Abstract
The optimal provision of green roof services is largely dependent on the health of the vegetation on the green roof, which is often affected by water availability.We assessed the impact of two substrate components, brick particle size and a polyacrylamide gel additive, and a living mulch (companion planting of Sedum spp.) on the growth, physiological and visual health of newly established plugs of Festuca ovina and Linaria vulgaris during an extreme drought (25 days) in a controlled environment greenhouse. Polyacrylamide gel and large brick substrates increased both plant species' drought tolerance whilst a Sedum living mulch had no effect. Polyacrylamide gel increased the reservoir of water that plants could access during the drought, whilst large brick promoted slower and therefore more drought resistant plant growth. Overall this study shows that polyacrylamide gel and coarser particle size substrates can significantly improve the drought tolerance of green roof plants.
Introduction
Green roofs are becoming much more common in urban areas due to the many services that they can provide (Getter & Rowe, Citation2006; Oberndorfer et al., Citation2007). These services range from storm water attenuation, urban heat island reduction, building cooling and provision of urban green space (Getter & Rowe, Citation2006; Oberndorfer et al., Citation2007). The vegetation component of a green roof is usually key to the successful provision of these services, for example transpiration of plants increases the cooling effect of a roof (Castleton et al., Citation2010) whilst at the same time recharging the available storm water reservoir (Wolf & Lundholm, Citation2008). However a relatively narrow spectrum of plant species are currently used on extensive green roofs (substrate depth < 120 mm) due to their relatively harsh growing environment which includes low moisture levels and nutrition, shallow rooting depth, high wind stress and fluctuating substrate temperatures (Dunnett & Kingsbury, Citation2010). Plants most often used include succulents (e.g. Sedum spp.), mosses, and some herbaceous perennials and annuals (Rowe et al., Citation2012; Snodgrass & Snodgrass, Citation2006). In particular the use of succulent Sedum spp. on green roofs (especially on extensive roofs where substrate depth is less than 100 mm) is very common due to their low growth, shallow rooting, ability to withstand extremely harsh growing conditions and ease of mass propagation (Getter & Rowe, Citation2006; Snodgrass & Snodgrass, Citation2006). However due to their water conservation strategies Sedum spp. can severely reduce the amount of water leaving the substrate via evapotranspiration compared to non succulent species which can lead to a reduced cooling service (Blanusa et al., Citation2013; Lundholm et al., Citation2010).
An alternative to using the small range of plants that are extremely well adapted to the green roof environment is to modify the substrate so it can support a wider range of plants. ‘Increasing the depth of substrate usually leads to more successful plant establishment, growth and long term survival (Durhman et al., Citation2007; Getter & Rowe, Citation2008, Citation2009; Rowe et al. Citation2012; Thuring et al. Citation2010). However increasing depth does not always improve stormwater retention or availability of water for plants (Fassman-Beck et al., Citation2013; Stovin, Citation2010), can lead to greater weed establishment (Nagase et al., Citation2013) and is not always possible due to economic and structural constraints (Dunnett and Kingsbury, Citation2010).’
Therefore a better alternative may be to alter the physical characteristics of the substrate by modifying or adding components (Young et al., Citation2014). By modifying for example; inter particle pore space (Graceson et al., Citation2013), organic content (Nagase & Dunnett, Citation2011; Rowe et al., Citation2006), or the addition of water retaining additives (Farrell et al., Citation2013; Olszewski et al., Citation2010; Savi & Marin, Citation2014) the WHC and plant available water can be increased. This increase in WHC in combination with increased organic matter (Nagase & Dunnett, Citation2011) and depth (Dunnett et al., Citation2008; Thuring et al., Citation2010) can lead to greater plant growth. However promoting high levels of luxuriant plant growth may not be desirable on a green roof as plants may then become more vulnerable to drought conditions (Nagase & Dunnett, Citation2011; Rowe et al., Citation2006). Alternatively, it is possible to increase green roof substrate WHC without causing a large increase in plant growth through the use of water retention gels which are able to absorb large amounts of water (Farrell et al., Citation2013; Olszewski et al., Citation2010; Savi & Marin, Citation2014; Young et al., Citation2014).
Similarly the use of smaller sized particles in green roof substrate has been shown to increase WHC and also plant growth and physiological health (Graceson et al., Citation2013; Young et al., Citation2014), however it is still not clear whether this will increase plant tolerance to drought conditions (Nagase & Dunnett, Citation2011; Rowe et al., Citation2006). Care must also be taken as the use of a large number of small particles can increase saturated substrate weight and also decrease substrate permeability and air filled porosity, both of which are needed for healthy plant growth (FLL, Citation2008).
