Abstract
The European Union Water Framework Directive represents a new, holistic approach to safeguarding water quality and improving aquatic ecosystems. The challenges faced by managers of multinational river basins are highlighted in the River Danube system, which covers an area exceeding 800,000 km2, incorporating all or part of 14 countries. This study investigates the challenges faced with attempting to achieve and maintain ‘good’ status for water quality due to the widespread occurrence of metal mining activity throughout the Danube drainage basin. The release of contaminant metals (notably Cd, Cu, Pb, Zn) and As from point and diffuse sources results in solute metal loads within certain affected rivers that exceed 3000 kg/day, causing metal concentrations to exceed water quality guidelines by up to 280 times and giving rise to the associated degradation of aquatic environments. Within mining-affected catchments, contaminant metals and As are being dispersed over a variety of spatial scales, with the dispersal of sediment-associated metals occurring over hundreds of kilometres resulting in the mobilization of contaminants across international borders. Establishing efficient contamination management and remediation strategies within the Danube drainage basin will be influenced by predicted increases in winter flood frequency, which will potentially increase the mobilization of contaminants from point and diffuse sources.
1 Introduction
The European Union Water Framework Directive (EU WFD) drawn up by the Council of the European Communities (CEC Citation2000) represents the latest and most ambitious legislative project undertaken within the EU aimed at safeguarding water quality and protecting and improving aquatic ecosystems (Kay et al. Citation2006). The WFD is seen as an innovation in water management in that it seeks to manage river basins based upon natural hydrological and geographical units, rather than on administrative or political boundaries (Blöch Citation1999, White and Howe Citation2003). Each River Basin District (RBD) has its own River Basin Management Plan, which should seek to achieve at least ‘good’ ecological, chemical and physical/morphological status for all water bodies by 2015 (CEC Citation2000). Status definitions are explained in Annex V of legislative document, but the WFD as a whole represents a move towards improving ‘stream health’ and incorporating the concepts of ecosystem health and ecosystem integrity (Karr and Chu Citation1999).
The WFD covers all surface and groundwater and coastal waters up to 1 km offshore (CEC Citation2000), and the use of RBDs creates challenges for the management of large multinational river catchments. Approximately 70 international RBDs can be identified within the European Union including major continental systems such as the Rhine and Danube. The River Danube drainage basin, for example, covers an area of 817,000 km2 and incorporates 14 countries.
Safeguarding and improving water quality will involve addressing problems arising from potential point and diffuse pollution sources (Kay et al. Citation2006). The relative problems of dealing with diffuse pollution sources have been highlighted in relation to both organic (e.g. Vinten et al. Citation2004, Kay et al. Citation2005) and inorganic (e.g. Rozema et al. Citation2008) contaminants. The Danube drainage basin, particularly the lower Danube comprising Hungary, Serbia, Bulgaria and Romania, has a long history of base and precious metal mining and metallurgy that have released contaminant metals into the riverine environment (Panayotova Citation1997, Odor et al. Citation1998, Forray and Hallbauer Citation2000, Macklin et al. Citation2003). The potential environmental implications of mining activities in the Danube drainage basin were highlighted by the Aurul tailings dam failure, which occurred in Maramureş County, north-west Romania in January 2000 (Wehland et al. Citation2002, Macklin et al. Citation2003). The release and subsequent dispersal of 100,000 m3 of metal and CN-rich water and sediment through the Tisa and Danube river systems caused widespread ecological damage (UNEP Citation2000) and highlighted the issues related to the trans-boundary dispersal of contaminants. The Aurul failure was followed in March 2000 by the failure of the Novaţ-Roşu tailings dam near Baia Borşa 100 km to the east (Bird et al. Citation2008). This event released a further 100,000 m3 of contaminated water and 20,000 tonnes of tailings-rich sediment into the upper Tisa catchment (UNEP Citation2000).
While numerous studies have identified contamination problems arising from metalliferous mining in constituent catchments within the Danube system, none have sought to assess the significance of contamination problems at the basin scale and evaluate the implications for river basin management and the implementation and application of the EU WFD.
2 The Danube drainage basin
The River Danube flows eastwards for 2850 km from its headwaters in the German Black Forest to its delta on the Romanian Black Sea coast. In addition to Germany and Romania, the Danube also drains parts of Switzerland, Austria, Slovakia, Slovenia, Hungary, Croatia, Serbia, Bosnia Herzegovina, Bulgaria, Moldova, Poland and the Ukraine. The River Danube drainage basin contains a number of major centres of base and precious metal mining () with ore deposits associated with volcanic rocks of varying ages and lithologies (Neubauer and Heinrich Citation2003). Of particular importance in the lower Danube drainage basin are base and precious metal deposits associated with the Apuseni–Banat–Timok–Srednogorie (ABTS) belt, also known as the Banatite Magmatic–Metallogenic belt (Ciobanu et al. Citation2002, Neubauer and Heinrich Citation2003), which comprises an L-shaped structure of Late-Cretaceous calc-alkaline magmatic activity within the Alpine–Carpathian–Balkan–Dinaride orogen of eastern Central Europe (Von Quadt et al. Citation2005). The ABTS belt is dominated by Cu and Cu–Au ore deposits (von Quadt et al. Citation2003) and links world-class deposits in the southern Apuseni Mountains of Romania with those in the Panagyurishte metallogenic zone of central Bulgaria and the Timok region of western Serbia (Kouzmanov et al. Citation2005). Ciobanu et al. Citation(2002) have identified 50 mineral deposits associated with the ABTS belt.
