Abstract
A series of poly (2-hydroxyethylmethacrylate-graft-folic acid) (FAHEMA) systems are synthesized by grafting of folic acid (FA) into poly (2–hydroxyethylmethacrylate) via an esterification reaction. The structure of these copolymers is confirmed by NMR and CHN analyses. The thermal behavior of these materials is characterized by DSC and TGA analyses. The surface morphology of FAHEMA films before and after the release process is examined by the SEM method. The cumulative FA released in different pH media from FAHEMA materials occurred via a retro-esterification reaction at body temperature during 72 h in which the influence of the swelling degree of PHEMA, the FA content and pH media on the dynamic release is widely investigated. The results obtained revealed that the solubility of FA in water deduced from the release process is widely improved compared with literature reports. It is also revealed that the diffusion of water in different pH media through the PHEMA matrix and that of FA through FAHEMA materials perfectly obeyed the Fickian models. It was deduced from the kinetic study that the release performance is obtained with the copolymers containing initially 10 and 20 wt% of FA contents.
Introduction
The solubility of drugs in water is considered as a principal key in order to reach therapeutic plasma concentrations after oral administration, therefore, enhancement of their dissolution rates is necessary. More than 40% of newly discovered drugs are water-insoluble or poorly soluble, which present a serious challenge to the success of new drugs development. During the two last decades several literature reports dealt with such a problem. In fact, to enhance the solubility of drugs, Hancock [Citation1] and Grau [Citation2] used the physical reduction of the drug particles sizes, which led to increase their surface areas and/or the generation of amorphous states. Indeed, according to different investigators, the smaller the drug particles size, the higher is the solubility.[Citation3–8] Solid dispersion method has also been employed by different investigators to increase the drug-water solubility. This technique applied on sulfathiazole [Citation9] and chloramphenicol [Citation10] by Sekiguchi led to promising results. Folic acid (FA) also called Folcacin is one of the important drugs containing three important active groups such as pteridine ring, para-aminobenzoique acid, and glutamic acid (Scheme ), is a vitamin poorly water-soluble (1.6 μg mL−1) at 25 °C.[Citation11]
This medication is essential in human diet, necessary for maintenance in growth and production of new cells. This substance is also necessary and important for rapid cell division and growth periods such as pregnancy and infancy. So it is often recommended to take specific dosages of FA on daily basis. Folic acid and conjugated derivatives combine with folate receptor grafted on the surface of cancer cells are internalized to intracellular compartments to form endosomes.[Citation12,13] According to different researchers,[Citation14,15] the conjugation between folates receptors and conjugates separate in acidic media (pH 5 at 5.5) converted after dissociation to be released in the cytosol of cell surface or degrades by liposome. Biodegradable and biocompatible polymers used as drug support have received a considerable attention in the last two decades. Thermoresponsive hydrogels have recently become more attractive particularly in the biomedical domain; its use included controlled drug delivery of which the poly(2-hydroxyethylmethacrylate)(PHEMA) is a typical one.[Citation16–18] PHEMA is a linear polymer composed of very active hydroxyester units distributed uniformly on the polymeric chain. This polymer is considered as one of the most important hydrophilic polymers, biodegradable, and nontoxic material.[Citation19–22] In certain case, this polymer is considered as a living tissue, inertness to biological metabolisms, permeable to metabolites, resistant to absorption by the body and can easily be manufactured into many shapes and forms.[Citation23,24] PHEMA have been widely employed in different domains as medicine, biotechnology, chemical, food, feed, agriculture, and environment protection.[Citation25–31] Dilmi et al. [Citation32] investigated on the ibuprofen release kinetics from hydrogels based on the 2-hydroxyethylmethacrylate and chitosan as drug carrier. The data obtained revealed that the swelling process was controlled by second-order kinetics, while the ibuprofen diffusion through the polymer matrix obeyed to the Fickian model. Başbağ et al. [Citation33] have prepared three types of delivery polymer systems based on (2-hydroxypropyl)-β-cyclodextrin (HPβCD) and 2-hydroxyethyl methacrylate (HEMA). The resulting materials are poly(HPβCD-co-HEMA) hydrogels containing different HPβCD contents. It is observed that, in presence of HEMA, hydrogels have acquired mechanical integrity with lower equilibrium content than that of hydrogels without HEMA. Cyclosporine A (CsA) used as a drug is loaded into the HPβCD-based hydrogels using the suspension technique. It is revealed that the poly(HEMA) hydrogels have released practically the total CsA amount initially incorporated in these materials during three days of the release process, while, a long-term release is realized from HPβCD-based hydrogels. It is also observed that, for sub-conjunctival administration, regarding the loaded CsA amounts, release profiles, and mechanical integrity, the most suitable system is poly(HEMA-co-HPβCD) hydrogels containing high HPβCD content. Kumar et al. [Citation34] investigated the encapsulation of hydrophobic drug as curcumin in hydrophilic polymeric core as PHEMA using the nanoprecipitation method to improve its efficacy. The anticancer activity of curcumin-PHEMA nanoparticles system estimated in the ovarian cancer cells regression (SKOV-3) revealed that, this drug–polymer system had better tumor cells regression activity compared with that obtained using the free curcumin. The toxicity of PHEMA nanoparticles studied in zebrafish embryo model revealed that the material was biocompatible and the in vitro release study revealed that, the curcumin-loaded PHEMA nanoparticles have potential therapeutic values in cancer treatment. In this same subject, recently Sudhakar et al. [Citation35] reported an investigation on the release of this pharmacon from a 2-hydroxyethylmethacrylate copolymerized with N-vinylcaprolactam used as drug carrier and the results obtained revealed a formation of nanogels in a spherical shape, with the size of 150 nm and indicated that the nanoparticles may be used for targeted drug delivery.
Grafting the FA on PHEMA through a catalytic esterification reaction to prepare a new smart material capable to release uniformly adequate amount of folic acid as long as possible by retro-esterification reaction can be a solution to resolve the inconvenience associated with the drug release technique. Among the advantages of this method are to achieve optimum concentrations, prolong time, reduce the side effects, enhance the drug activity and control the dynamic release – pH relation. In this investigation a series of poly(HEMA-graft-FA) (FAHEMA) is synthesized via an esterification reaction and characterized by different methods such as NMR, CHN, DSC, XRD, and SEM. The dynamic release of FA through a retroesterification reaction in different pH media and at different FA contents is widely investigated. The solubility of FA in the media and the diffusion behavior in the polymer matrix are also investigated.
Experimental
Materials
FA is supplied by Riyadh Pharma Company and used without purification. 2-hydroxyethylmethacrylate (HEMA) (Sigma Aldrich, 98% purity) was distilled under reduced pressure and kept at −20 °C under nitrogen atmosphere. Dimethyl sulphoxide (LOBA Chemie, purity 99%) is used without further purification. Azo-bis-isobutyronitrile (AIBN) (Aldrich, 98% purity) was purified by recrystallization in methanol.
