ABSTRACT
Urban resilience emerges not only from ‘what’ is done in relation to critical infrastructure systems, but in the ‘how’ of their conception, co-creation and integration into complex socio-ecological-technical systems. For green infrastructure, where ownership and agency may be distributed amongst organisations and diverse communities, inclusiveness and appropriateness require embedding in engineering assessments of green infrastructure and resilience. Through consideration of past, present and future engineering and resilience assessments – from monetising, through greening, to humanising – this paper examines the ways in which GI may be or has already contributed to enhancing urban resilience and types of assessment and indicators that have been or could be used. We suggest that enhancing visibility of the ‘whos’ (individuals, communities) is crucial to fully diversifying assessments. We also suggest some ideas for additional indicators and assert that co-production of future indicators needs to be undertaken with appropriate professionals (e.g. social impact assessment professionals).
1. Introduction
With a global focus on resilience and cities, the term ‘urban resilience’ has emerged to frame how actors and infrastructures across all scales (individual, household, community, organisation, region) contribute to the capacity to survive, respond, recover, adapt and evolve in reaction to chronic and acute stresses and events that disrupt everyday systems and practices. Whatever the source of risk, threat, stress or shock, there is growing recognition from scholars and practitioners that embedding resilience into complex systems within urban environments is a priority (e.g. 100 Resilient Cities Citation2017; Butler et al. Citation2016; Lloyd’s Register Foundation Citation2015; Ahern, Citation2011; Staddon Citation2010). Focusing on one particular system of systems – that of the provision, management and governance of water services – enables a focus on the practicalities of embedding resilience across socio-ecological-technical (SET) systems within urban spaces. Within this SET water system, transition approaches to alternative and potentially more resilient and sustainable configurations are well documented and highlight that a shift from grey to green or at the very minimum grey-green hybrid systems is preferable (e.g. Staddon et al. Citation2017a; Dunn et al. Citation2017; Butler et al. Citation2016; de Haan et al. Citation2015, Citation2016; Farrelly and Brown Citation2011). This emphasises a shift in the balance between the technical and the ecological. In the context of the recent sustainable development goals (SDGs), which apply to both developing and developed countries and span the triple bottom line, it represents environmental, economic and social dimensions (Sachs Citation2012; UN Citation2017). It also becomes crucial to reinforce the equity principle of sustainable development, which orientates around fairness (World Commission on Environment and Development Citation1987; Waas et al. Citation2011). The social dimension is only beginning to feature in engineering assessments of infrastructure systems in some contexts (Ashley et al. Citation2013). Greater attention and effort are needed to shift the balance in resilient SET systems toward socially inclusive design and engineering processes. That is, whilst it is clients who commission engineers to build assets, engineers must take on board that the clients of those clients are people and communities, and it is these communities that ultimately use and benefit or suffer due to those assets (Staddon, Sarkozi, and Langberg Citation2017b).
Whilst still engineered, green infrastructure (GI; categorised in Hoang and Fenner Citation2016; Vogel et al. Citation2015) and nature-based solutions (NBS) (and other such terms, including those outlined in Fletcher et al. Citation2015), are inherently less centralised approaches to infrastructure provision. These approaches can offer multi-objective delivery of services that meet the needs of everyday social practices or water-using microcomponents of demand, as well as bringing additional health and well-being benefits (Zuniga-Teran et al. Citation2017; White et al. Citation2016; Alcock et al. Citation2014; Browne et al. Citation2014; Tzoulas et al. Citation2007). Yet their decentralised character presents a challenge, where ownership and agency may be distributed amongst a range of organisations and communities (Ward and Butler Citation2016; Hoang and Fenner Citation2016; Winz, Brierley, and Trowsdale Citation2011; Winz, Trowsdale, and Brierley Citation2014). Methodologies for the inclusion of broad socio-technical, institutional, governance and planning (i.e. top-down) perspectives are also well documented in the literature, including cooperative management, new institutional arrangements and planning disruptions (e.g. Schramm et al. Citation2017; Malekpour, Brown, and de Haan Citation2017; Brown and Keath Citation2008). But we know far less about the appropriate methodologies of how best to include social impact relating to individuals, households and local communities (i.e. bottom-up) (Wutich et al. Citation2017; Jepson et al. Citation2017; Winz, Brierley, and Trowsdale Citation2011). To embed community scale resilience in SET water systems to subsequently enhance urban resilience, participatory approaches and social impact assessment methodologies require embedding in engineering assessments, particularly for GI and NBS. Resilience emerges not only from ‘what’ things are done in relation to critical SET infrastructure systems, but in the ‘how’ of their conception, co-creation and integration across scales (Staddon, Sarkozi, and Langberg Citation2017b; Winz, Brierley, and Trowsdale Citation2011), which can only be recognised through the inclusion of the ‘whos’ behind the ‘how’ (Spano et al. Citation2017). Who participates, how do they participate, using what interventions and for what ultimate goal?