2.1 Metal mining
Major centres of historic and contemporary metalliferous mining are found in north-west and west-central Romania, eastern Hungary, eastern Serbia and north-west and north-central Bulgaria (). Metal mining in Romania is principally focused in the north-west of the country with the mining of polymetallic ores found as hydrothermal veins hosted by Neogene-age andesites (Lang Citation1979, Cook Citation1995, Cook and Chiaradia Citation1997) and in the Apuseni Mountains at the northern end of the ABTS belt, which are believed to comprise over 50% of Romania's total metal resources (Milu et al. Citation2004). The Apuseni Mountains also contain the recently developed Au-rich deposit at Roşia Montana, which at 450 tonnes is believed to comprise Europe's largest gold deposit (Kouzmanov et al. Citation2005). Also in the Tisa basin are the Matra Mountains of eastern Hungary, which comprise magmatic exceptions to Hungary's sedimentary-dominated geology (Pécsi and Sárfalvi Citation1964). Mining in the Matra Mountains has focused upon the Recsk-Lahóca Cu–Au and the Gyöngyösorszi Pb/Zn deposits (), which are of Eocene and Miocene age, respectively (Gatter et al. Citation1999, Heinrich and Neubauer Citation2002). Metal mining in Serbia is focused on the Bor and Madjanpek Cu deposits (Jankovic Citation1990), which fall within the River Timok catchment, a right bank tributary of the Danube. Copper is also mined from the Elatsite deposit within the Panagyurishte ore district of central Bulgaria (Von Quadt et al. Citation2005).
3 Field sampling and analytical methods
This study presents trace metal and As data determined for samples of river water, river channel sediment, floodplain sediment and mining-related waste from throughout the Danube drainage basin. In the field, samples of river water were filtered through 0.45 µm cellulose nitrate membranes, stabilized with five drops of 50% HNO3 and stored in acid-washed Nalgene bottles prior to analysis by ICP-MS at Aberystwyth University. Analytical precision determining using blind duplicate samples (10% of total sample number) was found to be within 10% for As (5.3%), Cd (3.8%), Cu (4.2%), Pb (8.3%) and Zn (7.5%). Measurements of pH and EC were taken using a YSI 556/MPS multiprobe. Samples of river channel sediment were collected from exposed bar surfaces, with 10 spot samples being collected over a 5 m radius using a stainless-steel trowel. Samples of floodplain sediment were collected from between 10 and 60 cm below ground to quantify metal concentrations in longer-term storage. Prior to analysis using ICP-MS, sediment samples were disaggregated and sieved through a 63 µm mesh to isolate the chemically active silt and clay fraction; a sub-sample was then digested in 70% HNO3 at 100°C for 1 h. Analytical accuracy was monitored through repeat analyses of an in-house reference material (ABS-1) and precision established through the repeat analysis of blind duplicates (10% of total sample number). Precision and accuracy for As and all metals were found to be within 10%, with the exception of Cd (accuracy of 13.3%) due to low concentration (<0.2 mg/kg).
4 Actively mined river catchments
The surface drainage network has been described by Allan Citation(1988) as the principal conduit for the dispersal of mining-derived metals through the environment. This is principally due to the close spatial association between rivers and metal mining, historically driven by the mining industry's need for water as a source of power and in the processing of ore material (Allan Citation1997). Metal contaminants may be released into the fluvial environment from sites of mining activity through four key processes: Equation(1) the generation and release of acid mine drainage (AMD) and acid rock drainage (ARD) (Lind and Hem Citation1993, Wong et al.
Citation1998, Kurkjian et al.
Citation2004), (2) the release of metal-rich waste slurries (Miller et al.
Citation2002), (3) the erosion of spoil tips (Merrington and Alloway Citation1994) and (4) through mine tailings dam failures (Grimalt et al.
Citation1999, Hudson-Edwards et al.
Citation2003).
Throughout the River Danube drainage basin, the mining and processing of metal ores leads to point-source releases of contaminant metals (Cd, Cu, Pb, Zn) and As to the local surface drainage network. Data from mining-affected catchments indicate the importance of point sources in the delivery of solute metals to the riverine environment, often through the unmitigated release of mine drainage, resulting in concentrations that are elevated many times above concentrations found naturally occurring in surface water (). A majority of the drainage samples have low pH, typically 2.5–3.5, indicating ferruginous discharges with sufficient iron pyrite present to generate acidity. However, several samples, notably in Bulgaria, are what can be termed circum-neutral mine drainage (Nuttall and Younger Citation2000) with pHs in the region of 6–9. The higher pH is caused by either an absence of acid-generating pyrite in the ore material or buffering by the presence of a carbonate host rock or gangue constitute (Warrender and Pearce Citation2007). Despite the higher pH, solute Zn concentrations are present at up to 83,000 µg/l, potentially due to the relatively high pH of hydrolysis of Zn compared with other metals (Levinson Citation1974).
Table 1 Mean metal and As concentrations (µg/l) in point-source mine drainage within the Danube drainage basin
Release of metal-rich drainage waters severely contaminates recipient streams in mining-affected catchments throughout the Danube drainage basin (). The data presented in are from rural streams and rivers, where metal mining activity represents the only, or dominant, source of metals derived from anthropogenic activity. The Certej River in the southern Apuseni Mountains, for example, illustrates how untreated AMD and ARD discharges from a site of active metal mining can grossly contaminate and degrade local surface water quality. The River Certej is a small tributary (24 km) of the River Mureş that is affected by the mining of Pb/Zn + Au ores at the Coranda Mine in the southern Apuseni Mountains. Solute concentrations in the river water of the River Certej peak at 1400 µg/l Cd, 3400 µg/l Cu, 170 µg/l Pb and 156,000 µg/l Zn with a pH range of 2.8–7.4 (). These peak concentrations represent an enrichment over ‘imperative’ values defined by EU Directive 75/440/EEC of 280 (Cd), 68 (Cu), 3.4 (Pb) and 52 (Zn) times. The potential toxicological implications of contaminant metals to aquatic flora and fauna and human health have been reviewed by a number of authors (Das et al. Citation1997, Lindh Citation2005, Nordberg and Cherian Citation2005), and it is evident that safeguarding the ecological status of surface waters in a number of mining-affected catchments subjected to point-source inputs will be threatened.