An alternative to increasing the WHC of a substrate is to reduce the rate at which water is lost from it. Commonly used Sedum spp. mats have been shown to reduce green roof substrate temperatures (Butler & Orians, Citation2011), maintain higher levels of water in substrate by impeding evaporation (Wolf & Lundholm, Citation2008) and improve companion plant appearance during a severe drought potentially due to a facilitative effect (Butler & Orians, Citation2011). However a trade-off will exist using this method as reducing water loss from the substrate will reduce a substrates storm water retention capacity and therefore ability to mitigate storm water. This highlights the need for green roofs to be designed with specific services in mind in order to optimise their provision (Dvorak & Volder, Citation2010; Young et al., Citation2014).
A pot experiment was established to indentify the most effective strategy to increases drought resistance of green roof plants during their establishment period. The growth and physiological health of two common green roof perennial species with contrasting drought tolerances (Festuca ovina, Sheep's Fescue and Linaria vulgaris, Common Toadflax) was assessed during a drought of 25 days which represents an extreme drought. Both plant species were grown in green roof substrates composed of factorial combinations of (i) small or large crushed brick, (ii) presence/absence of a polyacrylamide gel (SwellGel™) and (iii) presence/absence of a Sedum spp. mat on the substrate surface.
It was hypothesised that plant physiological health (photosynthetic capacity) and drought tolerance could be enhanced using three different strategies;
Increase substrate water holding capacity using water absorbent polyacrylamide gel.
Increase substrate water holding capacity using smaller sized brick particles.
Reduce the rate of water loss from the substrate via evapotranspiration using a Sedum mat or ‘living mulch’.
Methods
The study used substrates with two component variables: (i) brick size (small brick of 2–5 mm particle diameter; large brick of 4–15 mm diameter) and (ii) presence or absence of a water retention polyacrylamide gel ‘SwellGelTM’ (www.swellgel.co.uk) (Table ). Brick was crushed waste red brick, sieved to ensure brick fragments were within the size limits set. SwellGelTM is a soil additive made of cross linked polyacrylamide which is designed to expand and store water during high moisture levels and release it slowly back to the plant as moisture levels decline.
Table 1 Factorial design of drought experiment.
All substrates included 20% v/v green waste compost (Green Estate, Sheffield), and 80% crushed brick aggregate of either small (fine, 2–5 mm) or large (coarse, 4–15 mm) particles. Dry polyacrylamide gel was then added as 1% of the total substrate volume (manufacturers recommended rate) and substrate added to pots (12 cm × 11 cm × 11 cm) to a level of 120 mm.
Four Festuca ovina and L. vulgaris plug plants (sourced as SkyPlugsTM from Boningale Nurseries Ltd) were planted per pot. Roots were washed before planting to remove any substrate. F. ovina plugs were split into four separate plugs in order to make up the required number. A treatment of Sedum cuttings (mixture of S. album and S. acre) was applied to half of the pots at a density of approximately 100 g m− 2 (1.21 g per pot) to achieve high coverage (>80%) of the substrate.
Planted pots were kept in a controlled greenhouse environment for 3.5 months with a temperature regime reflective of a temperate summer climate (16 hours, 20 °C; 8 hours, 15 °C) to allow plants to mature and Sedum to achieve high coverage of substrate (>80%). Where necessary, supplementary lighting (Helle Lamps, IR 400 HPS, 400 W, 250 μmol/s/m2) was used to ensure the required day length. A watering regime representing an average month in Sheffield, UK (240 ml per pot week− 1 = 80 mm month− 1) was used from planting until drought initiation.
The experiment therefore had a fully factorial design of two plant species (F.ovina and L.vulgaris), brick size (2–5 mm and 4–15 mm), polyacrylamide gel (presence or absence), Sedum treatment (presence or absence) and two drought treatments of each replicates each to make a total of 192 pots (Table ). The 25 day drought treatment was applied once and the control drought did not receive any drought. After the drought treatment ended the initial watering regime was restarted for two weeks in order to provide plants with a recovery period. After the two week drought recovery period shoot biomass was harvested, dried at 80 °C for 48 hours and weighed. Root biomass was removed, washed, dried (80 °C for 48 hours) and weighed from the control drought pots only. Roots from substrates containing polyacrylamide gel were soaked overnight in water in order to expand the gel, which was then manually removed from the roots using a scalpel.