Preparation of PHEMA
PHEMA is synthesized by bulk polymerization of HEMA through a free radical reaction at 70 °C during 30 min under nitrogen atmosphere using AIBN as initiator and the preparation conditions is summarized in Table .
Table 1. Preparation conditions of PHEMA and FAHEMA.
Preparation of FAHEMA
FAHEMA copolymer is synthesized through a catalytic esterification reaction between PHEMA and FA according to Scheme . A solution containing a well-defined FA amount in DMSO is added dropwise to the precedent reactor containing the resulted polymeric solution at the end of the polymerization of HEMA. The esterification reaction of PHEMA with FA carried out at 60 °C in presence of 0.5 ml of concentrated sulfuric acid. Water produced during the esterification reaction is removed by evaporation under reduced pressure using a vacuum pump. In this case, the equilibrated reaction would be forced to produce only the ester. A thick and very viscous solution is obtained from this reaction indicating that the poly (PHEMA-graft-FA) copolymer is produced. Residual FA is removed by washing the copolymer obtained several times with DMSO until the washing solution became colorless. The as prepared polymer and copolymers is left at ambient temperature for 24 h then dried under vacuum at 80 °C in a vacuum for 2 h. Noting that, the polymer and copolymers specimens heated at this temperature become water-insoluble, however, it swells in the media leading to a hydrogel – forming. A series of FAHEMA copolymers containing 5, 10, 15, and 20 wt% FA contents are prepared by the same method and their preparation conditions are given in Table .
Equipment and methods
The average number molecular weights of the prepared PHEMA and FAHEMA with different FA contents were estimated in DMSO at 60 °C by size exclusion chromatography (SEC) on a Varian apparatus. This instrument is equipped with a JASCO-type 880-PU HPLC pump with a flow rate of 1.0 ml, refractive index and UV detectors and Shodex GPC KF-806 M (8.0 mm I.D. × 300 mm) columns calibrated with polystyrene standards and the results obtained are summarized in Table .
Table 2. Characterization of FAHEMA copolymers by CHN and 1HNMR methods.
1H NMR and 13C NMR analysis of FA, PHEMA, and FAHEMA copolymers containing different FA contents are performed on a JEOL FX 90 Q NMR apparatus at 500 and 200 MHz, respectively in DMSO-d6 at room temperature. The composition of 2-folatethylmethacrylate comonomer (FEMA) in FAHEMA systems are determined by CHN elemental analysis using Perkin Elmer PE 2400 Series II apparatus and the results of FAHEMA characterization are grouped in Table . The FA amounts released from the synthesized materials are determined by UV–Vis Aultropec 2100 pro, Amersham Biosciences spectroscope at 281 nm using FA calibration curve. The release experiments are carried out at 37 °C in pH media of 1, 3, 5, and 7. The melting points (Tm) of FA and the glass transition temperatures (Tg) of PHEMA homopolymer and FAHEMA copolymers are performed on a DSC (Shimadsu DSC 60) apparatus, previously calibrated with indium. Samples weighing between 10 and 12 mg are packed in aluminum DSC pans before placing in DSC cell. The samples are heated from −50 to 200 °C at a heating rate of 20 °C min−1. For the surface morphology analysis, scanning electron microscopic (SEM) micrographs of dried thin films of FA, PHEMA, and FAHEMA films coated with gold grid are obtained using JSM-6060LV (JEOL). X-ray measurements of FA, PHEMA, and FAHEMA copolymers with different FA contents are collected from a BRUKER D8 advance diffractometer (Germany). The patterns were run with Cu Kα radiation at 40 mA and 40 kV with scanning speed in 2θ of 2° min−1.
The cytotoxicity test of pure PHEMA and HEMAFA materials is realized by MITT assay on L929 fibroblastic cell lines for cell toxicity evaluation according to Mosmann method.[Citation36] These cells are placed in Dulbecco’s modified Eagle’s medium (DMEM) complemented with 10% of fetal bovine serum (FBS) placed in an incubator maintained in a humidified atmosphere containing 5 wt% of CO2 at body temperature. The cells are trypsinized during 4 min. The cells cultured are incubated with different concentrations of PHEMA and HEMAFA system in DMEM/FBS solution ranged between 10.0 and 100 μg mL−1 for 24 h. The cells obtained from this step are then washed with PBS and stained with 50 μL of MITT solution. After 4 h, the medium is separated and the solution is measured at 570 nm using a microplate reader (Statfax 2100). The percentages of viability and toxicity are evaluated by Equation (1) and Equation (2), respectively [Citation37](1)
in which the toxicity is deducted from the following relationship:(2)
where DT and DC are the optical density of test and that of control, respectively.
In vitro drug release
FAHEMA film samples were immersed in defined pH media for 2 h which corresponded to the maximum swelling of PHEMA and dissolution of any FA traces deposited on the surface. The effective FA/PHEMA composition is obtained from a simple subtraction of the FA amount dissolved during the washing time (2 h) from that initially incorporated in the PHEMA matrix. FAHEMA films are placed in 15 ml of water/hydrochloric acid solution at a fixed pH and stirred at 130 rpm and 37 °C (body temperature). This experimentation is performed at pH 1, 3, 5, and 7. The film sample immersed in defined pH media is taken to a maximum swelling and dissolution of any FA traces deposited on the surface. Noting that the time and the FA amount dissolved in the media during this step is not taken in consideration in the cumulative FA-released calculation and the real FA/PHEMA composition was corrected by a simple subtraction of the FA amount dissolved from that initially incorporated in the PHEMA matrix. Aliquots of 0.5 ml are then withdrawn at time intervals. To prevent the concentration equilibrium between FA in the polymer matrix and FA in the media, the solution is immediately changed after each measurement. This operation is kept at constant volume of media and ensured a continuous release of FA during all the release process. The total mass of FA released during a definite duration is calculated from the standard absorbance curve obtained by UV analysis as mentioned above. It is important to note that during the release process the pH of water is practically not affected by the small amount of FA released because the amount of FA released is negligible compared to those of the media, however, FA is a weak acid (pKa1 = 4.7), therefore the addition of a buffer solution to water is not necessary. It is important to note that no polymer or other substances which can be produced during the release process were deposed on the bottom of the reactor except the FA released which its structure was proved by 1HNMR and its melting temperature by DSC analysis.