Table 2 Metal and As concentrations (µg/l) in river water as a result of inputs from mining point sources
While concentration data offer a valuable insight into the magnitude of contamination in surface waters impacted by metal mining activity, it is becoming increasingly apparent that calculating metal loads within affected river systems offers an insight into the flux of contaminant-metals from point sources and in within recipient rivers. Adapting the approach of Kimball et al. Citation(2007), the dissolved load (defined here as that passing through a 0.45 µm filter) can be defined as follows:
Metal loads in selected mine drainage and affected rivers in the Danube catchment have been calculated where discharge data for the time of sampling is available (). For river water, discharge data were only available for sample sites lower end of study reaches, therefore metal loads have been calculated for these locations and will indicate ‘cumulative’ effect of upstream metal inputs to the affected rivers. Within mine drainage, Cu and Zn loads of up to 6.9 and 110 kg/day, respectively, are observable, with loads of As (0.03–0.06 kg/day) and Cd (0.11–0.83 kg/day) reflecting their lower abundances in metal ores.
Table 3 Estimated daily metal loads (kg/day) from mining point sources and in mining-affected rivers
Within mining-affected rivers, metal loads in river water are generally higher than those in mine drainage, for example, up to 250 kg/day of Cu and 3600 kg/day Zn are estimated to be delivered through the downstream extent of the River Turţ in Romania. While the River Turţ receives direct inputs of mine drainage from the Penhiger and Ghezuri mines (), the metal loads in the downstream reach of the Turţ are 4.9 to 45 times higher than those measured in mine drainage from these inputs. In terms of percentage values, total fluxes of metals from the Ghezuri and Penhiger mines represent only 2–20% of the metal loads observed in the lower River Turţ. This can also be in other rivers for which data are available, for example, metal and As loads in mine drainage from the Roşia Montana mine are two to nine times lower than those measured in the lower River Roşia, representing between 11% and 60% of the metal loads. Given that metal mining constitutes the only industrial source of contaminants in these catchments, the higher loads in river water indicate that metals are being released into a number of these mining-affected rivers from other sources. This could represent metal inputs from other tributary streams: diffuse AMD or ARD from mining activity and/or changes in the partitioning of metals between ‘solute’ and sediment-associated phases. However, what is apparent from the data in and is that metal mining activity in river catchments in the Danube drainage basin results in severely contaminated surface water and very high metal loads within affected riverine environments.
While the previous discussion has highlighted how poorly managed mining practices result in the release of metal-rich drainage waters, it is apparent that the dispersal of solute contaminant metals within the Danube drainage basin is spatially limited to within approximately 15–30 km of major point sources. For example, metal levels measured along an 800 km reach of the Lapuş–Someş–Tisa river system in Romania and Hungary fall below target values within approximately 20 km of major point-source releases from the Băiut¸ mine (). This is likely to result from a combination of physical dilution with inputs of relatively cleaner water from tributaries and through interaction with particulate matter through the processes of sorption, precipitation and complexation (Salomons Citation1988, Bordas and Bourg Citation2001), especially in the geochemically active zone around mine waste inputs (Karlsson et al. Citation1988). As a result, it is apparent that the transboundary dispersal of solute metals is not occurring within the Danube system, as evidenced by concentrations within the River Danube itself and in tributary streams immediately upstream of national boundaries ().
Figure 2 Metal concentrations in river water measured in the Rivers Lapuş, Someş and Tisa and selected tributaries plotted with 75/440/EEC target and imperative values for metals in surface water
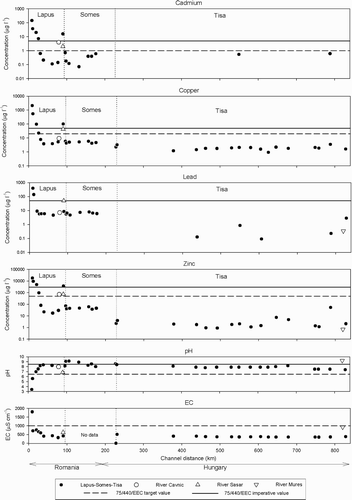
Table 4 Metal and As concentrations (µg/l) in river water measured at international borders
However, it is important to note that mining-related point sources will not constitute the only source of solute contaminant metals to the riverine environment. For example, the River Sasar in north-west Romania flows through the city of Baia Mare, a historical centre of mining activity, and its lower reaches were affected by the year 2000 Aurul tailings dam failure. Metal concentrations measured annually in river water between July 2000 and July 2003 () consistently exceeded EU target values within and downstream of the Baia Mare urban area. However, Cu and Pb concentrations measured 8 years prior to the Aurul failure (UNEP Citation2000) are generally higher than those measured after the mining accident, highlighting the historical, and ongoing, importance of other municipal and industrial sources in contributing to the contaminant-metal levels. Similarly, work by Stoica et al. Citation(2000), found elevated concentrations of Al (1.8–4300 µg/l) and Pb (1–163 µg/l) in the water of the Arges River, a Romanian tributary of the River Danube that receives metal inputs not associated with metal mining activity, but with industrial and municipal sources in the Bucharest region.
Figure 3 Metal concentrations in river water measured in the River Sasar, north-west Romania, plotted with 75/440/EEC target and imperative values
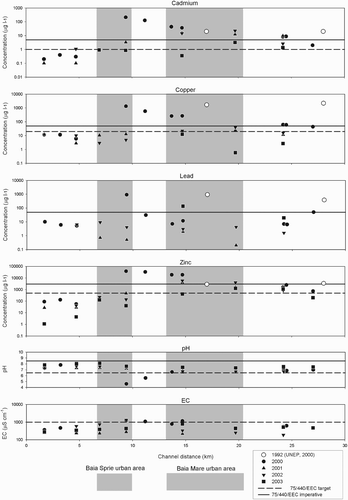
While there are numerous European- and nationally defined guideline values for contaminant substances in water (), a key legislative aspect of the EU WFD in evaluating water quality and ensuring at least ‘good’ status for water bodies is that concentrations of man-made contaminants should be zero, while naturally occurring substances, such as metal elements, should be at ‘background’ levels (CEC Citation2000). This approach therefore requires the characterization of water bodies, as carried out as part of the WFD implementation strategy, and the establishment of ‘background’ concentrations. Difficulties in establishing representative background concentrations in many European river basins, due to long histories of catchment modification, have been highlighted by Ward et al. Citation(2001).
