Abstract
We synthesized new bi-sensitive statistical copolymers and A-B-A triblock copolymers from 2-cyclopropyl-2-oxazoline (CPOxa) and 2-methoxycarbonylethyl-2-oxazoline (MEtOxa) using 1,4-dibromobutene as a bi-functional initiating moiety. These new bi-sensitive polymers were obtained via quasi-living polymerization of the oxazoline monomers and subsequent hydrolysis of the methyl ester of MEtOxa. Their thermal conformational transitions in aqueous solution were between 30 and 60 °C and these values were dependent of the copolymer composition, polymer structure (statistical or block copolymer), and of the pH of the solution, where the polymers were dissolved. The achieved polymers could find application in biomaterial science, in microsystems and sensors, and as smart nanocarriers.
1. Introduction
Currently, the synthesis and properties of macromolecules which display sensitivity to environmental changes such as temperature, pH, light, and magnetic and electric fields are widely investigated. These macromolecules have high potential for applications as biomaterials and membranes and in microsystems, sensors, and catalysis.[Citation1–3]
One way to create polymers with thermal response is using homopolymers and copolymers of N-isopropylacrylamide, N-vinylcaprolactame, and vinylmethylether. In recent years, some polymers of 2-oxazolines were widely investigated for their applications as polymers with thermal sensitivity such as poly(isopropyl-2-oxazoline), poly(n-propyl-2-oxazoline), poly(ethyl-2-oxazoline), and recently poly(cyclopropyl-2-oxazoline).[Citation4–12] This class of polymers presents, in aqueous solution, well-defined conformational transition where the physical properties change drastically in a narrow temperature range.[Citation3,5–17] This temperature is called lower critical solution temperature (LCST).[Citation3]
Furthermore, there are some polymers with pH sensitivity, for example, polybases as poly(vinylpyridine) or polyacids as poly(acrylic acid). Polybases are protonated at low pH values and this improves their water solubility. In contrast, polyacids are more soluble in water at high pH values, where strongly polar carboxylic groups are present.[Citation17]
Polymerization of 2-oxazolines has been investigated intensively.[Citation18–30] Poly(2-oxazolines) can be obtained by living cationic ring-opening polymerization (CROP). Initiators suitable for this polymerization are Brönsted or Lewis acids, tosylate, methyl triflate, and alkyl halide (e.g., benzyl chloride and 1,4-dibromobutene). Living polymerization of 2-oxazolines takes place under inert reaction conditions (monomers and solvents with high-purity removal of nucleophiles such as amines, alcohols, and moisture traces); this means that it occurs without termination reactions or chain transfer giving control over molecular weight, distribution, and functionality of the polymers from the beginning of reaction, and thus enabling the synthesis of a wide range of well-defined (co)polymers and polymer architectures.
Recently, poly(2-oxazolines) such as poly(cyclopropyl-2-oxazoline), poly(isopropyl-2-oxazoline), and poly(n-propyl-2-oxazoline) and their copolymers have attracted much scientific interest due to their temperature sensitivity. It was demonstrated that LCST of these polymers may vary through copolymerization of their monomers with hydrophilic and hydrophobic monomers or by the addition of surfactants and salts, similar to what happens with poly(N-isopropylacrylamide).[Citation5–11]
However, the phase transitions observed in polyoxazolines have advantages over poly(N-isopropylacrylamide) (PNiPAAm). Thus, they do not have the undesired effect of hysteresis that appears with PNiPAAm.[Citation5–7] Also, according to recent investigations, the PNiPAAm contain monomer residues that are known to have neurotoxic effects, whereas certain polyoxazolines obtained FDA approval already. Schubert et al. demonstrated that the polymerization of cyclopropyl-2-oxazoline is faster than that of other oxazolines [Citation11] due to the withdrawing effect of the cyclopropyl group. In addition, poly(cyclopropyl-2-oxazoline) has a LCST of 30 °C which is close to body temperature.
Two recent articles deal with kinetic studies on the homo and copolymerizations of 2-methoxycarbonylethyl-2-oxazoline with 2-alkyl-2-oxazolines.[Citation31,32] In this study, we present the synthesis and characterization of statistical and block copolymers of 2-cyclopropyl-2-oxazoline (CPOxa) and 2-methoxycarbonylethyl-2-oxazoline (MEtOxa) with thermal and pH sensitivity after hydrolysis of the methyl ester.
2. Experimental
2.1. Materials
Benzonitrile was purchased from Aldrich and distilled twice from CaH2 and stored under a dry nitrogen atmosphere. MEtOxa was synthesized from 2-chloroethylamine hydrochloride and methylsuccinate chloride as reported by Zarka et al. [Citation33]. CPOxa was synthesized from cyclopropylnitrile and ethanolamine as described in the literature.[Citation11,34] Structure and purity of the monomers were confirmed by NMR spectroscopy. The others substances were purchased from Aldrich and used as received.