Chlorophyll fluorescence (Fv/Fm)
Physiological health was determined by chlorophyll fluorescence of Photosystem II (PSII). This was quantified using a Walz Mini-PAM photosynthesis yield analyser using the saturation pulse method (Heinz Walz GmbH). Plants were measured every three to four days and at least two hours after sunset in order to ensure full dark adaption. Maximum quantum efficiency of PSII was recorded as the ratio of variable fluorescence to maximum fluorescence (Fv/Fm) each time on randomly selected F. ovina shoots (enough to fill the leaf clip) and on a randomly selected L. vulgaris leaf. This process was repeated once for each plant and therefore four times for each pot. Measurements began the day that drought commenced and continued at 3–4 day intervals until the day before harvesting.
Plant wilting index
On each measurement date the visual health of the plants was assessed with a wilting index adapted from Engelbrecht et al. (Citation2007). A score between zero and five was assigned to the plants in each pot depending on the visual signs of wilting (Table ).
Table 2 Wilt Index scoring system for F. ovina and L. vulgaris. Adapted from (Engelbrecht et al. Citation2007).
Water holding capacity/physical characteristics of substrate
Each pot was weighed daily (excluding weekends) throughout the trial. After the plant harvest each pot was soaked overnight in order to fully saturate the substrate and then allowed to freely drain for fifteen minutes. After which the pots were considered to be at field capacity and weighed. They were then oven dried at 80 °C for four days and weighed again to obtain dry weight. The difference between dry and field capacity weight was taken as the water holding capacity. Thus the rate of water loss via evapotranspiration for each pot (change in weight over time) was calculated as a percentage of total water holding capacity (not calculated to FLL (Citation2008) specifications).
Physical properties of the substrate (water holding capacity, permeability, air, oven and saturated density, pore volume and air content at water holding capacity max) were determined separately according to Forschungsgesellschaft Landschaftsentwicklung Landschaftsbau (FLL) (FLL, Citation2008) methods which are the standard industrial measuring techniques used for green roof substrate.
Temperature of substrate
The temperature of the 25 day drought substrate was measured throughout the trial with remote temperature loggers (Maxim Integrated™ iButton®). These were buried 2 cm below the surface of the substrate in the centre of each pot and programmed to take the temperature every hour to an accuracy of 0.5 °C. Each iButton® was wrapped in Clingfilm to prevent moisture damage.
Statistical analysis
To determine the main factorial effects and interactions of the substrate components on the physical characteristics of the substrate, shoot biomass, root biomass, root:shoot ratio, and substrate temperature, three-way ANOVAs were performed (with polyacrylamide gel presence or absence, brick size and Sedum spp. presence or absence as the fixed factors). Three way repeated measures ANOVAs were performed on the Fv/Fm and wilt index values. Fv/Fm values were Arcsine square root transformed before analysis. L. vulgaris data between days 20–37 was analysed with type III ANOVA due to an unbalanced data set caused by an incorrect watering event. A cumulative link model from the R Package ‘Ordinal’ was used to analyse the wilt index data due to the ordinal nature of the index. The models used to predict water loss were 2nd order polynomial. All statistical analyses were carried out in R Studio version 2.15.1 (22.6.2012), (R Development Core Team, 2011).
Results
Physical characteristics of substrate
Substrate WHC was increased by 56% with polyacrylamide gel but decreased by 21% with large brick compared to small brick (p < 0.0001) (see online supporting information). Permeability was reduced by polyacrylamide gel by 30% (p < 0.01) but increased by large brick by 140% (p < 0.0001). Oven dried and saturated density were reduced by 15% and pore volume increased by 100% by polyacrylamide gel (p < 0.0001) (see Supplementary Online Material).
Shoot biomass
Shoot biomass of both species for both drought treatments was reduced by small brick (25–37%) and Sedum (24–63%), whilst an increase with polyacrylamide gel was observed for both species during the long drought (34–42%) (p < 0.0001), and just F. ovina for the control (16%, p < 0.01) (Table ).
Table 3 Main factor effects and means (three-way ANOVA) for (a) F. ovina shoot biomass after two drought treatments and (b) L. vulgaris biomass after two drought treatments. Main factor means and the % change are shown between the two levels within that factor (e.g. absence vs. presence of SwellGel). Significant factorial interactions are also shown.