Without consideration of the ‘whos’, contestations regarding the socially positive effects resulting from GI emerge. Earlier research demonstrates that non-inclusive and poorly planned GI can lead to greater social inequality, with people from disadvantaged backgrounds forced to relocate, or being locked out of benefitting from improved ecosystem services. In some locations, such as Los Angeles, cultural politics has also been shown to interplay with green space use, with park or neighbourhood ethno-racial profiles being felt as unwelcoming or exclusionary to some groups who may have also experienced direct discrimination in those green spaces (e.g. Frantzeskaki et al. Citation2017; Haase et al. Citation2017; Wolch, Byrne, and Newell Citation2014; Byrne Citation2012; Abercrombie et al. Citation2008). GI can only work as an ‘inclusive’ intervention if different groups and social environments are respected, through participation in the co-production of decision-making criteria, even if these processes are more resource intensive (Haase et al. Citation2017). This requires reconfiguration of assessments that have in the past prioritised technical, economic and environmental dimensions, primarily through the use of quantitative tools such as cost-benefit analysis, life-cycle analysis, performance assessments, optimisation, ecosystem services assessment and risk assessments (Cousins et al. Citation2017; Hall et al. Citation2017; Look and Field Citation2017; Parsons et al. Citation2017; Naderpajouh et al. Citation2017).
Some resilience metrics and indices include social dimensions, including the City Resilience Index (100 Resilient Cities Citation2017), the Baseline Resilience Indicators for Communities (‘BRICS’, Cutter, Burton, and Emrich Citation2010) and methods developed under the ‘Safe and SuRe’ project (Casal-Campos et al., TBC; Sweetapple et al. Citation2017; Butler et al. Citation2016; Casal-Campos et al. Citation2015), but these do not necessarily follow through to SET water system engineering assessments. For example, Casal-Campos et al. (Citation2015) only include one ‘social impact category’ termed ‘acceptability’, which is a user-determined indicator of high/medium/low operationalised using arbitrary units of 1, 2 or 3 assigned to an intervention under a particular scenario. The UK’s Benefits of SuDS Tool (‘BeST’; SuDS refers to sustainable drainage systems) (CIRIA Citation2017) provides a structured approach to evaluating benefits. Its ‘social’ benefit categories orient around amenity, building temperatures (implied health impact), crime (implied wellbeing impact), education, health, recreation and traffic calming (implied health benefit). This is in addition to five ‘user defined benefits’, but it is suggested that both the social and user-defined categories can be represented by a monetised value (if some evidence is gathered). This suggests that to enhance GI and NBS engineering assessments with GI appropriateness and social inclusivity aspects, greater consideration of appropriate social impact assessment methods needs to be forthcoming. Thus, notwithstanding such emerging work in this area, this review paper aims to further elaborate on this gap in the state of the knowledge. Through an extensive review of past, present and consideration of future assessment styles for grey to green infrastructure engineering, we explore how this gap may begin to be addressed by suggesting an area for further research.
This review paper proceeds as follows. Firstly, we review the past domination of monetisation in grey but then green infrastructure engineering assessments. Then, we move to review the present focus on ‘greening’ coverage of environmental dimensions within both grey and green infrastructure engineering assessments. Finally, we consider the future of diversified (rather than just hybrid) engineering assessments (hybrid = two components; diversified = more than two components) by suggesting and reviewing some approaches to include social assessment. Hybridisation is important as the more diversified is an assessment, the greater the potential for reducing uncertainties in the assessment of resilience and sustainability. In turn, this may mean greater confidence for stakeholders in ensuring they go further to be inclusive of and appropriate to all aspects, as far as is currently possible. We suggest that tools such as social impact assessment have their place alongside their economic and environmental counterparts, which is essential for achieving amongst other things the SDGs. In our conclusion, we reiterate the main themes of discussion. In terms of methodological approach, this comprised a thorough review of the relevant literature and a meta-analysis of 10 extensive multiple organisation resilience engineering agenda setting scoping studies completed through The Resilience Shift coordinated by Arup funded through the Lloyd’s Register Foundation’s Resilience Engineering initiative. Through this meta-analysis, we were able to uncover the central tendencies in how the engineering community worldwide is thinking about and approaching resilience. The authors were responsible for one of the scoping studies, as well as a portfolio of case studies on GI and urban resilience, as part of the initiative.