Results and discussion
Characterization
The poly (HEMA-graft-FA) structure is confirmed by 1HNMR analysis through the comparison of the FAHEMA spectrum with those of pure PHEMA and FA presented in Figure . Indeed, in addition to the absorption signals attributed to both FA and PHEMA observed on the FAHEMA spectra, the intensity reduction of the signal (e) localized at 4.70 ppm is attributed to the hydroxyl proton assigned to the 2-hydroxyethylmethacrylate unit, while an increase in the signal at 3.81 ppm was attributed to the four protons (c + c′) of the two ester methylene groups (–CO–O–CH2–) of the 2-folateethylmethacrylate ester unit.
The comparison of the FAHEMA 13C NMR spectra with those of the pure PHEMA and FA as shown for FAHEMA20 in Figure indicates the presence of HEMA unit and FA substituent in the copolymer synthesized. The structure of FAHEMA material is confirmed by the increase of the peak localized at 61.21 ppm assigned to the carbon (d) linked to the oxygen ester (–CH2–O–CO–) of the folate substituent and also by the intensity decrease in the peak at 57.3 ppm attributed to the carbon (e) directly linked to the hydroxyl group (HO–CH2–) of HEMA unit in the copolymer.
The molar fraction of 2-folateethylmethacrylate comonomer (FEMA) in the copolymers, determined previously by CHN analysis, is confirmed by 1H NMR using the surface area of the two equivalent ethenyl protons (11), , in FEMA structure centered at 2.27 ppm and those of the 3 protons (a) of the methyl group,
, common to the two comonomers localized between 0.40 and 1.00 ppm using Equation (3).
(3)
The results of the molar fractions of FA obtained from the 1HNMR data and Equation (3) grouped in Table perfectly agreed with those previously obtained by the CHN method.
The DSC thermograms of PHEMA, FA, and FAHEMA with different FA contents are gathered in Figure . On the light of these curve profiles, a shift in the Tg value towards the high temperatures (from 50 to 57 °C) is observed in the thermograms of FAHEMA copolymers compared with that of the corresponding homopolymer. The increase in Tg is probably caused by a reduction of the chains sliding due to the sterically hindered folate grafted on PHEMA chains. As shown by the thermogram of FAHEMA containing 20 wt% of FA content, the transition showed at 110 °C is probably attributed to a shift in the melting temperature of the residual free FA aggregated and homogeneously distributed in the copolymer matrix. Noting that the results obtained by 1H and 13C NMR confirmed the presence of a small amount of FA none grafted incorporated in the copolymer materials. The shift in the glass transition temperature towards the right is probably caused by the inter-chains reaction between some FAHEMA chains in acidic media during the preparation process. Indeed, this fact assists the formation of a weakly cross-linked polymer leading to a reduction in the chains slide and consequently leads to an increase in the Tg value. However, the shift in the melting temperature attributed to the free FA aggregated into the copolymer matrix is generally caused by the slow heat transfer into the polymer material.
XRD analysis performed on the pure PHEMA, FA, and FAHEMA with different FA contents have led to the spectra of Figure . The XRD pattern of FA revealed a multitude of absorption peaks in which the principals are centered at 5.5, 11.0, 13.5, 22.0, 27.0, and 29.0 two-theta characterizing its crystalline structure, which perfectly agreed with those of the literature.[Citation38,39] While that of PHEMA is devoid of any signal indicating a crystalline structure. The FAHEMA samples with different FA contents revealed in their spectra a total disappearance of the peaks attributed to the crystalline structure of FA. However, new signals in which the principal peaks appeared at 34.0, 41.0, 48.0, 53.5, and 54.5 two-theta confirm the presence of free FA aggregated in another crystalline system distributed uniformly in the copolymer matrix. Indeed, folic acid has an extended conformation caused by the pteridine ring in which the equilibrium between the ketonic and enolic forms (Scheme ) can lead to different crystalline systems.
Figure 5 Cytotoxycity study of FAHEMA drug-carrier system with different FA contents using the MTT assay.
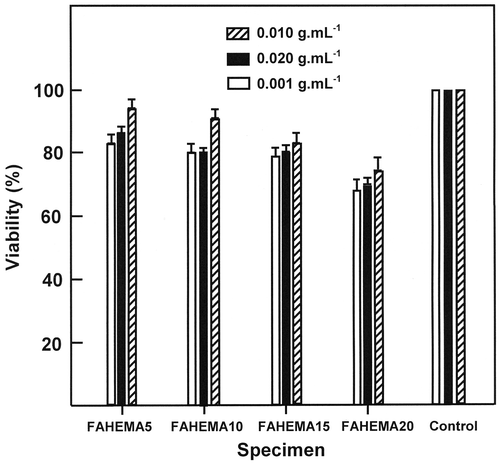
Figure 9. SEM micrographs of pure PHEMA and FAHEMA10 film before and after the release process in media pH 1 and 7, respectively.
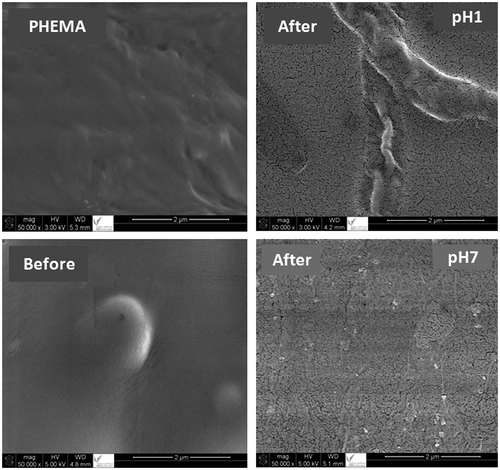
Figure 10. Variation of the diffusion coefficient (D) of FA through the FAHEMA film with different FA contents vs. t−1 at different and pH media.
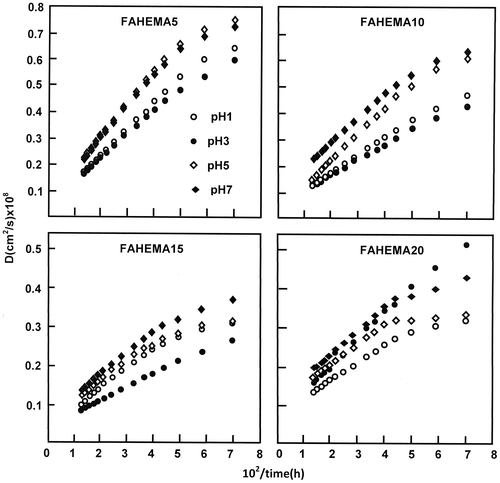
Figure 11. Variation of the cumulative FA released from FAHEMA systems vs. the swelling degree of PHEMA at equilibrium.