Table 5 Water quality standards for selected metals and As (values in µg/l)
provides a summary of ‘background’ metal and As concentrations for river catchments within the Danube drainage basin. These concentrations have been determined following the method of Scancar et al. Citation(2002), with samples collected from catchment headwaters, upstream of locations of mining and/or industrial activity, and will therefore reflect metal and As concentrations generally resulting from natural bedrock weathering. Notable differences in concentrations can be seen between catchments, in part reflecting differing geologies and land uses, and highlights the catchment specific nature of these values. It is also apparent that river waters affected by metal inputs from the metal mining industry () are elevated by several orders of magnitude above these background concentrations.
Table 6 Mean background As and metal concentrations (µg/l) and pH levels in river water from the Danube drainage basin
5 Transboundary dispersal of sediment-bound metals
The importance of sediment-associated metal transport within the Danube drainage basin is apparent from metal concentrations measured in river channel sediment (). Mean and maximum concentrations exceed quality guidelines for freshwater sediments defined by the US EPA Citation(2002). For example, mean Cd, Cu and Zn concentrations in the upper Tisa and Mureş river catchments are between 2.7 and 5.4 times enriched above US EPA probable effect concentration (PEC) thresholds, with maximum concentrations being enriched between 22 and 174 times PEC thresholds. While mean and maximum concentrations in the River Danube itself are generally lower, maximum Cd, Cu, Pb, Zn and mean Cu levels exceed US EPA threshold effect concentration (TEC) values and will reflect the cumulative effects of metal inputs from tributary catchments. While mobilization of these channel sediments may be episodic, governed by increases in discharge (Dennis et al. Citation2003), the widespread presence of elevated metal levels within these sediments, particularly in the lower reaches of river catchments, where corresponding solute concentrations are very low (e.g. ), highlights their importance in contributing to the catchment-wide dispersal of contaminant metals.
Figure 4 Minimum, mean and maximum metal concentrations measured river channel sediment in the River Danube and major tributaries. Data for Rivers Velika Morava and Siret adapted from Woitke et al. Citation(2003); data for the River Sava adapted from Ščančar et al. Citation(2007)
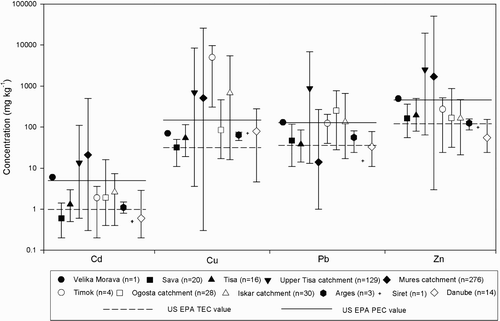
Further evidence for the transboundary dispersal of contaminant metals within the Danube drainage basin can be seen in the Tisa catchment where metals sourced from mining activity in Romania are dispersed downstream into Hungary via the Rivers Someş and Mureş (). While contamination of river water has been found to be generally restricted to within 30 km of point sources, sediment-associated metals are dispersed much greater distances. For example, Cd (1.3 mg/kg), Cu (71 mg/kg), Pb (36 mg/kg) and Zn (225 mg/kg) levels in the River Tisa exceed US EPA TEC values approximately 750 km downstream of the nearest location of mining activity ().
Figure 5 Metal concentrations in river channel sediment measured in the Rivers Lapuş, Someş and Tisa and selected tributaries plotted with US EPA TEC and PEC values for metals in freshwater sediments
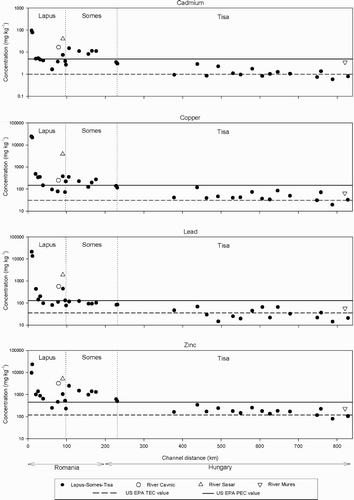
The spatially extensive downstream dispersal of sediment-associated contaminated metals has resulted in metal levels exceeding environmental quality guidelines in substantial proportions of river channel lengths (). For example Cd, Cu, Pb and Zn levels in mining-affected tributaries of the River Danube exceed at least US EPA TEC levels in between 60% and 91% of the rivers, while in the Bulgarian Danube enrichment of Cu is most spatially extensive; exceeding at least TEC values in 92% of the channel length. The data in indicate the widespread contamination of within-channel river sediments and highlight the significant challenge in managing a large international drainage basin, where contaminants are being dispersed across international boundaries.
Table 7 Percentage channel length where concentrations in river sediment fall below and above US EPA TEC and PEC concentrations
It is important to note that the environmental and ecological significance of these sediment-associated metals will be governed, at least in part, by their geochemical speciation, which is of critical importance in controlling potential mobility and toxicity (Buykx et al. Citation2000, Hursthouse Citation2001). Speciation has been widely investigated using sequential extraction procedures (SEPs) (Bacon and Davidson Citation2008), with metals associated with ‘weakly bound’ or ‘exchangeable’ sedimentary species being of primary environmental concern (Ma and Rao Citation1997). Work by Bird et al. (Citation2003, Citation2005) in the Tisa and Mureş catchments using the Bureau of Community Reference SEP has established that 19–75% of Cd and Zn in river channel sediments were present in the ‘exchangeable’ sedimentary phase and, as a result, was of environmental concern at a number of locations within these catchments due to their relative mobility and bioavailability.
