Abstract
Separation of wastewater split streams and utilization of the resources contained therein are an important prerequisite for a sustainable wastewater treatment. In areas with existing infrastructures, this leads to transition states during which current systems must continue to operate. A simple mass-flow based method was developed to assess the impacts of an incremental transition (co-digestion of blackwater and sewage sludge) upon plant operation. The results proved that blackwater co-digestion could be easily integrated into existing wastewater infrastructures; however, beyond 8% transition thickening was required due to hydraulic limitations in the digester. Additionally, nitrogen recovery was necessary beyond 35% transition to avoid unfavorable C:N ratios. The investigated concept also led to a power gain of 11 kWh/(PE∙a) due to enhanced biogas production and aeration savings. The developed Excel-based method was considered suitable for evaluating the benefits and probable tipping points for plant operation during transition to source-separated sanitation systems.
Introduction
Transition to sustainable urban systems is only possible through integrating and closing water, energy and material flows (van der Hoek et al. Citation2015). Slagstad and Brattebø (Citation2013) found out that among water and wastewater infrastructures wastewater treatment plants (WWTPs) contributed most significantly to the total environmental impact mostly due to high requirements of energy and chemicals. For instance, conventional phosphorous removal often uses costly chemicals such as ferric chloride, alum and polymers (Tchobanoglous et al. Citation2014). Indeed, in many municipalities wastewater facilities are the largest electricity consumers (Nowak et al. Citation2015) mostly because of energy consumption for pumping and aeration (Muga and Mihelcic Citation2008) - particularly the conventional nitrogen removal is energy-intensive (Lackner et al. Citation2014). Although in some countries many efforts have been undertaken to improve the energy balance of WWTPs, these usually do not directly address the recovery of resources in municipal wastewater (energy, nutrients and water) as wastewater is typically regarded as merely being an energy sink.
Nutrient recovery is not state-of-the-art at WWTPs, yet it has been tested in both lab and pilot-scale. Some authors (e.g. Wang and Peng Citation2010, Am Yusof et al. Citation2010) have suggested application of zeolites in the wastewater treatment because of their capability to adsorb ammonium, while being abundant and inexpensive (the ion-exchange properties of zeolites are well described in the scientific literature, e.g. Wang and Peng Citation2010). Other authors have proposed nutrient recovery through microalgae cultivation technologies (e.g. Cai et al. Citation2013); these are capable of incorporating nitrogen and phosphorus (required for cell growth) from wastewater under controlled environments. However, the high flow rates and low nutrient concentrations of conventional wastewater has hindered a large-scale implementation of these technologies. Phosphorus can be recovered through chemical precipitation from WWTPs as struvite or calcium phosphate (Driver et al. Citation2010) but current approaches for phosphorus recovery from sludge liquor only bring about low recovery rates (e.g. Cornel and Schaum Citation2009) or involve the use of costly chemicals to redissolve metal phosphates by acidic leaching of digested sludge (cf. Meyer et al. Citation2015). In view of all that, separate collection and selective treatment of blackwater would likely improve the applicability of current techniques and help overcome technical difficulties.
Resource-oriented systems prioritize water reuse, energy recovery and recycling of wastewater constituents, e.g. phosphorous, nitrogen, organic substances, through separate collection and selective treatment of wastewater split streams (WSWU and DWA Citation2016). Apart from relatively low flow rates, it was shown that blackwater accounts for 67% BOD5, 52% COD, 92% Ntot and 75% Ptot in domestic wastewater (DWA Citation2014b). Thus, blackwater is more suitable for anaerobic treatment (biogas production and energy use) and nutrient recovery than wastewater.
Many authors have suggested on-site or semi-centralized blackwater digestion (e.g. Wendland et al. Citation2007, de Graaff et al. Citation2010, Zeeman and Kujawa-Roeleveld Citation2013). With respect to treatment, Wendland et al. (Citation2007) reported a COD removal of 61% in an anaerobic lab-scale continuously stirred tank reactor treating concentrated blackwater (T = 37 °C; HRT = 20 d). This implies that blackwater digestion is a suitable treatment technology but alone not sufficient to comply with legal requirements for the wastewater discharge.
