077–6082.10.1021/jp211847b
- Tang B, Yu F, Li P, Tong LL, Duan X, Xie T, Wang X. A near-infrared neutral pH fluorescent probe for monitoring minor pH changes: imaging in living HepG2 and HL-7702 cells. J. Am. Chem. Soc. 2009;131:3016–3023.10.1021/ja809149g
- Wan XJ, Wang D, Liu SY. Fluorescent pH-sensing organic/inorganic hybrid mesoporous silica nanoparticles with tunable redox-responsive release capability. Langmuir. 2010;26:15574–15579.10.1021/la102148x
- Galindo F, Burguete MI, Vigara L, Luis SV, Kabir N, Gavrilovic J, Russell DA. Synthetic macrocyclic peptidomimetics as tunable pH probes for the fluorescence imaging of acidic organelles in live cells. Angew. Chem. Int. Ed. 2005;44:6504–6508.10.1002/(ISSN)1521-3773
- Pringsheim E, Zimin D, Wolfbeis OS. Fluorescent beads coated with polyaniline: A novel nanomaterial for optical sensing of pH. Adv. Mater. 2001;13:819–822.10.1002/1521-4095(200106)13:11<819::AID-ADMA819>3.0.CO;2-D
- Yan Q, Yuan J, Kang Y, Cai Z, Zhou L, Yin Y. Dual-sensing porphyrin-containing copolymer nanosensor as full-spectrum colorimeter and ultra-sensitive thermometer. Chem. Commun. 2010;46:2781–2783.10.1039/b926882k
- Pietsch C, Schubert US, Hoogenboom R. Aqueous polymeric sensors based on temperature-induced polymer phase transitions and solvatochromic dyes. Chem. Commun. 2011;47:8750–8765; and references cited therein.10.1039/c1cc11940k
- Katsumoto Y, Tanaka T, Sato H, Ozaki Y. Conformational change of poly(N-isopropylacrylamide) during the coil–globule transition investigated by attenuated total reflection/infrared spectroscopy and density functional theory calculation. J. Phys. Chem. A. 2001;106:3429–3435.
- Schild HG. Poly(N-isopropylacrylamide): experiment, theory and application. Prog. Polym. Sci. 1992;17:163–249.10.1016/0079-6700(92)90023-R
- Heskins M, Guillet JE, James E. Solution properties of poly(N-isopropylacrylamide). J. Macromol. Sci. Chem. 1968;2:1441–1455.10.1080/10601326808051910
- Eftekhari-Sis B, Zirak M. Chemistry of α-oxoesters: A powerful tool for the synthesis of heterocycles. Chem. Rev. 2015;115:151–264.10.1021/cr5004216
- Eftekhari-Sis B, Zirak M, Akbari A. Arylglyoxals in synthesis of heterocyclic compounds. Chem. Rev. 2013;113:2958–3043.10.1021/cr300176g
- Eftekhari-Sis B, Akbari A, Amirabedi M. Synthesis of new N-alkyl(aryl)-2,4-diaryl-1H-pyrrol-3-ols via aldol Paal–Knorr reactions. Chem. Heterocycl. Comp. 2011;46:1330–1334.10.1007/s10593-011-0669-4
- Khalili B, Jajarmi P, Eftekhari-Sis B, Hashemi MM. Novel one-pot, three-component synthesis of new 2-alkyl-5-aryl-(1H)-pyrrole-4-ol in water. J. Org. Chem. 2008;73:2090–2095.10.1021/jo702385n
- Young RW, Wood KH. The cyclization of 3-acyldithiocarbazate esters. J. Am. Chem. Soc. 1955;77:400–403.10.1021/ja01607a051
- Furniss BS, Hannaford AJ, Smith PWG, Tatchell AR. Vogel’s textbook of practical organic chemistry. 5th ed. Harlow: Longman; 1989. p. 1269.