6 Floodplain storage of sediment-associated metals
The dispersal of contaminant metals in association with river sediments allows for their incorporation into floodplain sedimentary stores, particularly during periods of overbank flow. provides a summary of contaminant-metal concentrations measured in floodplain sediment within the Danube drainage basin and highlights the presence of elevated concentrations in a number of river systems, further emphasizing the importance of floodplain sediments as long-term sinks for heavy metals in mining-affected catchments (Lewin and Macklin Citation1987, Marron Citation1992, Swennen and Van der Sluys Citation2002). Incorporation of contaminant metals into overbank floodplain sediments can provide a legacy of contamination long after the cessation of active mining. For example, As, Pb and Zn concentrations in the River Ogosta, a right-bank tributary of the River Danube in north-west Bulgaria, are enriched above US EPA thresholds, despite the fact that Pb/Zn-Au mining activity has ceased (). In addition to Cd, Cu, Pb and Zn, enriched As concentrations (10–10,800 mg/kg) are associated with the high arsenopyrite content of the Pb/Zn–Au ores (Kotsev Citation2001) and can also be found in deposits of tailings material present within the catchment (Vesselinov et al. Citation1996).
Figure 6 Minimum, mean and maximum metal concentrations measured in floodplain sediment in the River Danube and mining-affected tributaries. Data for the River Sava adapted from Maljević et al. Citation(2004)
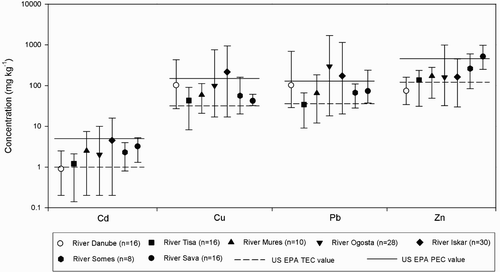
Work by Dennis et al. Citation(2003) has shown that alluvial material is particularly susceptible to remobilization during major floods, causing redispersal of contaminants over potentially large distances. With evidence of a recent increase in the frequency of major floods in the Danube drainage basin (Bardossy et al. Citation2003), and a predicted future increase in the frequency and magnitude of high flows in the Danube system (Feyen et al. Citation2006), the presence of contaminated alluvium within tributaries of the River Danube () may be of notable concern for the management of the wider Danube drainage basin. Further work is required to fully establish metal loads within contaminated floodplain environments and to quantify volumes of contaminated alluvium present within affected catchments (Dennis et al. Citation2009).
7 Conclusions and implications for catchment management
Implementation of the EU WFD represents a considerable challenge to responsible authorities in catchments that contain metal mining activity, both in respect to developing ecologically based assessment methods for water quality (Szilágyi et al. Citation2008) and characterizing RBDs (Munne and Prat Citation2004) and also in relation to ultimately safeguarding and improving water quality. The Danube drainage basin provides a clear example of the challenges to catchment and water resource management posed by point and diffuse-source release of contaminant metals associated with mining and metallurgy, as well as other industrial and municipal sources. The task of improving and maintaining the quality of aquatic environments is complicated in the multinational nature of the catchment and that contaminant metals, particularly associated with fluvial sediments, are being dispersed across national boundaries, sometimes creating a national differentiation between sources and sinks of contaminants.
Advancement in the development of treatment and remediation strategies for point-source contamination associated with ARD and AMD (Zoumis et al. Citation2001, Dinelli et al. Citation2007), treating mine waters (Perkins et al. Citation2006, Bearcock and Perkins Citation2007, Ríos et al. Citation2008) and stabilizing deposits of mine waste (Grandlic et al. Citation2008) offers a potential means of reducing the relatively localized, deleterious impacts upon river water quality. However, data collected from across the lower Danube drainage basin have highlighted the problems caused by the dispersal and storage of sediment-associated contaminant metals.
The data presented here have clearly highlighted that the challenges posed to responsible authorities in implementing the EU WFD by the impact and legacy of metalliferous mining are widespread. However, it is important to note that the EU WFD legislation offers river basin managers and responsible authorities the option of delaying compliance of an RBD by 2015 for reasons of technical feasibility, disproportionate cost or natural conditions (CEC Citation2000). The legislation also offers member states the possibility of engaging in a further two 6-year cycles of planning and implementation of measures through which they can achieve the outcome of achieving at least ‘good’ ecological status.
Acknowledgements
The authors would like to thank The Royal Society and Aberystwyth University for their support in funding their research in the Danube drainage basin over the past 10 years.
References
- Allan , R. J. 1988 . “ Mining activities as sources of metals and metalloids to the hydrosphere ” . In Metals and metalloids in the hydrosphere: impact through mining and industry, and prevention technology , Edited by: Strigel , G. 45 – 67 . Paris : UNESCO .
- Allan , R. 1997 . Introduction: mining and metals in the environment . Journal of Geochemical Exploration , 58 : 95 – 100 .
- Bacon , J. R. and Davidson , C. M. 2008 . Is there a future for sequential chemical extraction? . Analyst , 133 : 25 – 46 .
- Bardossy , A. , Stehlik , J. and Balint , G. 2003 . “ Investigation of the flood producing weather situations of the 20th century for the upper part of the Tisza bas ” . In Historical floods and climate variability: applications in flood risk assessment, proceedings of the PHEFRA international workshop in Barcelona Edited by: Thorndycraft , V. R. , Benito , G. , Barriendos , M. and Llasat , M. C. 301 – 306 .
- Bearcock , J. M. and Perkins , W. 2007 . “ The use of green rust to accelerate precipitation of ochre for mine water remediation ” . In IMWA symposium 2007: water in mining environments , Edited by: Cidu , R. and Frau , F. 135 – 139 . Cagliari, , Italy : Mako Edizioni .
- Behrami , S. , Bajraktari , F. and Zogaj , N. Ground water pollution in Mitrovica and surroundings . BALWOIS 2008: conference on water observation and information system for decision support . Ohrid, Republic of Macedonia. Edited by: Morell , M. , Popovska , C. , Morell , O. , Stojov , V. , Kostoski , G. , Dimitrov , D. , Drobot , R. , Radic , Z. and Selenica , A. pp. 1 – 5 . Ministry of Education and Science .