2.2. Analytical measurements
The 1H and 13C NMR spectra were recorded on a Bruker Avance III 500 spectrometer operating at 500.13 MHz for 1H and at 125.75 MHz for 13C. Solutions in CDCl3 were referenced on the solvent peak (δ (1H) = 7.26 ppm, δ (13C) = 77.0 ppm). Spectra recorded from D2O solutions were referenced on internal sodium 3-(trimethylsilyl)-3,3,2,2-tetradeuteropropionate (δ (1H) = 0 ppm, δ (13C) = 0 ppm). Size exclusion chromatography (SEC) was performed on modular system built from a HPLC pump, RI detector (1100 Series, Agilent Technologies, USA) and two Zorbax PSM Trimodal-S columns (Agilent Technologies, USA). DMAc containing 3 g/L LiCl and 2 vol.% water was used as an eluent at a flow rate of 0.5 mL/min. The molecular weights were determined based on the calibration with poly(4-vinylpyridine) standards.
2.3. Synthesis of poly(2-cyclopropyl-2-oxazoline) (PCPOxa, HP)
A mixture of 0.05 g (0.234 mmol) of 1,4-dibromobutene and 4.5 mL of benzonitrile was placed in a 50 mL round-bottom flask with nitrogen inlet. Then, CPOxa (1.57 g, 14.18 mmol) was added under dry nitrogen flow. The flask was closed and heated to 100 °C. After the desire reaction time (1, 2, 3, and 4 h, respectively), the mixture was cooled down to room temperature and a solution of KOH in methanol was added to terminate the reaction. Finally, the polymer was isolated by precipitation in chloroform/heptane and dried at 40 °C until constant weight. Yield: 85% (HP-1 h), 99% (HP-2 h, -3 h, -4 h).
1H NMR (CDCl3): δ 0.7–1.0 (CH2), 1.5–2.0 (CH), 3.3–3.9 (NCH2CH2), 4.0–4.2 (=CHCH2–), 5.5–5.7 ppm (=CH–).
13C NMR (CDCl3): δ 8.4 (CH2), 10.0–11.0 (CH), 44–49 (NCH2CH2), 50.5 (=CHCH2–), 127.5 (=CH–), 173–175 ppm (NC(O)).
2.4. Synthesis of statistical (st CP) and block copolymers (bl CP) from CPOxa and MEtOxa monomers
The procedure was similar to the synthesis of poly(2-cyclopropyl-2-oxazoline) homopolymer, but in case of statistical copolymers (st CP), a mixture of 2-cyclopropyl-2-oxazoline and 2-methoxycarbonylethyl-2-oxazoline (cf. Table ) was added to 1,4-dibromobutene and the reaction was carried out for 5 h at 100 °C. The block copolymers (bl CP) were synthesized by adding CPOxa first. After 3 h reaction at 100 °C, the desired amount of MEtOxa monomer (cf. Table ) was added and the reaction was continued for 5 h at 100 °C.
Table 1. Composition and characteristics of statistical copolymers from 2-cyclopropyl-2-oxazoline and 2-methoxycarbonylethyl-2-oxazoline.
Table 2. Composition and characteristics of A-B-A block copolymers from 2-cyclopropyl-2-oxazoline (block B) and 2-methoxycarbonylethyl-2-oxazoline (block A).
All polymerizations were terminated with a solution of KOH in methanol. The polymers were isolated by precipitation in chloroform/heptane and dried.
Yield: 96% (st CP1), 99% (st CP2), 99% (st CP3), 86% (bl CP1), 90% (bl CP2), 98% (bl CP3).
1H NMR (CDCl3): δ 0.7–1.0 (CH2 of CPOxa), 1.5–2.0 (CH of CPOxa), 2.55–2.8 (C(O)CH2CH2C(O) of MEtOxa), 3.3–3.9 (NCH2CH2), 3.65 (OCH3 of MEtOxa), 4.0–4.2 (=CHCH2–), 5.5–5.7 ppm (=CH–).
13C NMR (CDCl3): δ 8.3 (CH2 of CPOxa), 10.0–11.5 (CH of CPOxa), 27.5 (NC(O)CH2 of MEtOxa), 28.8 (CH2C(O)O of MEtOxa), 43–49 (NCH2CH2), 50.5 (=CHCH2–), 51.6 (OCH3 of MEtOxa), 127.5 (=CH–), 172.0 (NC(O) of MEtOxa), 173–175 (NC(O) of CPOxa), 173.5 ppm (C(O)OCH3).