Root biomass and root:shoot ratio
Control drought root biomass was reduced by 36% for both species by polyacrylamide gel (Table and b) (p < 0.0001). F. ovina control root biomass was reduced by 26% by large brick (p < 0.0001), although no effect of brick size was observed for L. vulgaris (Table and b). Both species were not affected by the presence of Sedum (Table and b). The control drought root:shoot ratio of both species was reduced by 38–50% by the presence of polyacrylamide gel, small brick or Sedum (Table and d) (p < 0.0001).
Table 4 Main factor effects and means (three-way ANOVA) for (a) F. ovina control pot root biomass (g), (b) L. vulgaris control pot root biomass (g), (c) F. ovina root:shoot ratio, (d) L. vulgaris root:shoot ratio. Main factor means and the % change are shown between the two levels within that factor (e.g. absence vs. presence of SwellGel). Significant factorial interactions are also shown when present.
Chlorophyll fluorescence (Fv/Fm) and wilt index
Control drought
Festuca ovina and L. vulgaris Fv/Fm and wilt index values did not decrease or differ between substrate treatments for the control drought treatments (p>0.05).
Long drought - F. ovina
Festuca ovina showed a decrease in Fv/Fm and wilt index values after 20 days of drought (Figures and ). Fv/Fm and wilt index values after 20 days were higher when polyacrylamide gel (p < 0.0001) or large brick (p < 0.01) were present in the substrate. This increase in Fv/Fm and wilt index values continued after the drought had finished with substrates containing polyacrylamide gel showing a much faster recovery. The presence of Sedum did not affect F. ovina Fv/Fm values (p>0.05) but did reduce wilt index values (p>0.001) throughout the trial (Figures and ).
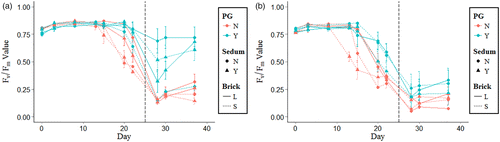
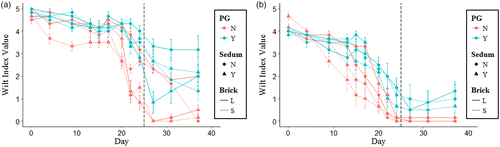
Long drought - L. vulgaris
Linaria vulgaris showed a decrease in Fv/Fm and wilt index values after 13 days of drought (Figure and ). Fv/Fm and wilt index values after 13 days of drought were higher for large brick (p < 0.05) and polyacrylamide gel substrates (p < 0.0001). The increase in Fv/Fm and wilt index values for large brick substrates only occurred between days 13–20, after which no significant effect of large brick substrates was observed (Figures and ). Polyacrylamide gel continued to promote greater Fv/Fm and wilt index values until the end of the drought at day 25 and showed a faster recovery once watering had restarted (Figures and ). The presence of Sedum did not affect L. vulgaris Fv/Fm and wilt index values (p>0.05) throughout the trial (Figures and ).
Drying out curves
The time taken for substrates to dry out from 70% to 20% WHC was increased by 1.6 days for F. ovina and by 1–1.7days for L. vulgaris with polyacrylamide gel and large brick (see Supplementary Online Material). The presence of Sedum also increased time taken to dry out from 70% to 20% WHC by 0.49 days and 1.27 days for F. ovina and L. vulgaris respectively (see Supplementary Online Material).
Substrate temperature
Substrate temperatures for F. ovina were increased by Sedum (0.28–0.37 °C) and large brick (0.46–0.78 °C), whilst temperatures for L. vulgaris were not affected by any substrate factor (see Supplementary Online Material).
Discussion
This study has shown that a polyacrylamide water retention additive (SwellGel) and the use of large crushed brick in green roof substrates can improve the drought tolerance of F. ovina and L. vulgaris. However the addition of Sedum as a companion plant did not improve the drought tolerance of both species despite reducing plant growth. Polyacrylamide gel and brick size differed in their method of increasing drought tolerance, with polyacrylamide gel increasing the WHC of a substrate while only causing a relatively small increase in initial plant growth. The use of large brick instead of small brick led to slower and more sustainable plant growth during the ambient watering regime before the drought, leading to greater tolerance during drought.