- Bird , G. 2003 . The solid-state partitioning of contaminant metals and As in river channel sediments of the mining affected Tisa drainage basin, northwestern Romania and eastern Hungary . Applied Geochemistry , 18 : 1583 – 1595 .
- Bird , G. 2005 . The magnitude, spatial extent and environmental significance of heavy metal contamination in the Aries river catchment, western Romania: implications for development of the Rosia Montana gold deposit . Journal of Geochemical Exploration , 86 : 26 – 48 .
- Bird , G. 2008 . River system recovery following the Novaţ-Roşu tailings dam failure, Maramureş County, Romania . Applied Geochemistry , 23 : 3498 – 3518 .
- Blöch , H. 1999 . The European Union Water Framework Directive: taking European water policy into the next millenium . Water Science and Technology , 40 : 67 – 71 .
- Bordas , F. and Bourg , A. C.M. 2001 . Effect of solid/liquid ratio on the remobilization of Cu, Pb, Cd and Zn from polluted river sediment . Water, Air and Soil Pollution , 128 : 391 – 400 .
- Buykx , S. E.J. 2000 . The effects of oxidation and acidification on the speciation of heavy metals in sulfide-rich freshwater sediments using a sequential extraction procedure . Journal of Environmental Monitoring , 2 : 23 – 27 .
- CEC . 2000 . Directive 2000/60/EC of the European Parliament and the Council of 23 October 2000 establishing a framework for Community action in the field of water policy . Official Journal of the European Communities , L327 : 1 – 72 .
- Ciobanu , C. L. , Cook , N. J. and Stein , H. 2002 . Regional setting and geochronology of the Late Cretaceous Banatitic Magmatic and Metallogenic Belt . Mineralium Deposita , 37 : 541 – 567 .
- Cook , N. J. Polymetallic massive sulphide deposits at Baia Borsa, Romania . Proceedings of the third biennial SGA meeting . Prague, Czech Republic. Edited by: Pašava , J. , Kríbek , B. and Žák , K. pp. 851 – 854 . Rotterdam : A.A. Balkema .
- Cook , N. J. and Chiaradia , M. Sources of base metal mineralisation in the Baia Borsa orefield, N. W. Romania: constraints from lead isotopes . Proceedings of the fourth biennial SGA meeting . Turku, Finland. Edited by: Papunen , H. pp. 813 – 816 . Rotterdam : A.A. Balkema .
- Das , P. , Samantaray , S. and Rout , G. R. 1997 . Studies on cadmium toxicity: a review . Environmental Pollution , 98 : 29 – 36 .
- Dennis , I. 2003 . The impact of the October–November 2000 floods on contaminant metal dispersal in the River Swales catchment, North Yorkshire, UK . Hydrological Processes , 17 : 1641 – 1657 .
- Dennis , I. A. 2009 . The role of floodplains in attenuating contaminated sediment fluxes in formerly mined drainage basins . Earth Surface Processes and Landforms , 34 : 453 – 466 .
- Dinelli , E. Treatment of acidic mine drainage at Labiola Mine, Liguria, Italy . IMWA symposium 2007: water in mining environments . Cagliari, Italy. Edited by: Cidu , R. and Frau , F. pp. 171 – 175 . Mako Edizioni .
- Feyen , L. 2006 . Flood risk in Europe in a changing climate , 20 Ispra, Italy : European Commission Joint Research Centre, Institute of Environment and Sustainability .
- Forray , F. L. and Hallbauer , D. K. 2000 . A study of the pollution of the Aries River (Romania) using capillary electrophoresis as analytical technique . Environmental Geology , 39 : 1372 – 1384 .
- Gatter , I. 1999 . “ High- and low-sulfidation epithermal mineralization of the Màtra Mountains, Northeast Hungary ” . In Epithermal mineralization of the western Carpathians. Society of Economic Geologists Guidebook Series , Edited by: Molnàr , F. , Lexa , J. and Hedenquist , J. W. Vol. 31 , 155 – 179 . Littleton, CO : Society of Economic Geologists .
- Grandlic , C. J. 2008 . Plant growth-promoting bacteria for phytostabilization of mine tailings . Environmental Science and Technology , 42 : 2079 – 2084 .
- Grimalt , J. O. , Ferrer , M. and Macpherson , E. 1999 . The mine tailing accident in Aznalcollar . Science of the Total Environment , 242 : 3 – 11 .
- Heinrich , C. A. and Neubauer , F. 2002 . Cu–Au–Pb–Zn–Ag metallogeny of the Alpine–Balkan–Carpathian–Dinaride geodynamic province . Mineralium Deposita , 37 : 533 – 540 .
- Hudson-Edwards , K. A. 2003 . The impact of tailings dam spills and clean-up operations on sediment and water quality in river systems: the Rios Agrio-Guadiamar, Aznacollar, Spain . Applied Geochemistry , 18 : 221 – 239 .
- Hursthouse , A. S. 2001 . The relevance of speciation in the remediation of soils and sediments contaminated by metallic elements – an overview with specific examples from central Scotland . Journal of Environmental Monitoring , 3 : 49 – 60 .
- Jankovic , S. 1990 . Types of copper deposits related to volcanic environment in the Bor district, Yugoslavia . Geologische Rundschau , 79 : 467 – 478 .
- Jordan , G. Environmental impact of metal mining on catchment drainage in the historic mining area of Recsk-Lahóca Mines, Hungary . Proceedings of the 4th European congress on regional geoscientific cartography and information systems . Bologna. pp. 713 – 715 .
- Karlsson , S. , Allard , B. and Hakansson , K. 1988 . Characterization of suspended solids in a stream recieving acid mine effluents, Bersbo, Sweden . Applied Geochemistry , 3 : 345 – 356 .
- Karr , J. R. and Chu , E. W. 1999 . Restoring life in running waters: better biological monitoring , 206 Washington, DC : Island Press .