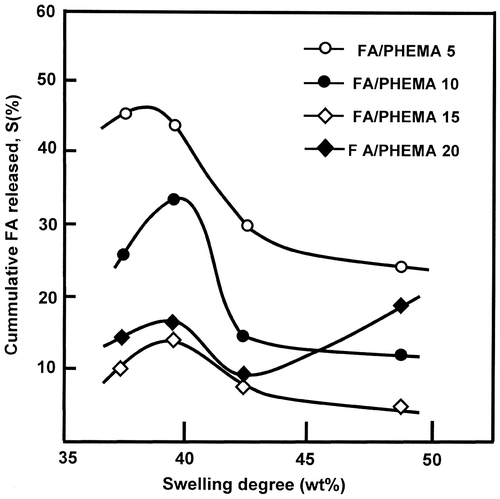
Figure 12. Influence of FA content in FAHEMA films on the dynamic release at different pH media during 10 and 24 h.
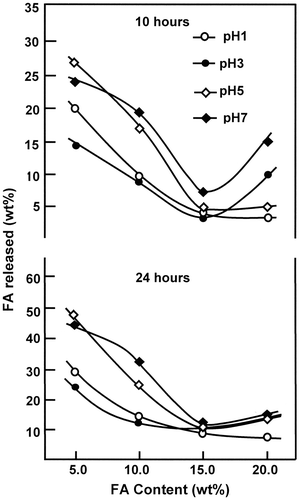
Figure 13. Effect of pH media on the release dynamic of FA from FAHEMA films with different FA contents during 10 and 24 h.
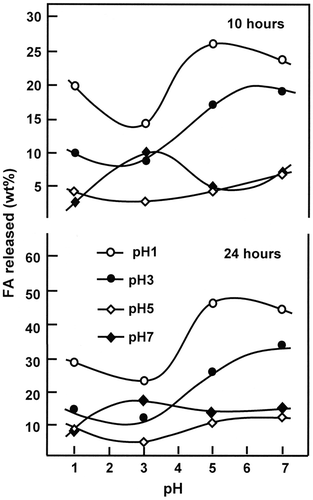
The cytotoxicity activity of the FAHEMA drug-carrier system with different FA content is presented in Figure . As it can be seen from these diagrams which show a viability superior to 68% for all concentrations range (0.010–0.001 g ml−1) indicating that all specimens are out of the toxic level.[Citation40,41] These results also reveal that the percentage of viability slowly decreases as the FA content grafted in the PHEMA increases and the best viability was observed at concentration of 0.010 g ml−1.
Swelling behavior of PHEMA
The swelling study of a polymer hydrogel in water is indispensable to understanding the diffusion of drug-water solution through a material used as carrier in the drug delivery domain. Indeed, Wan et al. [Citation42] who used the hydroxypropylmethylcellulose (HPMC) as a hydrophilic drug support revealed that an inverse relationship exists between the drug release rate and matrix swelling rate. The swelling behavior of HPMC is therefore useful in predicting drug release. In this investigation, a dried PHEMA film was immersed in defined pH media maintained at 37 °C under stirring for 4 h corresponding to the maximum swelling of PHEMA. Noting that, at the end of the swelling process, no traces of polymer were observed after removing the specimen and evaporation of water (media). This preliminary test indicates that this polymer is not soluble in water. However, it swells in the media leading to a hydrogel – forming. The capability test of PHEMA hydrogel to swell in different pH media evaluated using Equation (4).(4)
where wo and wt are the weight of the film sample before and at t time of the swelling process, respectively. S (%) is the swelling degree of PHEMA in different media. Noting that after specimen withdrawing and water (media) evaporation no residual polymer was observed at the bottom of the beaker.
On the light of the variation of the swelling degree vs. time presented in Figure it is revealed that the maximum swelling, S∞, corresponding to the equilibrium is practically reached at about the two first hours of the swelling process and the performance of PHEMA to swell in water at 37 °C is observed at pH 3 in which 49.11 ± 0.30 wt% of water is absorbed. However, only 37.59 ± 0.27 wt% is reached at pH 1 as shown in Table .
Table 3. Swelling properties of water-PHEMA system taken at different pH media and 37 °C.
The swelling kinetics is usually used to investigate the diffusion of small molecules through polymeric materials immersed into a penetrating media during a determined time, t. According to Comyn [Citation43] the kinetics that governs this dynamic is generally given by Equation (5).(5)
where Mt and M∞ are the mass of the absorbed molecules during t time and at the maximum absorption (equilibrium), respectively. l and D are the film thickness and the diffusion coefficient, respectively. For the short times of the initial stage of diffusion and when the Mt/M∞ ratio is lower than 0.5 Equation (4) takes the following Equation (6)(6)
in which D can be deduced from the slope of the linear portion of the curve corresponding to the variation of Mt/M∞ vs. square root of time. For the water /PHEMA system, Figure reveals straight lines during the first hour of the swelling process indicating that the diffusion of water molecules through the PHEMA matrix obeys the fick’s model as long as the temperature of this polymer in media (37 °C) is well above Tg (50 °C). This finding indicates that the diffusion of water through the polymer film is purely and simply governed by a mechanical process and non-disturbed by a probable esterification reaction which can occur between the alcoholic polymer and acidic media.
According to Massaro et al. [Citation44] the basic equation of mass uptake by polymer material is given by Equation (7).(7)
where n exponent is the type of diffusion mechanism and k is the constant which depends on diffusion coefficient and film thickness. By analogy with equation (3) n = 0.5 and k takes the following expression (8)(8)
As it can be seen from the D and k values reported in Table that the diffusion rate slightly decreased as the pH media increased, thus indicating a very low influence of the pH-media on the swelling process.
Solubility enhancement of FA in water
Solubility of FA in water as any other therapeutically bioactive substance is also considered as a key property in which this crucial parameter governs its dissolution in the media then its absorption by different organs. For this reason the in vivo FA efficacy depends on its solubility. FA is a crystalline drug, very slightly soluble in water (1.6 mg L−1) at 25 °C [Citation13] and its solubility varies with temperature and pH media.[Citation45–47] Hence, poorly absorbed from the gastrointestinal (GI) tract when used alone.[Citation48,49] Therefore, to enhance the solubility of FA in water constitutes one of our important objectives. Indeed, according to different investigations, the smaller the FA particle size, the higher the solubility.[Citation3–8] In this work, the maximum concentration of FA is deduced from the FA released at 37 °C in different pH media during one week. Noting that, at the end of the release process, only FA was deposed in the bottom of the Becker after removing the specimen and total evaporation of media. The 1HNMR and DSC analyses confirmed this observation through a perfect superposition of their spectra and thermograms with those of the starting FA. The data of the FA-water solubility gathered in Table reveals that the solubility of FA in the media increased with the FA content grafted, and the higher solubility is reached with FAHEMA20 in which more than 272 mg L−1 of FA is completely dissolved at pH 7 and more than 343 mg L−1 dissolved at pH 1.