Polyacrylamide gel
Substrates containing polyacrylamide gel maintained plant Fv/Fm and wilt index values at significantly higher levels throughout the drought with plants showing faster recovery once watering had commenced again. The mechanism behind this trend is likely due to the large increase in WHC which increased the amount of plant available water (Farrell et al., Citation2013; Savi & Marin, Citation2014). In addition polyacrylamide gel did not promote large amounts of extra biomass growth during ambient watering conditions (control drought) which is harder to maintain during drought conditions (Bates et al., Citation2013; Nagase & Dunnett, Citation2011), and also transpires more, reducing the time taken for the available water reservoir to be exhausted (Wolf & Lundholm, Citation2008; Young et al., Citation2014). ‘Although the time taken for dry down to 20% WHC was only increased by 1–1.7 days by polyacrylamide gel, this translated to a positive increase of 0.15–0.25 in Fv/Fm values after 25 days of drought. F. ovina with polyacrymide gel present still showed Fv/Fm values of 0.6 after 25 days of drought, indicating that plants were generally still healthy and only experiencing the early stages of stress (Ritchie, Citation2006).’
Previous studies have shown that polyacrylamide gel in green roof substrate did not have an effect on the shoot growth of Triticum aestivum and Lupinus albus at 0.1% v/v during drought conditions (Farrell et al., Citation2013) but did increase Sedum spp. growth (Olszewski et al., Citation2010) and Salvia officinalis (Savi & Marin, Citation2014) during ambient watering conditions at 0.05–0.22% v/v application. Therefore the effect of polyacrylamide gel on plant growth may depend on availability of water in the substrate (e.g. drought conditions).
Penetration of polyacrylamide gel by roots was observed for both species and therefore it was assumed that the majority of water held within the gels was available to the plants (Farrell et al., Citation2013; Savi & Marin, Citation2014; Woodhouse & Johnson, Citation1991). However root biomass and root:ratio was reduced by polyacrylamide gel for both species which agrees with previous trials (Farrell et al., Citation2013; Young et al., Citation2014). It is not clear whether this is a direct impact of the polyacrylamide gel or an artefact of the process of manually removing polyacrylamide gel from roots (Young et al., Citation2014).
The impact of polyacrylamide gels is also strongly dependent upon the rate of application. This study used an application rate of 1% v/v which is higher than most previous studies (0.1–0.6% v/v) (Farrell et al., Citation2013; Geesing & Schmidhalter, Citation2004; Olszewski et al., Citation2010; Savi & Marin, Citation2014). Applying polyacrylamide gels at the higher rate will increase the cost of a substrate, but this extra cost needs to be balanced out against the clear benefits of 1% addition, compared to lower rates (especially 0.1%) that do not always provide marked benefits (Farrell et al., Citation2013). More research is needed to find an optimal application rate for green roof substrate.
This trial looked at the short term effect of polyacrylamide gel on recently established plants during a single drought and therefore their long term effectiveness in green roof substrates is still unknown. Polyacrylamide gels are known to degrade and rapidly lose their WHC after 18 months due to UV light exposure, freeze thaw cycles, elevated temperatures and microbial/fungal activity (Holliman et al., Citation2005; Smith et al., Citation1997, Citation1996). Therefore although polyacrylamide gel may initially increase plant drought tolerance, it will likely lose its ability to store water at around 1–9% year− 1 (Al-Harbi et al., Citation1999; Hüttermann et al., Citation2009; Savi & Marin, Citation2014).
Overall polyacrylamide gel may still be an attractive option to improving the drought tolerance of newly established green roof plants. Given this is likely to be the most vulnerable stage of a green roof's plant coverage, even this early benefit can justify the extra cost of polyacrylamide gel, especially if seedlings/propagules are particularly vulnerable. This benefit may be increased further where polyacrylamide gel increases substrate bacterial and fungal populations (Li et al., Citation2014) as well as plant available nitrogen (Kay-Shoemake et al., Citation1998; Young et al., Citation2014). Despite this, other water retention additives such as silicate granules should not be discounted, and may be more effective at providing water to plants as they can increase water availability more homogeneously throughout the substrate (Farrell et al., Citation2013).
Brick particle size
Substrates containing large brick particles maintained plant Fv/Fm and wilt index values at significantly higher levels throughout the drought, with F. ovina showing faster drought recovery. However this effect was less pronounced than for polyacrylamide gel and L. vulgaris was only benefited by large brick in the earlier stages of the drought (days 12–20).