2.5. Hydrolysis of statistical and block copolymers to their acid forms st CP-H and bl CP-H
To hydrolyze the methyl ester groups, 1.0 g of the copolymer was dissolved in a mixture of 20 mL of 0.1 N NaOH and 8 mL methanol. The mixture was heated to 55 °C for 7 h under constant stirring. After the reaction time, the mixture was acidified at room temperature with 0.1 N HCl until pH 5.6. The methanol was removed and the polymer was purified by dialysis (membrane with MWCO 1000). Finally, the solution was freeze-dried, resulting in a soft white polymer.
1H NMR (D2O): δ 0.7–1.0 (CH2 of CPOxa), 1.8–2.1 (CH of CPOxa), 2.48 (CH2COOH of HEtOxa), 2.55–2.75 (NC(O)CH2 of HEtOxa), 3.4–4.0 (NCH2CH2), 4.15–4.3 (=CHCH2–), 5.5–5.7 ppm (=CH–).
13C NMR (D2O): δ 10–12 (CH2 of CPOxa), 13.5 (CH of CPOxa), 31.5 (NC(O)CH2 of HEtOxa), 34.7 (CH2COOH of HEtOxa), 45.5–50 (NCH2CH2), 52–54 (=CHCH2–), 130.0 (=CH–), 178.1 (NC(O) of HEtOxa), 179.3 and 179.6 (NC(O) of CPOxa), 183.0 ppm (COOH of HEtOxa).
2.6. Determination of phase transition temperature (Ttr) of the statistical and block copolymers
UV/Vis spectral turbidity measurements were carried out on a Helios Gamma spectrophotometer (Thermo Electron Corporation) equipped with a flexible thermocouple allowing direct and continuous monitoring of the sample temperature. A 1 wt.% polymer solution was prepared by dissolving 0.05 g of polymer in 5 mL of distillated water. Polymer solutions with pH values lower or higher than the pH value of the native solution were prepared by dropwise addition of HCl and NaOH solutions, respectively. The solution was filtered and transferred in a UV/Vis cuvette. The cuvette temperature was varied with a heating jacket and a thermostat bath. Optical transmittance of the polymer solutions was measured at 550 nm at different temperatures without stirring. The phase transition temperature Ttr of the polymer was determined as the inflection point of the transmittance versus temperature curve.
3. Results and discussions
3.1. Synthesis of statistical copolymers from 2-cyclopropyl-2-oxazoline (CPOxa) and 2-methoxycarbonylethyl-2-oxazoline (MEtOxa)
Statistical copolymers of CPOxa and MEtOxa were synthesized through cationic ring-opening polymerization using a bi-functional initiator and different initial monomer molar ratios (Scheme ).
The copolymerization was carried out at 100 °C for 5 h using 1,4-dibromobutene (DBB) as a bi-functional initiator and benzonitrile as the solvent. Yields obtained were between 96 and 99%. Table shows the detailed experimental data and results of the polymerization. The experimental molar ratio of MEtOxa and CPOxa and the degree of polymerization were calculated from appropriated 1H NMR signal integrals and were in good agreement with the theoretical values. The degrees of polymerization determined by the end group method (here: using the initiating butene group) are less accurate because the low intensity of the signals of the initiating butene unit results in a larger error for these integrals. Statistical copolymers containing units of CPOxa and MEtOxa were aimed for; however, due to the difference in reactivity of the two monomers (CPOxa is more reactive than MEtOxa), possibly gradient polymers instead of statistical copolymers were formed. Glassner et al. [Citation12] tuned the LCST of poly(2-cyclopropyl-2-oxazoline) via gradient copolymerization with 2-ethyl-2-oxazoline. Bouten et al. [Citation31,32] used their kinetic studies on the copolymerization of 2-methoxycarbonylethyl-2-oxazoline with 2-alkyl-2-oxazolines to estimate the monomer distribution in the resulting gradient polymers. Our gradient polymers could have a structure where at first CPOxa polymerized predominantly, forming a kind of middle block due to the bi-functional initiator, followed by a transition period, where both monomers polymerize almost randomly, and finally the remaining MEtOxa monomers polymerize. Still, the structure formed will depend, in kinetic terms, on the initial monomer molar ratio.
The reaction mechanism proposed for these polymerizations is a partial ionic mechanism. The nitrogen atom with basic character of the CPOxa reacts with the carbon of the bromomethylene group of the initiator DBB producing both an oxazolinic cation and bromide counterion. Then, the propagation occurs through the successive attack of monomer molecules to the oxazolinium cation. The proposed mechanism is based also on a previous study,[Citation35] where the polymerization of 2-methyl-2-oxazoline (MeOxa) with initiator DBB at 70 °C, acetonitrile as the solvent, and reaction time of 8 h produced polymers (bismacromonomers) with a polymerization degree of 10–54 and dispersities from 1.10 to 1.20. It was deduced that the bromide counterion was less nucleophilic than MeOxa, and therefore the polymerization proceeds in a living fashion through an ionic mechanism. Similarly, it is assumed in our study that the bromide counterion might be less nucleophilic than CPOxa and MEtOxa, and thus may also promote an ionic mechanism.[Citation11,18,35]
The copolymers were characterized via 1H NMR and 13C NMR spectroscopy. Figure (a) and (b) depicts the 1H and 13C NMR spectra of st CP2. There are typical signal regions due to cyclopropyl (signals a–c) and methoxycarbonylethyl side groups (signals e–i). Also, signals of the initiating butene unit can be identified (k, l) and allow an estimation of the degree of polymerization, comparing its integral intensity with signal intensities of the reacted monomer units. The molar ratio of both monomers was calculated from the 1H NMR signal integrals of proton c (CPOxa) and protons f and g (MEtOxa).