Table 4. Maximum FA amount dissolved in media at different pH media during one week of the release from FAHEMA materials at 37 °C.
From these observations, it is possible to conclude that the solubility of FA in different pH media has easily gone over these values, because no precipitate is observed during this period indicating that the saturation of the FA solutions is far from being reached. From this finding, it is important to emphasize that the incorporation of FA in PHEMA by grafting through an esterification reaction could be an appropriate method to increase the solubility of FA in water without addition of a third component or using the physical techniques such as the comminution or micronization techniques based on the mechanical stress to disaggregate the active compound inducing the drug degradation which is caused by the thermal and mechanical forces such as milling and grinding.[Citation50–54] This finding is predicted because the FA released via a retro-esterification reaction is completely dispersed in water in its molecular form and not in aggregate or crystalline form. In this state, the maximum hydration of FA molecules is reached leading to the maximum solubility compared with that of Zhang et al. [Citation55] in which the addition of a low generational polyamidoamine dentrimers enhanced a maximum solubility to 200 mg L−1 at pH 5. Noting that, the folic acid in suspension investigated by Pardeike et al. [Citation39] revealed a saturation solubility of 8.96 mg L−1. Reducing the particle size of folic acid to the nanometer range by the same investigators led to an increase in the saturation solubility only to 13.77 mg L−1. It could be highlighted that the US Department of Health and Human Services announced a rule that the folic acid should be added to grain products as a nutrition fortifier to ensure 0.4 mg intake for an adult and an additional 0.2 mg for pregnant women.[Citation56,57]
In vitro study of folic acid released
The dynamic release of FA from poly(HEMA-graft-FA) copolymers films with 5, 10, 15, and 20 wt% of FA contents is investigated during 72 h at media of 1, 3, 5, and 7 pH at body temperature. The cumulative folic acid released during time is calculated from Equation (9)(9)
where mt and mo are the mass of FA released in the media during t time and at t = 0, respectively. The results obtained from this equation and the experimental data are presented by Figure . As it can be seen from the curves profiles of the pretreated specimens the percentage of FA released vs. time follows a perfect logarithmic growth except that of FAHEMA20 in which the dynamic release in media pH 7 evolutes following a perfect straight line. As it can be seen from these data the higher dynamic release is reached at pH 7 for all FAHEMA specimens during 72 h except that containing 5 wt% of FA content in which the maximum cumulative FA released is reached in pH 5. On the other hand, for all FAHEMA compositions, the lower dynamic release is observed in media pH 3 except that with 20 wt% of FA which shows a minimum cumulative FA released in media pH 1.
The comparison of the results obtained using the different FAHEMA specimens reveals that the higher cumulative FA released is obtained using the FAHEMA containing 5 and 10 wt% FA content in which more than 60 and 68 wt% of folic acid are released in media pH 5 and 7 during 72 h of the release process, respectively. This finding reflects the capacity of the media to dissolve this drug poorly soluble in water, notably when is entrapped in the PHEMA matrix. Indeed, at any pH media, for a same mass of FAHEMA an amount of 5 wt% of FA released is easily dissolved in water than 20 wt%. SEM micrographs of FAHEMA10 film taken as example before and after the release in pH media 1 and 7 are gathered in Figure . The PHEMA and FAHEMA10 images obtained before the release process show a homogeneous and a smooth surface morphology. Whereas, the images of the specimen containing 10 wt% of FA obtained after the release process showed dense interconnected deep microgrooves less than 0.2 μm in width randomly oriented and homogeneously distributed in the polymer matrix. The density and the size of these microgrooves are slightly more marked at neutral pH, thus justifying the high FA amount released in these conditions.
Diffusion behavior of FA through FAHEMA films
According to Lin et al. [Citation58], for a release less than 60 wt% of the initial amount loaded, the dynamic release followed the Fickian model for the diffusion from a polymeric film. The value of the diffusion coefficient, D, can be calculated according to the following Equation (10).[Citation59–62](10)
where l is the thickness of the film and mt, mo, and t are as defined previously.
D value is determined when the permanent regime is reached and the FA particles deposited on the material surface are totally washed. In these conditions, the curves profiles of D vs. time were meaningful and reflected exactly the dynamic of FA released in the media solution inside the material. The variation of D vs. the reverse of time calculated from the data of Figure and Equation (4) showed a straight line for all FAHEMA systems as showed in Figure . This finding indicates that the diffusion of FA through the FAHEMA films obeyed the Fickian model and also indicates that the release dynamic of FA from these films is only due to the diffusion of folic acid through the copolymer matrix. In these conditions, the permanent regime of the release process is reached. On the light of these results, it is possible to build our investigation on the second zone and in case of FAHEMA20 in pH media of 1 and 7 on the ultimate zone of the release process in which the permanent regime is reached and the release dynamic of FA is governed only by the diffusion mechanism.
Effect of the swelling degree
The variation of the cumulative FA released from FAHEMA materials taken at 10 h of the release process vs. the swelling degree of the PHEMA matrix is shown in Figure . It was revealed from the curves profiles obtained a maximum rate of FA released when the swelling degree is 39.58 wt%, then dramatically decreased and stabilized beyond. The magnitude of this summit which is common for all FA/PHEMA compositions decreased as the total FA amount in the PHEMA matrix increased. This finding revealed that the optimum conditions to obtain performing release is reached with a material containing the lower FA content (5 wt%) and a PHEMA carrier capable to swell with 39.58 wt% and not with that with the higher swelling degree (49.11 wt%). Based on the principle that stipules a higher performance is reached with a polymer carrier characterized by higher swelling parameters (Table ) are not sufficient in this case. Contrarily an inverse relationship is revealed between the FA release rate and the PHEMA film swelling rate. This phenomenon was also observed by Wan et al. [Citation42] using hydroxypropylmethylcellulose as carrier. In addition to the water–polymer interactions, other important parameters as the drug–polymer, and drug–media interactions can also orient the dynamic release of a drug from a drug–polymer system.
Effect of the FA content in FAHEMA system
The influence of the total drug content grafted on PHEMA on the FA released is carried out at different pH media during 10 and 24 h of the release process which corresponds to the average intestinal transit time (AITT) [Citation54–65] and the results obtained are gathered in Figure . As it can be seen from these curve profiles that at any pH medium and at any duration the variation of the FA released vs. the total FA content grafted has practically the same profiles, which dramatically decreased when the total FA grafted on PHEMA increased and reached a minimum at about 15 wt% of FA content, notably those at pH 5 and 7 during 24 h of the release process, except that at pH 1 in which the dynamic release continue to decrease slowly.
The dramatic decrease in the cumulative FA released could be explained by an eventual reduction of the swelling degree of the polymer matrix due to the reduction of the hydrophilicity of FAHEMA caused by an increase in the ester groups when the FA content increased. The increase in FA released from the samples containing more than 15 wt% of FA content is probably due to a supplementary dissolution of a residual FA amount (non reacted) trapped initially in the polymeric matrix.