The ability of large brick to improve Fv/Fm and wilting index values during drought compared to small brick shows that an increase in substrate WHC does not always result in increased drought tolerance. By reducing the amount of water available to plants during ambient watering conditions as well providing a coarse growing media, plants grew at a much more sustainable rate and therefore were more resistant to drought (Bates et al., Citation2013; Hermans et al., Citation2006; Poorter & Nagel, Citation2000). The reduced amount of shoot biomass and increased root:shoot ratio for plants grown in large brick substrates also reduced evapotranspiration rates which increased the length of time for water reservoirs to be exhausted (Berretta et al., Citation2014; Nagase & Dunnett, Citation2011; Young et al., Citation2014). This highlights the danger of focusing on one aspect of substrate design and when seeking a substrate suitable for drought tolerance, substrates should not be selected for physical properties alone, but also the impact they have upon plant growth (Young et al., Citation2014). Although not as effective as polyacrylamide gel, the use of coarser substrates with a lower WHC should be considered as a cheaper and potentially longer term method of tailoring green roof substrates for plant drought tolerance.
Sedum cover
Despite reducing the growth of both plant species, Sedum did not have any effect on their drought resistance. Previous studies have shown that the presence of Sedum can improve substrate moisture content by reducing evapotranspiration rates (Durhman et al., Citation2006; VanWoert et al., Citation2005; Wolf & Lundholm, Citation2008) and mat forming species can reduce substrate temperature (Heim et al., Citation2014; Heim & Lundholm, Citation2014). Although Butler and Orians (Citation2011) have previously shown that Sedum can facilitate the appearance of neighbouring plants during drought, this current trial shows that the negative competitive effects of Sedum outweigh any potential benefits of this species as a companion plant.
Fv/Fm and wilt index
Although not as accurate or quantitative as chlorophyll fluorescence, the wilt index used in this trial showed good association with Fv/Fm values. Chlorophyll fluorescence is an extremely useful tool to quantify the level of stress in plants (Baker & Rosenqvist, Citation2004; Ritchie, Citation2006) but uses specialist equipment that many laboratories/nurseries do not have access to. Our work with Fv/Fm supports the use of the wilt index as a quick, easy and accurate method for quantifying water stress in plants.
Conclusions
This plant establishment study suggests a combination of strategies can be used to increase drought resistance of green roofs to increase water supply, decrease water demand and increase plant resilience.
The use of polyacrylamide gels on green roofs is not common but this trial has shown that they can increase the drought tolerance of perennial green roof plants by increasing the amount of water available during drought whilst not promoting excessive shoot growth. The use of larger sized substrate particles can also increase drought tolerance by promoting slower and therefore more sustainable plant growth. The use of Sedum as a nurse plant did not significantly improve plant drought resistance in this trial but may still be effective at facilitating companion plant growth in certain green roof environments.
This study only measured the benefits of polyacrylamide gel at a high 1% v/v application rate during establishment, i.e., over 3.5 months and a single drought. In addition the effectiveness of polyacrylamide gels is likely to decrease over time and over multiple wet-dry cycles needs and to be investigated further. However it is still apparent that polyacrylamide gels can be used as a tool for increasing the drought tolerance of green roof plants.
We propose that a more cost effective and potentially longer term method of increasing green roof plant drought tolerance is to use substrates that promote slower and more sustainable plant growth i.e. use of coarser particle sizes.
Acknowledgements
We are grateful to Despina Berdeni and Irene Johnson for their research and technical support.
Disclosure statement
No potential conflict of interest was reported by the authors.
Additional information
Funding
References
- Al-Harbi, A.R., Al-Omran, A.M., Shalaby, A.A., and Choudhary, M.I., 1999. Efficacy of a Hydrophilic Polymer Declines with Time in Greenhouse Experiments. HortScience, 34, 223–224.
- Baker, N.R. and Rosenqvist, E., 2004. Applications of chlorophyll fluorescence can improve crop production strategies: an examination of future possibilities. Journal of Experimental Botany, 55, 1607–1621.
- Bates, A.J., Sadler, J.P., and Mackay, R., 2013. Vegetation development over four years on two green roofs in the UK. Urban Forestry & Urban Greening, 12, 98–108.
- Berretta, C., Poë, S., and Stovin, V., 2014. Moisture content behaviour in extensive green roofs during dry periods: The influence of vegetation and substrate characteristics. Journal of Hydrology, 511, 374–386.
- Blanusa, T., Vaz Monteiro, M.M., Fantozzi, F., Vysini, E., Li, Y., and Cameron, R.W.F., 2013. Alternatives to Sedum on green roofs: Can broad leaf perennial plants offer better ‘cooling service’? Building and Environment, 59, 99–106.