Figure 1. 1H (a,c) and 13C NMR spectra (b,d) of a statistical copolymer of CPOxa and MEtOxa (st CP2; a,b; solvent: CDCl3) and the corresponding copolymer after hydrolysis (st CP2-H; c,d; solvent: D2O).
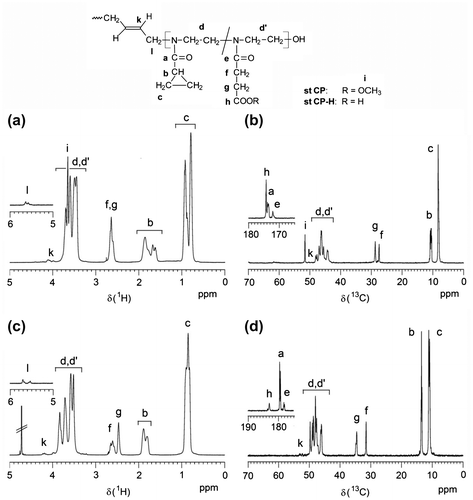
Results of SEC, displayed in Table , show degrees of polymerization and molecular weights close to those calculated theoretically for the polymers, but with high dispersity. The results of NMR and SEC state that the oxazoline polymerization was less ‘living’ as expected and the broad distribution in molecular weight suggests slow initiation or propagation. One can assume that not all initiating bromide functions start a polymer chain at the same time since the ionic pathway is favored but not exclusively taking place, as in the case of iodide initiating functions, and the slower covalent propagation mechanism may still compete to some extent.
The copolymers hydrolyze at mild conditions, as 55 °C, 7 h, and low sodium hydroxide concentration (0.1 N) allows selective hydrolysis of ester groups avoiding amide hydrolysis. Complete hydrolysis of MEtOxa to HEtOxa units was confirmed by the disappearance of the methyl ester group signals in the 1H NMR (~3.65 ppm, Figure (c)) and in the 13C NMR spectrum (~51.6 ppm, Figure (d)) of the hydrolyzed polymer.
3.2. Synthesis of A–B–A block copolymers from CPOxa and MEtOxa
Prior to the synthesis of triblock polymers, it was important to know reaction times for the monomer CPOxa to achieve almost 100% conversion. Therefore, the synthesis of the homopolymer of CPOxa was carried out using the same initiator and reaction conditions of the statistical and triblock copolymers. We determined that the reaction time for almost 100% monomer conversion is approximately 2 h, resulting in a polyCPOxa with DP = 60, which corroborates Schubert’s findings about the high polymerization rate of the cyclopropyloxazoline compared to other oxazolines,[Citation11] although Schubert used another polymerization system (initiator was methyl tosylate, temperature at 140 °C, solvent was acetonitrile, and microwave heating).
Likewise, it was determined that MEtOxa, under the same reaction conditions, polymerized in about 5 h with 100% conversion.
Subsequently, by the method of sequential addition of monomers, block copolymers were synthesized from the mentioned monomers. This is possible because of the ‘living’ nature of the polymerization of 2-oxazolines. So, after consuming the first monomer (CPOxa), the polymerization of the second monomer (MEtOxa) can be initiated from the ‘living’ chain end of first segment.
ABA-type block copolymers were obtained in our case because the initiator (DBB) is bi-functional. The internal segment (block B) was polyCPOxa and the external segment (block A) was polyMEtOxa. The yield of the copolymerization was relative high (>86%) and the presence of both monomers in the polymer was confirmed by NMR spectroscopy. Detailed information and experimental results are shown in Table . Despite some small differences in the signal pattern of statistical and block copolymers, there are no obvious features in both the 1H and 13C NMR spectra which allow a certain distinction between both copolymer structures. Nevertheless, the comonomer contents and the number average length of both blocks can be calculated from 1H NMR signal integrals as described for the statistical copolymers. Thus, about 49–56 monomer units were calculated for the PCPOxa block (block B) centered by the butene initiator unit. The average number of MEtOxa units added in the second reaction step on each side of this central block B is increasing from 6 to 21 (block A). Thus, the comonomer CPOxa to MEtOxa ratio is changing (cf. Table ) from about 49/12 (bl CP1) to 49/42 (bl CP-3).