- Kay , D. 2005 . Predicting faecal indicator fluxes using digital land use data in the UK's sentinel Water Framework Directive catchment: The Ribble study . Water Research , 39 : 3967 – 3981 .
- Kay , D. 2006 . Europe: a challenging new framework for water quality . Civil Engineering , 159 : 58 – 64 .
- Kimball , B. A. , Walton-Day , K. and Runkel , R. L. 2007 . “ Quantification of metal loading bu tracer injection and synoptic sampling, 1996–2000 ” . In Integrated investigations of environmental effects of historical mining in the Animas River watershed Edited by: Church , S. E. , von Guerard , P. and Finger , S. E. 423 – 495 . San Juan County, , Colorado USGS Professional Paper 1651, Boulder, Colorado
- Korac , M. and Kamberovic , Z. Characterization of wastewater streams from Bor site . Proceedings of the 4th Balkan conference on metallurgy . pp. 411 – 421 . Zlatibor : Association of Metallurgical Engineers of Serbia .
- Kotsev , T. Contemporary heavy metal and arsenic river pollution in the ‘Ogosta’ reservoir drainage basin after the end of the mining activities (in Bulgarian) . Natural potential and sustainable development of the mountain regions, proceedings of the Balkan scientific-applied conference . pp. 415 – 426 . Vratsa, , Bulgaria
- Kouzmanov , K. , Ivaˇşcanu , P. and O'Connor , G. 2005 . Porphyry Cu–Au and epithermal Au–Ag deposits in the southern Apuseni Mountains, Romania . Ore Geology Reviews , 27 : 46 – 47 .
- Kurkjian , R. , Dunlap , C. and Flegal , A. R. 2004 . Long-range downstream effects of urban runoff and acid mine drainage in the Debed River, Armenia: insights from lead isotope modeling . Applied Geochemistry , 19 : 1567 – 1580 .
- Lang , B. 1979 . The base-metals-gold hydrothermal ore deposits of Baia Mare, Romania . Economic Geology , 74 : 1336 – 1351 .
- Levinson , A. A. 1974 . Introduction to exploration geochemistry , 614 Wilmette : Applied Publishing .
- Lewin , J. and Macklin , M. G. Metal mining and floodplain sedimentation in Britain . International geomorphology 1986: proceedings of the first international conference on geomorphology . Edited by: Gardiner , V. pp. 1009 – 1027 . Chichester : John Wiley and Sons .
- Lind , C. J. and Hem , J. D. 1993 . Manganese minerals and associated fine particulates in the streambed of Pinal Creek, Arizona, USA – a mining-related acid drainage problem . Applied Geochemistry , 8 : 67 – 80 .
- Lindh , U. 2005 . “ Biological functions of the elements ” . In Essentials of medical geology: impact of the natural environment on public health , Edited by: Selenius , O. , Alloway , B. J. , Centeno , J. A. , Finkelman , R. B. , Fuge , R. , Lindh , U. and Smedley , P. 115 – 160 . Amsterdam : Elsevier .
- Ma , L. and Rao , G. N. 1997 . Chemical fractionation of Cd, Cu, Ni and Zn in contaminated soils . Journal of Environmental Quality , 26 : 259 – 264 .
- Macklin , M. G. 2003 . The long term fate and environmental significance of contaminant metals released by the January and March 2000 mining tailings dam failures in Maramures County, upper Tisa Basin, Romania . Applied Geochemistry , 18 : 241 – 257 .
- Maljević , E. , Ileš , M. and Miletić , A. Screening and quantification of some heavy metals in sediments from the Sava River . BALWOIS 2004: conference on water observation and information system for decision support . Ohrid. Edited by: Morell , M. , Todorovik , O. , Dimitrov , D. , Selenica , A. and Spirkovsky , Z. pp. 1 – 10 . Ministry of Education and Science . Republic of Macedonia
- Marron , D. C. 1992 . Floodplain storage of mine tailings in the Belle Fourche River system: a sediment budget approach . Earth Surface Processes and Landforms , 17 : 675 – 685 .
- Merrington , G. and Alloway , B. J. 1994 . The transfer and fate of Cd, Cu, Pb and Zn from two historic mine sites in the UK . Applied Geochemistry , 9 : 677 – 687 .
- Miller , J. R. 2002 . Lead isotopic fingerprinting of heavy metal contamination, Rio Pilcomayo basin, Bolivia . Geochemistry: Exploration, Environment, Analysis , 2 : 225 – 233 .
- Milu , V. , Milesi , J. P. and Leroy , J. L. 2004 . Rosia Poieni copper deposit, Apuseni Milu, V., Leroy, J.L., and Peiffert, C., 2002. Water contamination downstream from a copper mine in the Apuseni Mountains, Romania . Environmental Geology , 42 : 773 – 782 .
- Mountains . Romania: advanced argillic overprint of a porphyry system . Mineralium Deposita , 39 173 – 188 .
- Munne , A. and Prat , N. 2004 . Defining river types in a Mediterranean area: a methodology for the implementation of the EU water framework directive . Environmental Management , 34 : 711 – 729 .
- Neubauer , F. and Heinrich , C. A. 2003 . “ Late Cretaceous and Tertiary geodynamics and ore deposit evolution of the Alpine–Balkan–Carpathian–Dinaride orogen ” . In Mineral exploration and sustainable development , Edited by: Eliopolous , D. G. 1133 – 1136 . Rotterdam : Millpress .
- Nordberg , M. M. and Cherian , M. G. 2005 . “ Biological responses of elements ” . In Essentials of medical geology: impact of the natural environment on public health , Edited by: Selenius , O. , Alloway , B. J. , Centeno , J. A. , Finkelman , R. B. , Fuge , R. , Lindh , U. and Smedley , P. 179 – 200 . Amsterdam : Elsevier .
- Nuttall , C. A. and Younger , P. L. 2000 . Zinc removal from hard, circum-neutral mine waters using a novel closed-bed limestone reactor . Water Research , 34 : 1262 – 1268 .