Influence of pH media on the dynamic release of FA
The effect of pH media on the dynamic release of FA from FAHEMA systems is performed during 10 and 24 h of the release process and the results collected are illustrated in Figure . As it can be seen from these curve profiles that, at any duration, the variation of the cumulative FA released from FAHEMA samples in the same way evolved with the pH media and the FA content grafted on PHEMA. In general, the percentage of FA released from FAHEMA systems containing FA amount inferior or equal to 15 wt% passed by a minimum at pH 3 and increased beyond. This phenomenon is probably due to a competition between an esterification and a retro-esterification reactions (Scheme ) in which the higher percentage of FA released is justified by the retro-esterification (pass 2), which is favored at neutral pH media. While at acidic media the esterification reaction (pass 1) is favored leading to the FAHEMA regeneration. The slight decline in the FA released observed with FAHEMA5 and FAHEMA15 when pH media increased from 1 to 3 seemed to be due to the decrease in the solubility of the free FA initially entrapped in the copolymer matrix, bearing in mind that the solubility of FA increased as the pH media decreased in the acidic range. Whereas, the cumulative FA released from FAHEMA20 which contained the highest rate of FA grafted evolved with the pH media following a totally different behavior compared with those containing an inferior FA content. The percentage of FA amount released from this material passed through a maximum at pH 3 then decreased beyond. The sudden drop of the FA released at pH above 3 is probably due to the decrease in the swelling degree of the material due to the decrease in its hydrophylicity caused by the increase in the folic ester group substituted. At pH 1 in which the esterification reaction is favored. The cumulative FA released was minimal and results mostly by the dissolution of the residual free FA entrapped in polymer matrix.
Kinetic study of released FA
The instantaneous release rates of FA liberated from FAHEMA systems are determined from the slopes of the linear portions of the curves indicating the variation of the cumulative FA released vs. time of Figure and the results obtained are gathered in Table . From these data, it was revealed in all samples, the presence of two principal stable zones of the release rate except that of FAHEMA containing 20 wt% of FA in which only a single zone was observed at pH 1 and 7. The first one is relatively short; varying between 6 and 28 h depending on the initial FA content grafted on PHEMA and the pH media is characterized by a relatively high release rate which is probably due to the rapid dissolution of the first free FA molecules liberated from the retro-esterification reaction of the external macromolecules on the surface of the specimens.
Table 5. Stability zones of instantaneous rate of FA released from FAHEMA at 37 °C and different pHs.
This phenomenon is also reported by different authors using other drug-carrier systems as poly (lactic acid)/drugs composites by Taranti et al. [Citation66], and by Zilberman [Citation67] using Dexamethasone-loaded bioresorbable films which attributed the first zone to different reasons: (i) drug particles embedded on the external surface of granules due to imperfect encapsulation and/or (ii) the solubility. The second zone is relatively long and slower, varying between 32 and 65 h also depending on the initial FA content grafted and the pH media is attributed to the FA liberated inside the specimens according to a mechanism based on a retro-esterification reaction of FAHEMA copolymer followed by a diffusion of the released drug through the polymer matrix. On the other hand, as mentioned previously, FAHEMA 20 which contained 20 wt% of FA presents an ultimate stable zone at pH 1 and 7 throughout the lifespan of the release process. Basing on the criteria, higher cumulative FA released in neutral pH, longer time, adequate release rate, it was revealed that the FAHEMA containing 10 wt% of FA grafted has the best performance. Indeed, this specimen is capable to release the higher cumulative FA (54 wt %) at neutral pH uniformly with a release rate of 0.92 during the longer time (59 h). On the other hand, this same specimen released only 24 wt% of FA at pH 1 with a release rate of 0.33 wt%/h during practically the same period. The second position is occupied by FAHEMA containing 20 wt% of FA which is capable to release uniformly 47.0 wt% of FA with a release rate of 0.65 wt%/h during 72 h. However, this same material released only 10 wt% of FA uniformly with a release rate of 0.15 wt%/h during practically the same time.
Conclusions
It could be concluded from this investigation that the incorporation of drugs containing carboxylic groups such as FA that is slightly soluble in water by grafting on the polymer containing reactive hydroxyl groups via an esterification reaction could be an appropriate method that can be used in drug delivery domain. Indeed, this method leads to the best distribution of FA in its molecular state in the polymer matrix and by this way considerably increases the water–drug contact and widely favored its solubility in the media. Indeed, the solubility test of FA in water estimated from the maximum release of FA indicates that the FAHEMA20 containing the higher FA grafted easily exceed 272 mg L−1 at neutral pH and 343 mg L−1 at pH 1 a rate that is never reached by the traditional methods. The diffusion of water through PHEMA matrix and that of FA through FAHEMA system at different pHs revealed that the diffusion behavior in the stationary regime perfectly obeyed Fickian models. The higher performance of the cumulative FA released from FAHEMA systems is obtained at pH 7 with FAHEMA containing 10 and 20 wt% of FA content. This finding seemed to be very interesting in drug release field because these systems are capable to release uniformly only a small FA amount during the stomach transit time (pH 1, duration ~2 h). However, the majority of FA amount would be uniformly released during the intestines transit time (pH 7, duration ~70 h).
Disclosure statement
No potential conflict of interest was reported by the authors.
Funding
This work was supported by King Saud University, Deanship of Scientific Research, College of Science, Research Centre.
References
- Hancock BC, Zografi G. Characteristics and significance of the amorphous state in pharmaceutical systems. J. Pharm. Sci. 2007;86:1–12.
- Grau MJ, Kayser O, Müller RH. Nanosuspensions of poorly soluble drugs-reproducibility of small scale production. Int. J. Pharm. 2000;196:155–159.10.1016/S0378-5173(99)00411-1
- Parmar N, Bagda A, Patel M, et al. Formulation strategy for dissolution enhancement of simvastatin. Int. J. pharmaceut. Sci. Res. 2012;3:3817–3822.
- Tandale P, Joshi D, Gaud RS. Formulation and evaluation of extended release solid dispersions containing simvastatin. Asian J. Biomed. Pharmceut. Sci. 2011;1:13–19.
- Premchandani TA, Barik BB. Preparation and statistical optimization of alginate based stomach specific floating microcapsules of simvastatin. Acta Pol. Pharm. Drug Res. 2012;69:751–761.
- Pandya VM, Patel JK, Patel DJ. Effect of different stabilizer on the formulation of simvastatin nanosuspension prepared by nanoprecipitation technique. Res. J. Pharm. Biol. Chem. Sci. 2010;1:910–917.