Mn values of all polymers determined by SEC were smaller as calculated from feed ratio or NMR signals, as also observed for the statistical copolymers (Table ). Possibly, this is due to different interactions with the SEC solvent of the amphiphilic block copolymers compared to the standard (polyvinylpyridine) used in the SEC calibration. The molecular weight also exhibited a relatively broad dispersity, indicating a slow initiation and propagation, similar as in the case of the statistical copolymers.
Also, the block copolymers were hydrolyzed in aqueous NaOH solution resulting in 100% saponification as proved by NMR spectroscopy. The hydrolyzed forms of the polymers were obtained by adjusting the pH value to 5.6 before polymer isolation.
3.3. Determination of phase transition temperature (Ttr) by turbidimetry
The Ttr of homopolymer HP-1 h with a polymerization degree of approximately 50 was ~36 °C (cf. Table ). For HP-4 h with a polymerization degree of 60, the Ttr is 31.3 °C. This result is consistent with other temperature-sensitive polymers as, for example, polyNiPAAm, where LCST value increases with decrease in molecular weight.
Table 3. Phase transitions temperatures of CPOxa polymers and CPOxa/MEtOxa copolymers measured by turbidimetry.
In Figure , it is shown that Ttr of copolymers increases in the series st CP1, st CP2, and st CP3 and Ttr is about 31, 34, and 37 °C, respectively (cf. Table ). This is corresponding to the fact that the percentage of hydrophilic comonomer MEtOxa increases from st CP1 to st CP3.
Figure 2. Temperature-dependent turbidity measurements of statistical copolymers from CPOxa and MEtOxa.
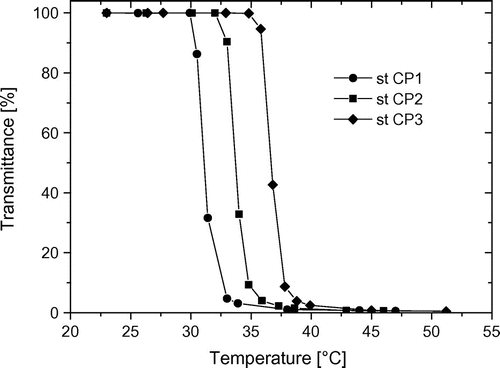
When the polymers are hydrolyzed, the hydrophilic character increases due to the appearance of strongly polar carboxylic acid group, which, with the variation of the pH, changes from acid form to carboxylate and vice versa. Recent studies showed that at pH 5.6, a 1:1 equilibrium exists between both carboxylic acid and carboxylate groups.[Citation26]
Figure (cf. Table ) presents the turbidity results of the hydrolyzed statistical copolymers, which have higher Ttr values than the non-hydrolyzed polymers, which is also consistent with the theory. In addition, for st CP2-H (hydrolyzed polymer), the hydrophilic content is so high that no macroscopic aggregates are formed in the inspected temperature range. At this point, one cannot distinguish if this is due to the full loss of LCST behavior or due to the presumably blocky structure and potential certain amphiphilic nature, if micelles or nanometer-sized molecular aggregates have formed that do only lead to a slight transmittance decrease. The same is observed for st CP3-H (not shown).
Figure 3. Temperature-dependent turbidity measurements of statistical copolymers from CPOxa and MEtOxa (st CP1 and st CP2) in ester form (filled symbols) and in acid form at pH 5.6 (open symbols).
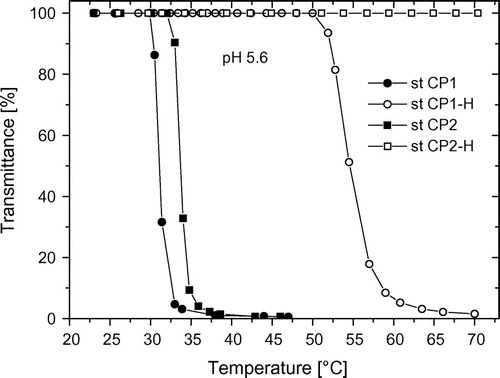
Table 4. Phase transitions temperatures of hydrolyzed CPOxa/CEtOxa statistic and block copolymers measured by turbidimetry at varied pH values.
Measurements on the hydrolyzed copolymer at different pH values were also performed. In Figure , for example, the results of st CP1-H are shown. At high pH values (pH 12), the carboxylic acid groups exist as carboxylates and that gives a high polarity to the copolymer, and thus the Ttr value significantly increases but the transmittance decreases only to 45%. Conversely, at pH 1, only carboxylic acid groups are present and those can allow for hydrogen bonding interactions between amide groups of the cyclopropyl-2-oxazoline and carboxylic acid groups inducing the appearance of the LCST phenomenon at lower temperatures.[Citation16,36] Otherwise, it is also possible that the HEtOxa unit in the acid form has a hydrophobic character and causes a decrease of Ttr (cf. Table ). At pH 5.6, the situation is intermediate because carboxylates and acid groups coexist.