With regard to greywater, decentralized treatment and recycling have been successfully implemented in several rural areas worldwide. Pidou et al. (Citation2007) analyzed numerous studies In addition, Penn et al. (Citation2012) reported 26–41% reduction of the daily household wastewater flows by reuse of light greywater. Recycling of treated greywater for toilet flushing was shown to be an efficient method for minimization of drinking water consumption in a densely populated semi-urban area (cf. Zhang et al. Citation2009).
Within some pilot projects, the infrastructure to collect blackwater at low dilution has been implemented over the last decade (Nowak et al. Citation2015) but mostly within new settlements (decentralized systems). Indeed, processes and ideas for source-separated sanitation systems have already been thoroughly discussed (e.g. Kaufmann Alves Citation2013, Kjerstadius et al. Citation2015); however, centralized transition concepts, probable transition pathways and impacts upon plant operation during transition have thus far not been evaluated in any study.
Particularly in countries where centralized wastewater infrastructures with long service lives are present, an incremental shift from conventional to resource-oriented systems will have to be undertaken eventually, for instance through the treatment of concentrated blackwater in anaerobic digesters to improve biogas production and nutrient recovery. In order to avoid treatment failure the operation of the centralized WWTP must be investigated and assessed during different transition states.
This study proposed the direct conveyance of blackwater through vacuum sewers to municipal digesters, based on the knowledge that in the EU anaerobic sludge digestion is used in 24 of the 27 member states (Kelessidis and Stasinakis Citation2012) and that many digesters are overdimensioned, so hydraulic capacities for co-digestion exist (Dichtl and Schmelz Citation2015). To the best of the authors’ knowledge, no one has so far considered a transition concept in which blackwater is co-digested in municipal digesters alongside primary and excess sludge. Critical transition states in which operating problems may arise were assessed and discussed. Additionally, changes in energy demand, biogas production and nutrient reuse were quantified for the transition concepts proposed. For that a simplified mass-flow based algorithm has been developed, so that comparisons among different transition scenarios could be drawn and critical tipping points assessed, after which conventional systems would not operate satisfactorily without altering process conditions significantly.
Due to possible operating bottlenecks during transition to source-separated sanitation such as wastewater stagnation, hydraulic overloading in the anaerobic stage, C:N shift in the anoxic stage or even underload-related problems in the aerobic stage, etc., which can be further aggravated by decoupling greywater, a very thorough analysis has been undertaken within this study. Also, corrective actions were proposed in order to assure a safe and stable plant operation as well as good cleaning efficiencies (the main objective of a wastewater treatment facility).
Materials and methods
Within this study impacts of an incremental blackwater co-digestion and greywater recycling upon the functionality of a conventional wastewater treatment plant were investigated using mass and volume balances for different transition states. “Transition” was defined as the fraction of inhabitants within a catchment area using vacuum toilets for direct blackwater discharge to municipal digesters of WWTPs whereas for greywater two cases were investigated:
(1) | Residual wastewater transport via the existing combined sewer to the aerobic stage of the WWTP (case one). | ||||
(2) | Greywater decoupling from the plant and decentralized treatment (case two). |
Sources and assumptions used for the mass and volume flow balances are listed in Table . The inhabitant-specific balances (applicable to all WWTPs with anaerobic sludge stabilization) are mainly founded on typical data from literature (cf. Table ), yet the lack of representative data with regard to the transition to source-separated sanitation was the most challenging problem within this study due to insufficient experience in the research field. These difficulties could be overcome by using own data (where applicable) and simplifying a few calculation processes (cf. Table ). For clarity a value of 100,000 PE was set as a reference (model WWTP). The loads used are 85 percentile values. In the model WWTP a nitrification and an upstream denitrification stage as well as a digester for separate sewage sludge stabilization with available hydraulic reserves (HRTactual = 25 d; HRTdesired = 20 d) were assumed.