- Vyas A, Saraf S. Encapsulation of cyclodextrin complexed simvastatin in chitosan nanocarriers: a novel technique for oral delivery. J. Incl. Phenom. Macrocycl. Chem. 2010;66:251–259.10.1007/s10847-009-9605-y
- Kulhari H, Pooja D, Prajapati SK, et al. Performance evaluation of PAMAM dendrimer based simvastatin formulations. Int. J. Pharm. 2011;405:203–209.10.1016/j.ijpharm.2010.12.002
- Sekiguchi K, Obi N. Studies on absorption of eutectic mixtures. I. A comparison of the behavior of eutectic mixtures of sulphathiazole and that of ordinary sulphathiazole in man. Chem. Pharm. Bull. 1961;9:866–872.10.1248/cpb.9.866
- Sekiguchi K, Obi N. Studies on absorption of eutectic mixture. II. Absorption of fused conglomerates of chloramphenicol and urea in rabbits. Chem. Pharm. Bull. 1964;12:134–144.10.1248/cpb.12.134
- Oneil MJ. The Merck index: an encyclopedia of chemicals, drugs, and biologicals. 14th ed. Beijing: People’s Health Publishing House; 2006.
- Antony AC. The biological chemistry of folate receptors. Blood. 1992;79:2807–2820.
- Turek JJ, Leamon CP, Low PS. Endocytosis of folate-protein conjugates: ultrastructural localization in KB cells. J. Cell. Sci. 1993;106:423–430.
- Sudimack J, Lee RJ. Targeted drug delivery via the folate receptor. Adv. Drug Deliv. Rev. 2000;41:147–162.10.1016/S0169-409X(99)00062-9
- Lu Y, Low PS. Folate-mediated delivery of macromolecular anticancer therapeutic agents. Adv. Drug Deliv. Rev. 2002;54:675–693.10.1016/S0169-409X(02)00042-X
- Longenecker R, Mu T, Hanna H, et al. Thermally response 2-Hydroethylmethacrylate polymers soluble-insoluble and soluble-insoluble-soluble transitions. Macromolecules. 2011;44:993–998.
- Aguilar MR, Elvira C, Gallardo A, et al. Smart polymers and their applications as biomaterials. Topics Tissue Eng. 2007;3:1–27.
- Melnyczuk JM. Development of hydroxypropyl cellulose-filled Poly (2- hydroethylmethacrylate) semi-interpenetrating networks for drug delivery [Master of Science Thesis]. Tuscaloosa [AL]: University of Alabama; 2010.
- Horák D, Červinka M, Půža V. Hydrogels in endovascular embolization. Biomaterials. 1997;18:1355–1359.10.1016/S0142-9612(97)00059-8
- Jeyanthi R, Panduranga KR. In vivo biocompatibility of collagenpoly(hydroxyethyl methacrylate) hydrogels. Biomaterials. 1990;11:238–243.10.1016/0142-9612(90)90004-A
- Montheard JP, Chatzopoulos M, Chappard D. 2- Hydroxyethyl methacrylate (HEMA): chemical properties and applications in biomedical fields. J. Macromol. Sci. Macromol. Rev. 1992;32:1–34.
- Alam BM, Aouak T, Alandis NM, et al. Synthesis, characterization, drug solubility enhancement, and drug release study of poly (methacrylic acid- graft -simvastatin). Int. J. Polym. Mater. Polym. Biomater. 2015;64:229–241.10.1080/00914037.2014.936595
- Peppas NA. Hydrogels in medicine and pharmacy. Boca Raton (FL): CRC Press; 1987.
- Andrade JD. Hydrogels for medical and related applications. Amer. Chem. Soc. 1976;31:1–5. doi:10.1021/bk-1976-0031.
- Saini R, Bajpai J, Bajpai AK. Synthesis of poly(2-hydroxyethylmethacrylate)(PHEMA) based nanoparticles for biomedical and pharmaceutical applications. Methods Mol. Biol. 2012;906:321–328.
- Yavuz M, Baysal Z. Preparation and use of poly(hydroxylethylmethacrylate) cryogels containing L-histidine for β-casein adsorption. J. Food Sci. 2013;78:E238–E243.10.1111/jfds.2013.78.issue-2
- Khelfallah NS, Decher G, Mesini PJ. Design, synthesis and degradation studies of new enzymatically erodible poly(hydroxylethylmethacrylate)/poly(ethylene oxide) hydrogels. Biointerfaces. 2007;2:131–135.
- Karpushkin E, Dušková-Smrčková M, Remmler T, et al. Rheological properties of homogeneous and heterogeneous pol(2-hydroxyethylmethacrylate). Polym. Int. 2012;61:328–336.10.1002/pi.v61.2
- Campan R, Cazaux F, Coqueret X. Controlled swelling of poly(hydroxyethylmethacrylate) hydrogels by photochemical grafting of hydrophobic acrylates. Macromol. Mater. Eng. 2002;287:924–930.10.1002/mame.200290026
- Senshu K, Yamashita S, Mori H, et al. Time-resolved-block–isoprene in response to environment changes. Langmuir. 1999;15:1754–1762.10.1021/la980815+
- Horak D, Rittich B, Safar J, et al. Properties of RNAse a immobilized on magnetic poly(2-hydroxyethylmethacrylate) microspheres. Biotechnol. Prog. 2001;17:447–452.10.1021/bp0100171
- Dilmi A, Bartil T, Yahia N, et al. Hydrogels based on 2-hydroxyethylmethacrylate and chitosan: preparation, swelling behavior, and drug delivery. Int. J. Polym. Mater. Polym. Biomater. 2014;63:502–509.10.1080/00914037.2013.854221
- Başbağ B, Gümüşderelioğlu M, Şimşek M, et al. Poly (HEMA)/cyclodextrin-based hydrogels for subconjunctival delivery of cyclosporin A. J. Appl. Polym. Sci. 2014;131:1–9. doi:10.1002/app.40397.
- Kumar SS, Surianarayanan M, Vijayaraghavan R, et al. Curcumin loaded poly(2-hydroxyethylmethacrylate) nanoparticles from gelled ionic liquid-in vitro cytotoxicity and anti-cancer activity in SKOV-3 cells. Eur. J. Pharm. Sci. 2014;51:34–44.10.1016/j.ejps.2013.08.036
- Sudhakar K, Madhusudana Rao KM, Subha MCS, et al. Temperature-responsive poly(N-vinylcaprolacton-co-hydroxyethylmethacrylate) nanogels for controlled release studies of curcumin. Des. Monom. Polym. 2015;18:705–713.10.1080/15685551.2015.1070497
- Mosmann T. Rapid colorimetric assay for cellular growth and survival: application to proliferation and cytotoxicity assays. J. Immunol. Methods. 1983;65:55–63.10.1016/0022-1759(83)90303-4
- Petty RD, Sutherland LA, Hunter EM, et al. Comparison of MTT and ATP-based assays for the measurement of viable cell number. J. Biolumin. Chemilumin. 1995;10:29–34.10.1002/(ISSN)1099-1271
- Vora A, Riga A, Dollimore D, et al. Thermal stability of folic acid. Thermochim. Acta. 2002;392–393:209–220.10.1016/S0040-6031(02)00103-X
- Pardeike J, Daniela M, Strohmeier N, et al. Nanosuspensions as advanced printing ink for accurate dosing of poorly soluble drugs in personalized medicines. Int. J. Pharm. 2001;420:93–100.