Figure 4. Temperature-dependent turbidity measurements of hydrolyzed statistical copolymer st CP1-H at different pH values.
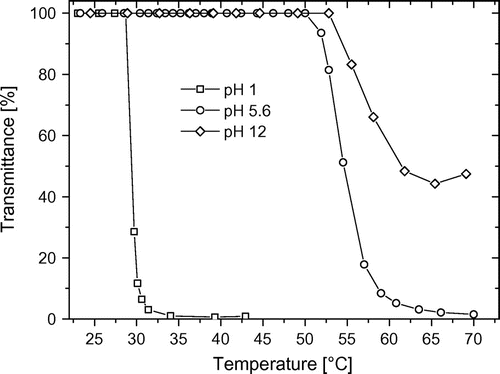
The Ttr of the block copolymers (cf. Table ) do not present significant differences between bl CP1, bl CP2, and bl CP3, even though within this series there are variations in the hydrophilic MEtOxa comonomer content (cf. Table ). The Ttr value is about 33 °C for all polymers. This could be explained by the fact that in contrast to statistical polymers, in block polymers, the MEtOxa units are not mixed with CPOxa units, but are isolated as a segment at end of the polymer chain. In other words, the polyCPOxa segment in the middle of the polymeric induced the chain collapse and this is only slightly influenced by polyMEtOxa segments.
However, there is a great difference when one compares the ester form and the hydrolyzed form of the block copolymers. At pH 1, HEtOxa units are completely non-dissociated and the Ttr distinctly decreases with increase in length of the more hydrophobic block A. At pH 5.6, block A is now more hydrophilic (cf. Figure ), and only bl CP1-H copolymer, which contains the least amount of CEtOxa units, forms large macromolecular aggregates and the transmittance percentage is significantly reduced. The samples bl CP2-H and bl CP3-H do not show a really temperature-dependent reduction in their solution transmittance. It can be interpreted that bl CP2-H and bl CP3-H acquire a significant amphiphilic character due to their relative high content of polar hydrolyzed polyMEtOxa segments (cf. Table ), and thus micelles and nano-sized aggregates could be formed that lead only to a very small decrease in transmittance. At pH 12, the influence of block A predominates the phase transition of block copolymers and it comes again to no transmission change with temperature. Thus, compared to the statistical copolymers, the block copolymers show similarities in their LCST behavior in the hydrolyzed form which indicates, as already discussed above, that a rather blocky structure is obtained also when both monomers are added at the same time due to the differences in their reactivity. Still, the slight dependency in the Ttr of the statistical copolymers from the monomer composition, which is not observed for the block copolymers, gives evidence that probably only a gradient copolymer is achieved when both monomers are added simultaneously.
4. Conclusions
It is shown that pH- and temperature-responsive polymers can be synthesized through statistical and block copolymerizations from 2-cyclopropyl-2-oxazoline and 2-methoxycarbonylethyl-2-oxazoline monomers initiated by 1,4-dibromobutene and subsequent hydrolysis of the ester units of MEtOxa. The polymers exhibit defined phase transition temperatures as function of their monomer composition, order of the monomers inside the polymeric structure, and acidity of the media (pH).
The A–B–A block copolymers in the hydrolyzed form show an amphiphilic character at pH 5.6 and pH 12, leading to the formation of micelles or nanoaggregates, whose specification is dependent on the ratio of the temperature-sensitive middle block B and the pH-sensitive outer block A. Most likely, also in the case of the statistical copolymers, a gradient or blocky like structure and thus a certain amphiphilicity is obtained due to the difference in the monomer reactivity. This leads to the fact that also in the hydrolyzed form, mostly no phase transition is observed at neutral or basic pH in the turbidity measurements, probably due to the formation of nanoscale agglomerates.
All statistical and block copolymers in the ester form show Ttr in the physiological range between 30 and 36 °C which are only slightly dependent on the monomer ratio for the statistical copolymers (increasing from 31 to 36 °C with increase in MEtOxa from 8.9 to 21.5 mol%). A temperature-dependent transmission change due to macroaggregation is only observed for all hydrolyzed (block) copolymers at pH 1 when all COOH groups are protonated, and only for the st and bl CP1 with the lowest HEtOxa content at pH 5.6 only. In the latter case, the Ttr is significantly increased by about 32 °C to above 53 °C.
Our synthetic approach based on 2-oxazolines is able to form T- and pH-sensitive copolymers with exact monomer composition and also well-defined polymeric structure, and thereby controllable sensitive behavior. Especially, the block copolymers have a great potential in the amphiphilic state to form, by self-assembly, smart nanoparticles for many applications.