- Odor , L. 1998 . Mobilization and attenuation of metals downstream from a base-metal mining site in the Matra Mountains, northeastern Hungary . Journal of Geochemical Exploration , 65 : 47 – 60 .
- Panayotova , M. 1997 . Impact of sulphide non-ferrous ore mining and dressing activities on the environment . Journal of Environmental Science and Health Part A – Environmental Science and Engineering and Toxic and Hazardous Substance Control , 32 : 2213 – 2228 .
- Pécsi , M. and Sárfalvi , B. 1964 . The geography of Hungary , 299 London : Collet's .
- Perkins , W. 2006 . Bioadsorption in remediation of metal mine drainage: the use of dealginated seaweed in the BIOMAN project . Geochimica Et Cosmochimica Acta , 70 : A482 – A482 .
- Prestor , J. , Štrucl , S. and Pungartnik , M. 2003 . Mežica lead and zinc mine closure and impact on hydrogeological conditions in upper Meža valley . Materials and Geoenvironment , 50 : 313 – 316 .
- von Quadt , A. 2005 . Geochronology and geodynamics of Late Cretaceous magmatism and Cu–Au mineralization in the Panagyurishte region of the Apuseni–Banat–Timok–Srednogorie belt, Bulgaria . Ore Geology Reviews , 27 : 95 – 126 .
- von Quadt , A. , Peytcheva , I. and Cvetkovic , V. 2003 . “ Geochronology, geochemistry and isotope tracing of the Cretaceous magmatism of east-Serbia and Panagyurishte district (Bulgaria) as part of the Apuseni–Timok–Srednogorie metallogenic belt in eastern Europe ” . In Mineral exploration and sustainable development , Edited by: Eliopolous , D. G. 407 – 410 . Rotterdam : Millpress .
- Ríos , C. A. , Williams , C. D. and Roberts , C. L. 2008 . Removal of heavy metals from acid mine drainage (AMD) using coal fly ash, natural clinker and synthetic zeolites . Journal of Hazardous Materials , 156 : 23 – 35 .
- Rozema , J. 2008 . Do high levels of diffuse and chronic metal pollution in sediments of Rhine and Meuse floodplains affect structure and functioning of terrestrial ecosystems? . Science of the Total Environment , 406 : 443 – 448 .
- Salomons , W. 1988 . “ Impact of metals from mining and industry on the hydrosphere ” . In Metals and metalloids in the hydrosphere: impact through mining and industry, and prevention technology , Edited by: Strigel , G. 1 – 41 . Paris : UNESCO .
- Šcancar , J. , Milacic , R. and Horvat , M. 2002 . Comparison of various digestion and extraction procedures in analysis of heavy metals in sediments . Water, Air and Soil Pollution , 118 : 87 – 99 .
- Ščančar , J. 2007 . Report on the contamination of the Sava River sediments with metals and organic pollutants , 17 SARIB, Ljunbljana .
- Stoica , A. , Capota , P. and Baiulescu , G. E. 2000 . Determination of copper, cadmium, zinc and lead in Arges River in Romania during four seasons by inductively coupled plasma atomic emission spectrometry and anodic stripping voltammetry . Analytical Letters , 33 : 3025 – 3035 .
- Swennen , R. and Van der Sluys , J. 2002 . Anthropogenic impact on sediment composition and geochemistry in vertical overbank profiles of river alluvium from Belgium and Luxembourg . Journal of Geochemical Exploration , 75 : 93 – 105 .
- Szilágyi , F. 2008 . Application of water framework directive in Hungary: development of biological classification systems . Water Science and Technology , 58 : 2117 – 2125 .
- UNEP . 2000 . Cyanide spill at Baia Mare Romania , 57 Geneva : United Nations Environmental Programme .
- UNEP . 2000 . Mining waste spill from the Baia Borsa processing complex in Romania , 32 Geneva : United Nations Environment Programme .
- US EPA . 2002 . A guidance manual to support the assessment of contaminated sediments in freshwater ecosystems , US EPA-905-B02-001-C, 232 pp
- Vesselinov , I. 1996 . Mineralogical and geochemical characteristics of two tailings ponds of the Martinovo and Chiprovtsi ore-dressing plants (in Bulgarian) . Geochemistry, Mineralogy and Petrology , 31 : 89 – 102 .
- Vinten , A. J.A. 2004 . Relative risk of surface water pollution by E. coli derived from faeces of grazing animals compared to slurry application . Soil Use Management , 20 : 13 – 22 .
- Von Quadt , A. 2005 . The Elatsite porphyry Cu–Au deposit, Bulgaria . Ore Geology Reviews , 27 : 128 – 129 .
- Ward , J. V. 2001 . Understanding natural patterns and processes in river corridors as the basis for effective river restoration . Regulated Rivers – Research and Management , 17 : 311 – 323 .
- Warrender , R. and Pearce , N. J.G. Remediation of circum-neutral, low-iron waters by permeable reactive media . Water in mining environments: IMWA Symposium 2007 . Cagliari, Italy. Edited by: Cidu , R. and Frau , F. pp. 289 – 293 . Mako Edizioni .
- Wehland , F. 2002 . The dam breakage of Baia Mare – a pilot study of magnetic screening . Physics and Chemistry of the Earth , 27 : 1371 – 1376 .
- White , I. and Howe , J. 2003 . Planning and the European Union Water Framework Directive . Journal of Environmental Planning and Management , 46 : 621 – 632 .
- Woitke , P. 2003 . Analysis and assessment of heavy metal pollution in suspended solids and sediments in the River Danube . Chemosphere , 51 : 633 – 642 .
- Wong , J. W.C. , Ip , C. M. and Wong , M. 1998 . Acid-forming capacity of lead–zinc mine tailings and its implications for mine rehabilitation . Environmental Geochemistry and Health , 20 : 149 – 155 .
- Zoumis , T. 2001 . Contaminants in sediments: remobilisation and demobilisation . Science of the Total Environment , 266 : 195 – 202 .