2. The past: monetising engineering assessment
Historically, engineering tools and assessments have included a range of modelling and simulation approaches such as empirical methods, agent-based approaches, systems dynamics, economic theory, network-based or graph theory methods and optimisation. However, most mathematical or quantitative methods present a narrow field of application usually focusing on probabilities (as in risk assessment) or probabilistic approaches (Cousins et al. Citation2017; Hall et al. Citation2017; Look and Field Citation2017; Parsons et al. Citation2017; Naderpajouh et al. Citation2017). The priority of design and assessment has predominantly been to focus on the financial and economic, or monetised aspects, usually through cost-benefit analysis (CBA). For example, performance objectives and indicators are currently interpreted and assessed in design guidance documents, such as the Levee Handbook-CIRIA C731; EurOtop: The overtopping Manual; The Flood Estimation Handbook and the Risk assessment for flood and coastal defence systems for strategic planning (RASP) (Parsons et al. Citation2017). The designs produced through the application of these manuals and guides are then generally subject to CBA (Naderpajouh et al. Citation2017). This is paralleled in the field of natural resource management, where natural capital accounting has been used since the 1980s to determine whether countries are building or depleting their natural wealth, which will impact their ability to sustain their economic growth (Science for Environment Policy Citation2017). Methods within this field include payment for ecosystem services (PES), where ecosystem assets (stocks) and services (flows) are quantified in both monetary and biophysical units using tools such as INVEST (Integrated Valuation of Ecosystem Services and Tradeoffs) and RIOS (Resource Investment Optimisation System) (The Nature Conservancy Citation2018). We will return briefly to PES-style approaches in Section 3, which explores the ‘greening’ of engineering assessment.
With specific reference to GI, CBA has been used widely in practice, across the globe, for example, in the USA (NOAA Citation2015; USEPA Citation2014) and China (Liu et al. Citation2016) (a range of examples can be revealed with a simple internet search or for the UK visiting the ‘SusDrain’ website). The monetised approach of the previously mentioned BeST (CIRIA Citation2017) is a common one, even for intangible benefits relating to health, education and recreation that may not be particularly suitable for monetisation. However, there is a strong consensus that GI practices are more cost-beneficial than grey – as well as GI yielding additional benefits. For example, in the case of constructed wetlands, research suggests they are cheaper than conventional ‘end of the pipe’ systems while providing additional community benefits (Levy et al. Citation2014). Construction costs of constructed wetlands are lower than traditional grey infrastructure by about 20–30% and their implementation may lead to avoidance/delay in requiring additional grey infrastructure, along with energy savings (due to gravity-based processes) and lower staff costs. In a CBA of competing GI and grey infrastructure, Levy et al. (Citation2014) identified GI as the most effective solution.
However, regulations for constructed wetlands that mandate particular water quality goals may offset this financial benefit if wetland performance does not result in such goals being met. The prescriptive norms-based model of regulation is also harder for GI to meet as even engineered natural solutions offer stochastic not fully prescribed outcomes (Staddon et al. Citation2017a). Further to this, Liu et al.’s (Citation2016) stormwater-focused case study, located in Beijing, undertook a CBA of four interventions (green space depression, porous brick pavement, storage pond and a combination of all). The bespoke method estimated costs based on the average service life method, infrastructure-related benefits based on seven types focusing on water/stormwater reduction and utilisation, the estimate of stormwater and pollution reduction and the use of three cost-benefit indexes to compare economic feasibility. The average ratio of benefits to costs was estimated to be 1.91, with the combined intervention option yielding a ratio of 2.14. Whether this represents the real social value of the interventions to residents living in or near these developments is undetermined by the study, but we will return to this theme in Section 4, which explores ‘humanising’ engineering assessment. In the case of GI, it is not a client (e.g. a company, a municipality) who pays more or less, but rather there is potentially a shift in responsibility from a public/private entity to individual residents (depending on context). Overall, economic assessments may find GI to be cheaper, but not for the individual residents who may not have had to pay stormwater services before and therefore issues of inclusivity may arise – should it be assumed that everyone should and can share the responsibility?