- Butler, C. and Orians, C.M., 2011. Sedum cools soil and can improve neighboring plant performance during water deficit on a green roof. Ecological Engineering, 37, 1796–1803.
- Castleton, H.F., Stovin, V., Beck, S.B.M., and Davison, J.B., 2010. Green roofs; building energy savings and the potential for retrofit. Energy and Buildings, 42, 1582–1591.
- Dunnett, and Kingsbury, 2010. Planting Green Roofs and Living Walls. 2nd edn. Portland: Timber Press.
- Dunnett, N., Nagase, A., and Hallam, A., 2008. The dynamics of planted and colonising species on a green roof over six growing seasons 2001–2006: influence of substrate depth. Urban Ecosystems, 11, 373–384.
- Durhman, A.K., Rowe, D.B., and Rugh, C.L., 2006. Effect of Watering Regimen on Chlorophyll Fluorescence and Growth of Selected Green Roof Plant Taxa. HortScience, 41, 1623–1628.
- Durhman, A.K., Rowe, D.B., and Rugh, C.L., 2007. Effect of Substrate Depth on Initial Growth, Coverage, and Survival of 25 Succulent Green Roof Plant Taxa. HortScience, 42, 588–595.
- Dvorak, B. and Volder, A., 2010. Green roof vegetation for North American ecoregions: A literature review. Landscape and Urban Planning, 96, 197–213, doi:10.1016/j.landurbplan.2010.04.009.
- Engelbrecht, B.M.J., Tyree, M.T., and Kursar, T.A., 2007. Visual assessment of wilting as a measure of leaf water potential and seedling drought survival. Journal of Tropical Ecology, 23, 497–500.
- Farrell, C., Ang, X.Q., and Rayner, J., 2013. Water-retention additives increase plant available water in green roof substrates. Ecological Engineering, 52, 112–118.
- Fassman-Beck, E., Voyde, E., Simcock, R., and Hong, Y.S., 2013. 4 Living roofs in 3 locations: Does configuration affect runoff mitigation? Journal of Hydrology, 490, 11–20.
- FLL, 2008. Guidelines for the Planning, Construction and Maintenance of Green Roofing. FLL: The Landscape Development and Landscaping Research Society.
- Geesing, D. and Schmidhalter, U., 2004. Influence of sodium polyacrylate on the water-holding capacity of three different soils and effects on growth of wheat. Soil Use and Management, 20, 207–209.
- Getter, K.L. and Rowe, D.B., 2006. The Role of Extensive Green Roofs in Sustainable Development. HortScience, 41, 1276–1285.
- Getter, K.L. and Rowe, D.B., 2008. Media depth influences Sedum green roof establishment. Urban Ecosystems, 11, 361–372.
- Getter, K.L. and Rowe, D.B., 2009. Substrate Depth Influences Sedum Plant Community on a Green Roof. HortScience, 44, 401–407.
- Graceson, A., Hare, M., Monaghan, J., and Hall, N., 2013. The water retention capabilities of growing media for green roofs. Ecological Engineering, 61 (part A), 328–334.
- Heim, A. and Lundholm, J., 2014. Species interactions in green roof vegetation suggest complementary planting mixtures. Landscape and Urban Planning, 130, 125–133, doi:10.1016/j.landurbplan.2014.07.007.
- Heim, A., Lundholm, J., and Philip, L., 2014. The impact of mosses on the growth of neighbouring vascular plants, substrate temperature and evapotranspiration on an extensive green roof. Urban Ecosystems, 1–15, doi:10.1007/s11252-014-0367-y.
- Hermans, C., Hammond, J.P., White, P.J., and Verbruggen, N., 2006. How do plants respond to nutrient shortage by biomass allocation? Trends In Plant Science, 11, 610–617.
- Holliman, P.J., Clark, J.A., Williamson, J.C., and Jones, D.L., 2005. Model and field studies of the degradation of cross-linked polyacrylamide gels used during the revegetation of slate waste. The Science of the Total Environment, 336, 13–24.
- Hüttermann, A., Orikiriza, L.J.B., and Agaba, H., 2009. Application of Superabsorbent Polymers for Improving the Ecological Chemistry of Degraded or Polluted Lands. CLEAN – Soil, Air, Water, 37, 517–526.