Table 1. Assumptions, calculations and references for mass and volume balances in the actual state if not otherwise stated.
The mass-flow method proposed is an Excel-based algorithm which comprises iterative calculations coupled either with load or flow rate values (for further information, cf. Table ). For instance, loads and volumes of separated blackwater and greywater vary with increasing transition, which leads to changes in volume and composition of further streams such as WWTP influent, excess and primary sludge, influent to the digester etc. Infiltration water was assumed to be constant. The chargeback of dewatering and thickening stages was considered into the calculations, as the chargeback tends to increase with increasing transition.
Figure depicts the actual state of the model WWTP with specific COD, Ntot and Ptot loads and flow rates, thus allowing a more comprehensive understanding of the relationships among the different parameters stated in Table . The total solids contents were occasionally indicated to clarify the volume flow distribution after thickening or dewatering and assumed constant for several streams such as blackwater, primary and excess sludge, so flow rates varied accordingly within the transition scenarios. It is important to note that the bars merely provide a qualitative understanding (two scales depicted), whereas the absolute values allow any quantitative analysis.
Figure 1. Mass and volume balances for the actual state (0% transition) of a conventional WWTP, with: PC = primary clarifier; DN = denitrification stage; SC = secondary clarifier; ST = sewage sludge thickener; DGT = digester; FP = chamber filter press (for sewage sludge dewatering); PS = primary sludge; ES = excess sludge; RS = raw sludge; DS = digested sludge; SL = sludge liquor (chargeback).
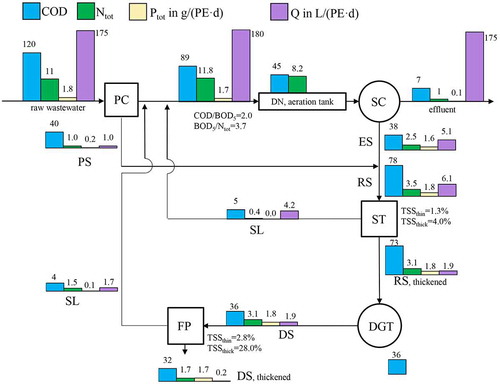
Results
Process stability
Based on the mass balances it could be shown for case one (blackwater co-digestion and treatment of residual wastewater including greywater in the activated sludge tank) that the existing infrastructure is capable of operating normally up to approx. 8% transition (i.e. for the model WWTP up to a blackwater co-digestion of 8000 PE); after this point the digester’s hydraulic reserves are exhausted. This effect was ascribed to the higher volume flows of blackwater – 7 L/(PE∙d) at 0.9% TSS – compared to pre-thickened sewage sludge – approx. 1.9 L/(PE∙d) at 4% TSS. As a result, a few structural alterations of the WWTP must be undertaken from 8% transition onwards. A static or mechanical thickener, e.g. settling tank, emerges as an alternative, as preliminary lab-investigations showed that blackwater has better settleability than conventional excess sludge.
Attaining an unfavorable BOD5:Ntot ratio in the aerobic stage may be a critical point for plants undergoing transition because of the displacement of BOD5 to the anaerobic stage. This proved critical at 35% blackwater separation (for the model WWTP 35,000 PE). BOD5:Ntot ratios < 3.5 would be expected hereafter, as can be inferred from Figure . An incomplete denitrification would rapidly reflect in increasing nitrate effluent values, which has to be avoided. Denitrification problems can be counteracted by sidestream N recovery processes (processes implemented on N-rich sidestreams within sewage sludge processing lines), as these emerge as an alternative to conventional N removal and contribute to resource-efficiency in wastewater treatment. Minimum nitrogen recovery rates of 20% (in terms of N in both sludge liquor and blackwater supernatant after thickening) have proved to be sufficient to not compromise the functionality of the nitrogen removal stage in the WWTP up to 100% transition.