Acknowledgments
The authors gratefully acknowledge the Research Department (DGI) of Catholic University Peru (PUCP) and the research project PUCP-DGI-2032 (synthesis and characterization of new commutable polymers) for their financial support. The work was further related to the Center of Excellence cfaed at TU Dresden. The help of C. Harnisch of IPF for SEC measurements is gratefully acknowledged.
Disclosure statement
No potential conflict of interest was reported by the authors.
Additional information
Funding
References
- Miyata T, Uragami T. In: Dumitriu S, editor. Polymeric biomaterials. 2nd ed. New York (NY): Marcel Dekker; 2002. p. 959–974.
- Langer R, Tirrell DA. Designing materials for biology and medicine. Nature. 2004;428:487–492.10.1038/nature02388
- Schild HG. Poly(N-isopropylacrylamide): experiment, theory and application. Prog. Polym. Sci. 1992;17:163–249.10.1016/0079-6700(92)90023-R
- Viegas TX, Bentley MD, Harris JM, et al. Polyoxazoline: chemistry, properties, and applications in drug delivery. Bioconjugate Chem. 2011;22:976–986.10.1021/bc200049d
- Uyama H, Kobayashi S. A novel thermo-sensitive polymer. Poly(2-iso-propyl-2-oxazoline). Chem. Lett. 1992;21:1643–1646.10.1246/cl.1992.1643
- Diab C, Akiyama Y, Kataoka K, Winnik F. Microcalorimetric study of the temperature-induced phase separation in aqueous solutions of poly(2-isopropyl-2-oxazolines). Macromolecules. 2004;37:2556–2562.10.1021/ma0358733
- Park JS, Akiyama Y, Winnik F, Kataoka K. Versatile synthesis of end-functionalized thermosensitive poly(2-isopropyl-2-oxazolines). Macromolecules. 2004;37:6786–6792.10.1021/ma049677n
- Hoogenboom R, Schlaad H. Bioinspired poly(2-oxazoline)s. Polymers. 2011;3:467–488.10.3390/polym3010467
- Huber S, Jordan R. Modulation of the lower critical solution temperature of 2-alkyl-2-oxazoline copolymers. Colloid Polym. Sci. 2008;286:395–402.10.1007/s00396-007-1781-y
- Huber S, Hutter N, Jordan R. Effect of end group polarity upon the lower critical solution temperature of poly(2-isopropyl-2-oxazoline). Colloid Polym. Sci. 2008;286:1653–1661.10.1007/s00396-008-1942-7
- Bloksma MM, Weber C, Perevyazko IY, et al. Poly(2-cyclopropyl-2-oxazoline): from rate acceleration by cyclopropyl to thermoresponsive properties. Macromolecules. 2011;44:4057–4064.10.1021/ma200514n
- Glassner M, Lava K, de la Rosa VR, Hoogenboom R. Tuning the LCST of poly(2-cyclopropyl-2-oxazoline) via gradient copolymerization with 2-ethyl-2-oxazoline. J. Polym. Sci., Part A: Polym. Chem. 2014;52:3118–3122.10.1002/pola.v52.21
- Obeid R, Maltseva E, Thünemann A, Tanaka F, Winnik F. Temperature response of self-assembled micelles of telechelic hydrophobically modified poly(2-alkyl-2-oxazoline)s in Water. Macromolecules. 2009;42:2204–2214.10.1021/ma802592f
- Kuckling D, Richter A, Arndt KF. Temperature and pH-dependent swelling behavior of poly(N-isopropylacrylamide) copolymer hydrogels and their use in flow control. Macromol. Mater. Eng. 2003;288:144–151.10.1002/mame.200390007
- Schmaljohann D, Oswald J, Jørgensen B, Nitschke M, Beyerlein D, Werner C. Thermo-responsive PNiPAAm-g-PEG films for controlled cell detachment. Biomacromolecules. 2003;4:1733–1739.10.1021/bm034160p
- Kobayashi S, Uyama H. Polymerization of cyclic imino ethers: from its discovery to the present state of the art. J. Polym. Sci., Part A: Polym. Chem. 2002;40:192–209.10.1002/(ISSN)1099-0518
- Dimitrov I, Trzebicka B, Müller A, Dworak A, Tsvetanov C. Thermosensitive water-soluble copolymers with doubly responsive reversibly interacting entities. Prog. Polym. Sci. 2007;32:1275–1343.10.1016/j.progpolymsci.2007.07.001
- Aoi K, Okada M. Polymerization of oxazolines. Prog. Polym. Sci. 1996;21:151–208.10.1016/0079-6700(95)00020-8