- Kay-Shoemake, J.L., Watwood, M.E., Lentz, R.D., and Sojka, R.E., 1998. Polyacrylamide as an organic nitrogen source for soil microorganisms with potential effects on inorganic soil nitrogen in agricultural soil. Soil Biology and Biochemistry, 30, 1045–1052.
- Li, X., He, J.-Z., Hughes, J.M., Liu, Y.-R., and Zheng, Y.-M., 2014. Effects of super-absorbent polymers on a soil–wheat (Triticum aestivum L.) system in the field. Applied Soil Ecology, 73, 58–63.
- Lundholm, J., MacIvor, J.S., MacDougall, Z., and Ranalli, M., 2010. Plant Species and Functional Group Combinations Affect Green Roof Ecosystem Functions. PLoS ONE, 5, e9677.
- Nagase, A. and Dunnett, N., 2011. The relationship between percentage of organic matter in substrate and plant growth in extensive green roofs. Landscape and Urban Planning, 103, 230–236.
- Nagase, A., Dunnett, N., and Choi, M.-S., 2013. Investigation of weed phenology in an establishing semi-extensive green roof. Ecological Engineering, 58, 156–164.
- Oberndorfer, E., Lundholm, J., Bass, B., Coffman, R., Doshi, H., Dunnett, N., Gaffin, S., Köhler, M., Liu, K., and Rowe, B., 2007. Green Roofs as Urban Ecosystems: Ecological Structures, Functions, and Services. BioScience, 57, 823–833.
- Olszewski, M.W., Holmes, M.H., and Young, C.A., 2010. Assessment of Physical Properties and Stonecrop Growth in Green Roof Substrates Amended with Compost and Hydrogel. HortTechnology, 20, 438–444.
- Poorter, H. and Nagel, O., 2000. The role of biomass allocation in the growth response of plants to different levels of light, CO2, nutrients and water: a quantitative review. Functional Plant Biology, 27, 595–607.
- Ritchie, G.A., 2006. Chlorophyll fluorescence: What is it and what do the numbers mean? USDA Forest Service Proceedings, RMRS-P-43, 34–43.
- Rowe, D.B., Getter, K.L., and Durhman, A.K., 2012. Effect of green roof media depth on Crassulacean plant succession over seven years. Landscape and Urban Planning, 104, 310–319.
- Rowe, D.B., Monterusso, M.A., and Rugh, C.L., 2006. Assessment of Heat-expanded Slate and Fertility Requirements in Green Roof Substrates. HortTechnology, 16, 471–477.
- Savi, T. and Marin, M., 2014. Green roofs for a drier world: Effects of hydrogel amendment on substrate and plant water status. Science of The Total Environment, 490, 467–476.
- Smith, E.A., Prues, S.L., and Oehme, F.W., 1996. Environmental degradation of polyacrylamides. 1. Effects of artificial environmental conditions: temperature, light, and pH. Ecotoxicology and Environmental Safety, 35, 121–135.
- Smith, E.A., Prues, S.L., and Oehme, F.W., 1997. Environmental degradation of polyacrylamides. II. Effects of environmental (outdoor) exposure. Ecotoxicology and Environmental Safety, 37, 76–91.
- Snodgrass, E.C. and Snodgrass, L., 2006. Green Roof Plants - A Resource and Planting Guide. Portland: Timber Press.
- Stovin, V., 2010. The potential of green roofs to manage Urban Stormwater. Water and Environment Journal, 24, 192–199.
- Thuring, C.E., Berghage, R.D., and Beattie, D.J., 2010. Green Roof Plant Responses to Different Substrate Types and Depths under Various Drought Conditions. HortTechnology, 20, 395–401.
- VanWoert, N., Rowe, D.B., Andresen, J.A., Rugh, C.L., Fernandez, R.T., and Xiao, L., 2005. Green roof stormwater retention: effects of roof surface, slope, and media depth. Journal Environment Quality, 34, 1036–1044.
- Wolf, D. and Lundholm, J.T., 2008. Water uptake in green roof microcosms: Effects of plant species and water availability. Ecological Engineering, 33, 179–186.
- Woodhouse, J. and Johnson, M.S., 1991. Effect of superabsorbent polymers on survival and growth of crop seedlings. Agricultural Water Management, 20, 63–70.
- Young, T., Cameron, D.D., Sorrill, J., Edwards, T., and Phoenix, G.K., 2014. Importance of different components of green roof substrate on plant growth and physiological performance. Urban Forestry & Urban Greening, 13, 507–516, doi:10.1016/j.ufug.2014.04.007.