Figure 2. Mass and volume balances for 35% blackwater separation (e.g. 35,000 PE out of 100,000 PE) of a conventional WWTP undergoing transition to new sanitation technologies, with: BW = blackwater; BT = blackwater thickener, cf. Figure 1 for remaining legends.
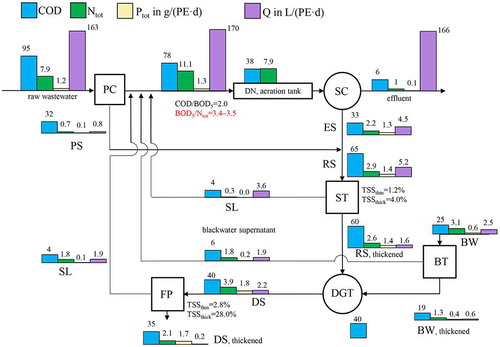
It can be inferred from Figure that blackwater thickening enables additional blackwater loading up to approx. 75,000 PE (at 4% TSS). Assuming 5% TSS in thickened sludge volume balances proved blackwater co-digestion possible up to 100% blackwater separation.
Figure 3. Mass and volume balances for 75% blackwater separation (e.g. 75,000 PE out of 100,000 PE) of a conventional WWTP undergoing transition to new sanitation technologies, with: BW = blackwater; BT = blackwater thickener; N = nitrogen recovery, cf. Figure 1for remaining legends.
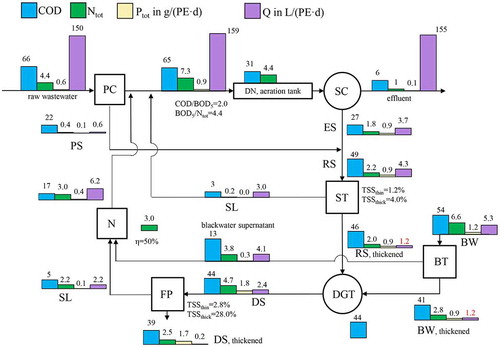
In case two, blackwater was treated in the digester (like case one), yet decentralized treatment and recycling of greywater was considered. Thus, less BOD5 reached the WWTP influent, deteriorating denitrification at an earlier transition state (15,000 rather than 35,000 PE). This required, at 75% transition, a minimum N recovery rate of 50% in sludge liquor and blackwater supernatant for maintenance of a favorable BOD5:Ntot ratio for denitrification, which is higher than in case one.
A summary of the tipping points for plant operation and respective corrective measures to overcome these problems is given in Table .
Table 2. Transitions states with respective tipping points for plant operation and corrective measures to overcome operating problems.
Energy demand and energy production
Aeration in the aerobic stage is the principal power consumer in WWTPs. By displacing carbon and nitrogen (contained in blackwater) to the anaerobic stage, aeration requirements could be greatly reduced, as depicted in Figure (for both case one and two).
Figure 4. Expected developments of power demand for aeration and power generation from biogas in a conventional WWTP for cases one and two.
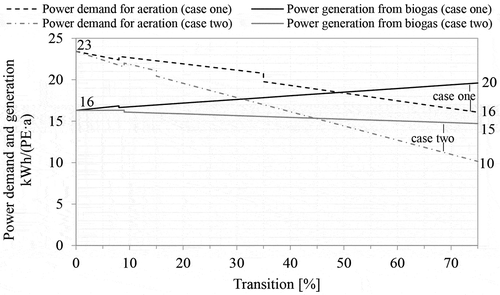
For case one, it was observed that the energy demand for aeration could be reduced in total by approx. 7.5 kWh/(PE·a) at 75% transition, which corresponded to 30% of the actual state. It can be inferred from Figure that a slightly heightened power demand for aeration of approx. 0.5 kWh/(PE·a) was observed immediately after the set-up of blackwater thickening at 8% transition due to recirculation of the blackwater thickening supernatant. This had only a marginal impact on biogas production. Furthermore, with the implementation of a nitrogen recovery process (η = 50%) from both sludge liquor and blackwater supernatant at 35% transition, the power required for aeration fell abruptly from approx. 21 to 20 kWh/(PE·a), as can be seen in Figure . In parallel the power generation could be increased to approx. 3.5 kWh/(PE·a) in total, which is a 20% increase on the actual state, so a total energy gain of 11 kWh/(PE·a) could be achieved. All in all, WWTPs may become energy producers rather than consumers, while greatly decreasing their environmental impact.