- Hoogenboom R, Fijten MWM, Schubert US. Parallel kinetic investigation of 2-oxazoline polymerizations with different initiators as basis for designed copolymer synthesis. J. Polym. Sci., Part A: Polym. Chem. 2004;42:1830–1840.10.1002/(ISSN)1099-0518
- Rueda J, Suica R, Komber H, Voit B. Synthesis of new polymethyloxazoline hydrogels by the “macroinitiator” method. Macromol. Chem. Phys. 2003;204:954–960.10.1002/macp.200390065
- Rueda J, Komber H, Cedrón JC, Voit B, Shevtsova G. Synthesis of new hydrogels by copolymerization of poly(2-methyl-2-oxazoline) bis(macromonomers) and N-vinylpyrrolidone. Macromol. Chem. Phys. 2003;204:947–953.10.1002/macp.200390062
- Hoogenboom R, Thijs H, Jochems M, van Lankvelt B, Fijten M, Schubert US. Tuning the LCST of poly(2-oxazoline)s by varying composition and molecular weight: alternatives to poly(N-isopropylacrylamide)? Chem. Commun. 2008;44:5758–5760.10.1039/b813140f
- Rueda J, Zschoche S, Komber H, Schmaljohann D, Voit B. Synthesis and characterization of thermoresponsive graft copolymers of NIPAAm and 2-alkyl-2-oxazolines by the “grafting from” method. Macromolecules. 2005;38:7330–7336.10.1021/ma050570p
- Zschoche S, Rueda J, Komber H, et al. Thermo-responsive nanogels based on poly[NIPAAm-graft-(2-alkyl-2-oxazoline)]s crosslinked in the micellar state. Macromol. Chem. Phys. 2010;211:1035–1042.10.1002/macp.200900559
- Rueda J, Zschoche S, Komber H, Krahl F, Arndt K-F, Voit B. New thermo-sensitive graft copolymers based on a poly(N-isopropylacrylamide) backbone and functional polyoxazoline grafts with random and diblock structure. Macromol. Chem. Phys. 2010;211:706–716.10.1002/macp.v211:6
- Zschoche S, Rueda J, Binner M, et al. Reversibly switchable pH- and thermoresponsive core–shell nanogels based on poly(NiPAAm)-graft-poly(2-carboxyethyl-2-oxazoline)s. Macromol. Chem. Phys. 2012;213:215–226.10.1002/macp.201100388
- Weber C, Wagner M, Baykal D, et al. Easy access to amphiphilic heterografted poly(2-oxazoline) comb copolymers. Macromolecules. 2013;46:5107–5116.10.1021/ma400947r
- Rossegger E, Schenk V, Wiesbrock F. Design strategies for functionalized poly(2-oxazoline)s and derived materials. Polymers. 2013;5:956–1011.10.3390/polym5030956
- Guillerm B, Monge S, Lapinte V, Robin JJ. How to modulate the chemical structure of polyoxazolines by appropriate functionalization. Macromol. Rapid Commun. 2012;33:1600–1612.10.1002/marc.201200266
- Lava K, Verbraeeken B, Hoogenboom R. Poly(2-oxazoline)s and click chemistry: a veratile toolbox toward multi-functional polymers. Eur. Polym. J. 2015;65:98–111.10.1016/j.eurpolymj.2015.01.014
- Bouten PJM, Hertsen D, Vergaelen M, et al. Synthesis of poly(2-oxazoline)s with side chain methyl ester functionalities: detailed understanding of living copolymerization behavior of methyl ester containing monomers with 2-alkyl-2-oxazolines. J. Polym. Sci., Part A: Polym. Chem. 2015. doi:10.1002/pola.27733.
- Bouten PJM, Hertsen D, Vergaelen M, et al. Accelerated living cationic ring-opening polymerization of a methyl ester functionalized 2-oxazoline monomer. Polym. Chem. 2015;6:514–518.10.1039/C4PY01373E
- Zarka T, Nuyken O, Weberskirch R. Amphiphilic polymer supports for the asymmetric hydrogenation of amino acid precursors in water. Chem. Eur. J. 2003;9:3228–3234.10.1002/chem.200304729
- Witte H, Seeliger W. Cyclische Imidsaeureester aus Nitrilen und Aminoalkoholen [Cyclic imidic esters by reaction of nitriles with amino alcohols]. Liebigs Ann. Chem. 1974;1974:996–1009.10.1002/(ISSN)1099-0690
- Christova D, Velichkova R, Goethals EJ. Bismacromonomers of 2-alkyl-2-oxazolines – synthesis and polymerization. Macromol. Rapid Commun. 1997;18:1067–1073.10.1002/marc.1997.030181210
- Weber C, Becer CR, Guenther W, Hoogenboom R, Schubert US. Dual responsive methacrylic acid and oligo(2-ethyl-2-oxazoline) containing graft copolymers. Macromolecules. 2010;43:160–167.10.1021/ma902014q