- Chang B, Sha X, Guo J, et al. Thermo and pH dual responsive, polymer shell coated, magnetic mesoporous silica nanoparticles for controlled drug release. J. Mater. Chem. 2011;21:9239–9247.10.1039/c1jm10631g
- Manzano M, Vallet-Regí M. New developments in ordered mesoporous materials for drug delivery. J. Mater. Chem. 2010;20:5593–5604.10.1039/b922651f
- Wan LSC, Heng PWS, Wong LF. Relationship between swelling and drug release in a hydrophilic matrix. Drug Dev. Ind. Pharm. 1993;19:1201–1210.10.3109/03639049309063012
- Comyn J. Introduction to polymer permeability and the mathematics of diffusion. In: Polymer permeability. J. Comyn. Northern Ireland: Elsevier Applied Science; 1985. p. 1–10.10.1007/978-94-009-4858-7
- Masaro L, Zhu XX. Physical models of diffusion for polymer solutions. Prog. Polym. Sci. 1999;24:731–775.10.1016/S0079-6700(99)00016-7
- Wu Z, Li X, Hou C, et al. Solubility of folic acid in water at pH values between 0 and 7 at temperatures (298.15, 303.15, and 313.15) K. J. Chem. Eng. Data. 2010;55:3958–3961.10.1021/je1000268
- Taub A, Lieberman H. Stability of vitamin B12 – folic acid parenteral solutions. J. Am. Pharm. Assoc.. 1953;42:183–186.10.1002/jps.3030420402
- Hillcoat BL, Nixon PF, Blakley RL. Effect of substance decomposition on the spectrophotometric assay of dehydrofolate reductase. Anal. Biochem. 1967;21:178–189.10.1016/0003-2697(67)90179-0
- Ambike AA, Mahadik KR, Paradkar A. Spray-dried amorphous solid dispersions of simvastatin, a low Tg drug: in vitro and in vivo evaluations. Pharm. Res. 2005;22:990–998.10.1007/s11095-005-4594-z
- Cheng H, Sutton SC, Pipikin JD, et al. Evaluation of sustained/controlled-release dosage forms of 3-hydroxy-3-methylglutaryl- coenzyme A (HMG-CoA) reductase inhibitor in dogs and humans. Pharm. Res. 1993;10:683–687.
- Savjani KT, Gajjar AK, Savjani JK. Drug solubility: importance and enhancement techniques. ISRN Pharm. 2012:1–10.
- Khadka P, Ro J, Kim H, et al. Pharmaceutical particle technologies: an approach to improve drug solubility, dissolution and bioavailability. Asian J. Pharmaceut. Sci. 2014;9:304–316.
- Hancock BC, Zografi G. Characteristics and significance of the amorphous state in pharmaceutical systems. J. Pharm. Sci. 1997;86:1–12.10.1021/js9601896
- Grau MJ, Kayser O, Müller RH. Nanosuspensions of poorly soluble drugs-reproducibility of small scale production. Int. J. Pharm. 2000;196:155–159.10.1016/S0378-5173(99)00411-1
- Blagden N, de Matas M, Gavan PT, et al. Crystal engineering of active pharmaceutical ingredients to improve solubility and dissolution rates. Adv. Drug Deliv. Rev. 2007;59:617–630.10.1016/j.addr.2007.05.011
- Zhang Y, Xu MY, Jiang TK, et al. Low generational polyamidoamine dendrimers to enhance the solubility of folic acid: a “dendritic effect” investigation. Chin. Chem. Lett. 2014;25:815–818.10.1016/j.cclet.2014.02.004
- McCarthy M. USA plants to add folic acid to food products. Lancet. 1996;347:629–702.10.1016/S0140-6736(96)91228-3
- Eichholzer M, Tönz O, Zimmermann R. Folic acid: a public-health chalange. Lancet. 2006;367:1352–1361.10.1016/S0140-6736(06)68582-6
- Lin M, Wang H, Meng S, et al. Structure and release behavior of PMMA/silica composite drug delivery system. J. Pharm. Sci. 2007;96:1518–1526.10.1002/jps.20809
- Reinhard CS, Radomsky ML, Saltzman WM, et al. Polymeric controlled release of dexamethasone in normal rat brain. J. Controlled Release. 1991;16:331–339.10.1016/0168-3659(91)90010-B
- Cypes SH, Saltzman WM, Giannelis EP. Organosilicate – polymer drug delivery systems: controlled release and enhanced mechanical properties. J. Controlled Release. 2003;90:163–169.10.1016/S0168-3659(03)00133-0
- Frank A, Rath SK, Venkatraman SS. Controlled release from bioerodible polymers: effect of drug type and polymer composition. J. Controlled Release. 2005;102:333–344.10.1016/j.jconrel.2004.10.019
- Dilmi A, Bartil T, Yahia N, et al. Hydrogels based on 2-hydroxyethylmethacrylate and chitosan: preparation, swelling behavior, and drug delivery. Int. J. Polym. Mater. Polym. Biomater. 2014;63:502–509.10.1080/00914037.2013.854221
- Kim SK. Small intestine transit time in the normal small bowel study. Am. J. Roentgenol. 1968;104:522–524.10.2214/ajr.104.3.522
- Ghoshal UC, Sengar V, Srivastava D. Colonic transit study technique and interpretation: can these be uniform globally in different populations with non-uniform colon transit time. J. Neurogastroenterol. Motil. 2012;18:227–228.10.5056/jnm.2012.18.2.227
- Kim ER, Rhee PL. How to interpret a functional or motility test – colon transit study. J. Neurogastroenterol. Motil. 2012;18:94–99.10.5056/jnm.2012.18.1.94
- Tarantili PA, Andreopoulus AG. Design of biodegradable polymer systems for drug release applications. Proceeding of the 8th Polymers for Advanced Technologies International Symposium Budapest, 2005 September 13–16; Hungary.
- Zilberman M. Dexamethasone loaded bioresorbable films used in medical support devices: structure, degradation, crystallinity and drug release. Acta Biomater. 2005;1:615–624.10.1016/j.actbio.2005.06.007