For case two, Figure shows that under energy aspects decoupling of greywater from the WWTP provided no benefits other than those observed in case one. While in the course of transition energy requirements for aeration sank relatively rapidly from 23 to 10 kWh/(PE·a) due to both nitrogen recovery and further load decoupling from the activated sludge process, no increased biogas production was reported (in view of reduced amounts of primary sludge and excess sludge) but rather 10% reduction in power generation at 75% transition, in contrast to case one. Nevertheless, within an overall wastewater management scheme the decentralized treatment and usage of greywater might have further advantages.
Additionally, it was shown for case one that heat production from biogas increased from 30 to 36 kWh/(PE·a) at 75% transition; yet, the energy required for sludge heating also increased from 20 to 25 kWh/(PE·a) due to higher substrate volumes at the digester’s inlet, so no net thermal energy gain can be expected.
Nutrient recovery
Nitrogen recovery
In order to further comply with nitrogen regulation standards from 35% transition onwards, nitrogen recovery processes from N-rich sidestreams (from both sludge liquor and blackwater supernatant after set-up of blackwater thickening) were considered. Figure shows that the total N recovery potential increases with increasing transition. At 35% transition, a critical point for the WWTP operation, a nitrogen removal efficiency of ƞ = 60% (in terms of N in sludge liquor and blackwater supernatant) brought about a recovery rate of approx. 20% (in terms of N load to the WWTP) or correspondingly 2.2 g NH4-N/(PE·d). An efficiency of 30% yielded 1.1 g NH4-N/(PE·d). The results show that nitrogen can be partially recovered during transition to resource-oriented sanitation systems, which implies that mineral fertilizers can be partially substituted with recycling products from WWTPs. Additionally, the energy demand and costs for aeration can be significantly decreased.
Figure 5. Total nitrogen recovery potential and recovery ratesFootnote 1 at two different recovery efficiencies.
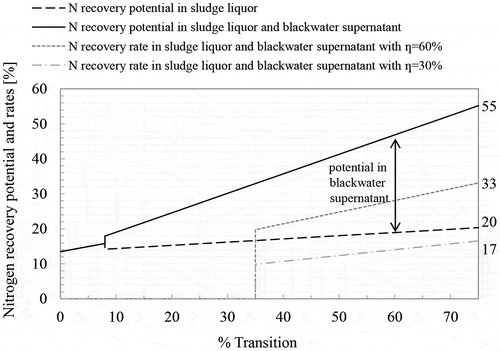
Phosphorus recovery
In contrast to nitrogen, phosphorus recovery is not essential for the functionality of the WWTP in the long-term, because it can be eliminated for instance by chemical processes. Nevertheless, phosphorus is a finite resource and other ways rather than unsustainable extraction from rock phosphate must be considered in the mid-term.
Figure depicts phosphorus potentials in both digested sludge and blackwater supernatant (after blackwater thickening set-up). As P accumulates in digested sludge, theoretical P recovery potentials in digested sludge are very high; however, recovery rates are in fact proportional to the extent of P release e.g. by acidic leaching of digested sludge. A total recovery efficiency of 40–60% (in terms of P in digested substrate and blackwater supernatant) was assumed. This is paralleled by a P recovery rate of 38–57% (about 0.7–1 g PO4-P/(PE·d)). All in all, relatively high phosphorus recovery potentials were reported, if the P fraction contained in the blackwater supernatant is considered.
Figure 6. Total phosphorus recovery potential and recovery ratesFootnote 2 at two different recovery efficiencies.
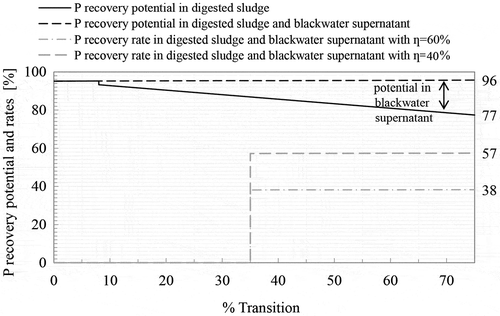
With respect to nutrient utilization, greywater decoupling from the plant (case two) reported just slightly lower P and N recovery rates, as greywater retains only a minor fraction of the N and P found in domestic wastewater (cf. DWA Citation2014b). Therefore, greywater decoupling is not discussed in this section.
The results of the algorithm proposed corroborate the applicability of different transition concepts, in which blackwater is co-digested alongside primary and excess sludge in centralized WWTPs. These emphasize that the centralized WWTP can be easily integrated into different transitions concepts, while benefits with regard to energy efficiency and nutrient recovery can be achieved. It was shown that a simple Excel-based model is suitable for evaluating the benefits and probable tipping points for plant operation during transition to resource-oriented systems.
Conclusions
It is evident that resource-oriented systems are a necessity for longer-term wastewater treatment. The mass-flow based method developed has proved to be appropriate for assessing probable impacts of existing WWTPs undergoing transition to resource-oriented systems upon plant operation. It is the first time that a simple mass-flow based method has been developed to pave sustainable transition pathways to source-separated sanitation. Under consideration of specific boundary conditions this method can be applied to any WWTP undergoing transition.
Additionally, it was shown that co-digestion of blackwater alongside primary and excess sludge is an advantageous transition concept for improving the energy balance and nutrient recovery potentials in municipal WWTPs despite the wide palette of new technical solutions, yet hydraulic reserves must be available in the digester. Also, transition is likely to be achieved with only a few process and structural alterations in the plant, e.g. set-up of nitrogen recovery and blackwater thickening. However, more comprehensive alterations in the sewer system may be required.
The findings of this study are a preliminary attempt (mainly due to the large number of assumptions made and the simplicity of the mass-flow based method reported) to assess when and where operating problems will likely occur and which benefits can be expected therefrom. A deeper analysis is only viable by consideration of real boundary conditions.
Due to the requirement of an incremental set-up of a vacuum sewer, future research should also contemplate energy balances within both the sewer system and the WWTP rather than the latter only. Additionally, transition concepts including further scenarios and technologies other than blackwater co-digestion should be assessed in future works.
Disclosure statement
No potential conflict of interest was reported by the authors.
Funding
This work was supported by the German Federal Ministry of Education and Research (BMBF) under Grant 033W011D.
Notes
1. Referred to nitrogen load to the WWTP, i.e. 11 g/(PE·d).
2. Referred to phosphorus load to the WWTP, i.e. 1.8 g/(PE·d).
References
- AbwV. , 2004. Verordnung über Anforderungen an das Einleiten von Abwasser in Gewässer. Anhang 1 Häusliches und kommunales Abwasser. German . German wastewater regulation on requirements for wastewater discharge into waterbodies. Appendix 1 Domestic and Municipal Wastewater. Available from: http://www.gesetze-im-internet.de/abwv/anhang_1.html
- ATV-DVWK , 2000a. Bemessung von einstufigen Belebungsanlagen .Dimensioning of single-stage biological wastewater treatment. Hennef: ATV-DVWK, Deutsche Vereinigung für Wasserwirtschaft, Abwasser und Abfall; Abwassertechnische Vereinigung. German
- ATV-DVWK , 2000b. Rückbelastung aus der Schlammbehandlung - Menge und Beschaffenheit der Rückläufe. 1 . Arbeitsbericht.Chargeback from the sludge treatment - Quantity and quality of return streams 1 Report. Hennef: Deutsche Vereinigung für Wasserwirtschaft, Abwasser und Abfall. German.
- BDEW , 2011. Trinkwasserverwendung im Haushalt Drinkwater use at household level. Bundesverband der Energie- und Wasserwirtschaft e. V. Berlin, German
- Cai, T. , Park, S.Y. , and Li, Y. , 2013. Nutrient recovery from wastewater streams by microalgae. Status and prospects. Renewable and Sustainable Energy Reviews , 19, 360–369.10.1016/j.rser.2012.11.030
- Cornel, P. and Schaum, C. , 2009. Phosphorus recovery from wastewater: needs, technologies and costs. Water Science & Technology , 59 (6), 1069–1076.10.2166/wst.2009.045
- Dichtl, N. and Schmelz, K.-G. , 2015. Verfahrenstechniken zur Behandlung von Klärschlamm Sewage sludge treatment processes. In: K.-H. Rosenwinkel , et al. , eds. Anaerobtechnik. Abwasser-, Schlamm- und Reststoffbehandlung, Biogasgewinnung . Berlin, Heidelberg: Springer Berlin Heidelberg, 112–274. German.
- Driver, J. , Lijmbach, D. , and Steen, I. , 2010. Why recover phosphorus for recycling, and how? Environmental technology , 20 (7), 651–662.
- DWA , 2014a. Biologische Stabilisierung von Klärschlamm Biological stabilisation of sewage sludge. 2014th ed. Hennef: DWA. German.
- DWA , 2014b. Grundsätze für die Planung und Implementierung Neuartiger Sanitärsysteme (NASS) .Principles for the planning and implementation of new alternative sanitation systems. 2014th ed. Hennef (Sieg): Deutsche Vereinigung für Wasserwirtschaft Abwasser und Abfall e. V. German.
- DWA , 2015. Gasdurchflussmessungen auf Abwasserbehandlungsanlagen Gas flow measurements in wastewater treatment plants. 2015th ed. Hennef (Sieg): Dt. Vereinigung für Wasserwirtschaft Abwasser und Abfall. German.
- de Graaff, M.S. , et al. , 2010. Anaerobic treatment of concentrated black water in a UASB reactor at a short HRT. Water , 2 (1), 101–119.10.3390/w2010101
- van der Hoek, J.P. , Struker, A. , and de Danschutter, J. , 2015. Amsterdam as a sustainable European metropolis. Integration of water, energy and material flows. Urban Water Journal , 14 (1), 61–68.
- Kaufmann Alves, I. , 2013. A mathematical approach to find long-term strategies for the implementation of resource-orientated sanitation. Water Science and Technology : A Journal of the International Association on Water Pollution Research , 67 (7), 1442–1454.10.2166/wst.2013.691
- Kelessidis, A. and Stasinakis, A.S. , 2012. Comparative study of the methods used for treatment and final disposal of sewage sludge in European countries. Waste Management ( New York, N.Y.), 32 (6), 1186–1195.10.1016/j.wasman.2012.01.012
- Kjerstadius, H. , Haghighatafshar, S. , and Davidsson, Å. , 2015. Potential for nutrient recovery and biogas production from blackwater, food waste and greywater in urban source control systems. Environmental Technology , 36 (13), 1707–1720.10.1080/09593330.2015.1007089
- Kujawa-Roeleveld, K. , Elmitwalli, T. , and Zeeman, G. , 2006. Enhanced primary treatment of concentrated black water and kitchen residues within DESAR concept using two types of anaerobic digesters. Water Science and Technology , 53 (9), 159–168.10.2166/wst.2006.265
- Lackner, S. , et al. , 2014. Full-scale partial nitritation/anammox experiences – An application survey. Water Research , 55, 292–303.10.1016/j.watres.2014.02.032
- Meyer, C. , Preyl, V. , and Steinmetz, H. , 2015. High quality MAP production from digested sewage sludge. Proceedings of the IWA Specialist Conference on Nutrient Removal and Recovery: moving innovation into practice, 18–21 May 2015 Gdańsk, Poland.
- Muga, H.E. and Mihelcic, J.R. , 2008. Sustainability of wastewater treatment technologies. Journal of Environmental Management , 88 (3), 437–447.10.1016/j.jenvman.2007.03.008
- Nowak, O. , Enderle, P. , and Varbanov, P. , 2015. Ways to optimize the energy balance of municipal wastewater systems: Lessons learned from Austrian applications. Journal of Cleaner Production , 88, 125–131.10.1016/j.jclepro.2014.08.068
- Penn, R. , Hadari, M. , and Friedler, E. , 2012. Evaluation of the effects of greywater reuse on domestic wastewater quality and quantity. Urban Water Journal , 9 (3), 137–148.10.1080/1573062X.2011.652132
- Pidou, M. , et al. , (2007). Greywater recycling. Treatment options and applications. In Proceedings of the Institution of Civil Engineers - Engineering Sustainability , 160 (3), 119–131.
- Slagstad, H. and Brattebø, H. , 2013. Life cycle assessment of the water and wastewater system in Trondheim, Norway – A case study. Urban Water Journal , 11 (4), 323–334.
- Steinmetz, H. , et al. , 2015. Leitfaden Energieeffizienz auf Kläranlagen . Guideline Energy Efficiency in Wastewater Treatment Plants. Ministerium für Umwelt, Klima und Energiewirtschaft Baden-Württemberg. Stuttgart, German.
- Tchobanoglous, G. , et al. , 2014. Wastewater engineering. treatment and resource recovery . New York, NY : McGraw-Hill Education.
- UBA , 2015. Daten zur Umwelt; Umwelt, Haushalte und Konsum. DESTATIS, UBA (Umweltbundesamt) Data on the environment; Environment, households and consumption. DESTATIS, UBA (German Environmental Protection Agency). German, Berlin. Available from: http://www.umweltbundesamt.de/publikationen/daten-zur-umwelt-umwelt-haushalte-konsum-0
- Wang, S. and Peng, Y. , 2010. Natural zeolites as effective adsorbents in water and wastewater treatment. Chemical Engineering Journal , 156 (1), 11–24.10.1016/j.cej.2009.10.029
- Wendland, C. , et al. , 2007. Anaerobic digestion of blackwater from vacuum toilets and kitchen refuse in a continuous stirred tank reactor (CSTR). Water Science & Technology , 55 (7), 187–194.
- WSWU, and DWA , 2016. New alternative sanitation systems - NASS. Terminology, material flows, treatment of partial flows, utilisation . 1st ed. Kromsdorf: Bauhaus-Universitätsverlag als Imprint von VDG Weimar.
- Yusof, A.M , et al. , 2010. Kinetic and equilibrium studies of the removal of ammonium ions from aqueous solution by rice husk ash-synthesized zeolite Y and powdered and granulated forms of mordenite. Journal of Hazardous Materials , 174 (1–3), 380–385.10.1016/j.jhazmat.2009.09.063
- Zeeman, G. and Kujawa-Roeleveld, K. , 2013. Anaerobic treatment of source-separated domestic wastewater. In: T.A. Larsen , K.M. Udert , and J. Lienert , eds. Source separation and decentralization for wastewater management . London, New York: Iwa Publishing, 307–319.
- Zhang, D. , et al. , 2009. Decentralized water management: Rainwater harvesting and greywater reuse in an urban area of Beijing, China. Urban Water Journal , 6 (5), 375–385.10.1080/15730620902934827