More recently, CBA has been enhanced to include resilience in the form of a proposed ‘Resilience Return on Investment’. However, as with risk management techniques, this relies on probabilities of events occurring, which focuses on the identification of direct and indirect risks, an approach that is not considered to encompass resilience as resilience is not inherently probabilistic (Sweetapple et al. Citation2017). Additionally, we observe a growing consensus in the literature that resilience engineering must consider how organisations and systems function as a whole (Staddon et al. Citation2017a). There is an urgent need to develop the significant body of theory-related work on resilience into practice as engineers are beginning to determine how to apply concepts and new tools in the real world, underpinning action with evidence-based research. This broadening of application has occurred recently through a shift from specification-based to performance-based engineering (‘properties’ versus ‘performance’ (Butler et al. Citation2016) or ‘what’ and ‘how’ (Staddon et al. Citation2017a)). The latter has yet to become mainstream, possibly as some tools and approaches to engineering assessment of existing and new infrastructures require adapting for emerging agendas and priorities to focus more on a safe to fail rather than fail-safe philosophy (Sweetapple et al., TBC; Dong, Guo, and Zeng Citation2017; Sweetapple et al. Citation2017; Ahern, Citation2011). Additionally, it is recognised that there are few specific examples of where resilience engineering is integrated into project-level decision-making, due to regulation, guidance and standards being seen as restrictive. However, one positive example is Copenhagen’s Cloudburst Management Plan, which integrates these levels across the decision-making process (Cousins et al. Citation2017).
Whilst the focus to date has been monetising infrastructure assessment, applying systems and resilience thinking in practice, particularly as the transition from grey to green infrastructure has been forthcoming, brings with it an expectation that other aspects will also be included in engineering assessments. Other variations on CBA include its combination with geographical information systems (GIS) and building integrated management (BIM) systems to yield hybrid GIS-CBA and CBA-BIM approaches (Hall et al. Citation2017). Consequently, there has been a dominance of monetising infrastructure assessment, primarily as minimising cost was the main priority. However, this is changing as recognition that multiple benefits for minor additional cost can be worthwhile. Furthermore, GI has risen up the agenda and become a focus of assessment itself, necessitating consideration of consequences for the environment or ecosystems. The following section discusses approaches that have more recently been added to monetisation approaches to better embed costs and benefits to the environment or ecosystems within engineering assessments.
3. The present: greening engineering assessment
Despite available tools in the field of natural capital accounting and methods such as PES, which represent both monetisation and consequences for the environment, until recently they were not widely embedded into urban water engineering assessments, perhaps with the exception of the previously mentioned BeST. Recently this has changed and perhaps the most well known and applied addition to represent environmental aspects within urban water infrastructure engineering assessment currently is life-cycle assessment or analysis (LCA) or life-cycle impact assessment (as according to ISO 14040:2006 and 14044:2006). Traditionally used to assess environmental impacts associated with product design, manufacture and decommissioning, LCA has more recently been applied to wastewater, drinking water, stormwater and integrated urban water systems (Byrne et al. Citation2017) and to compare decentralised and centralised water systems for potable water production from harvested rainwater (Yan et al. Citation2017).
GI has also featured in such assessments, including in comparisons with and as hybrid solutions alongside conventional systems (such as sewers and combined sewer overflows (CSOs), in the case of waste and stormwater) (Spatari, Yu, and Montalto Citation2011; de Sousa, Montalto, and Spatari Citation2012). For example, Spatari, Yu, and Montalto (Citation2011) conducted a life-cycle energy consumption and greenhouse gas emissions analysis between conventional street design and GI, street trees, and permeable surfaces. A slow payback time was explained through GI reducing runoff and energy use, but potentially consuming more embodied energy. Similarly, Jeong et al. (Citation2016) examined low impact development (LID) interventions such as bioretention areas and rainwater harvesting within a hybrid green-grey system across multiple residential zones. The LCA estimated a higher impact from the hybrid system than from the conventional system where population densities were low; an effect not seen at higher densities. A similar ecological-economic technique trialled on constructed wetland and conventional wastewater treatment systems in Beijing, emergy analysis, used the ratio of purchased/free, local/imported (both excluding wastewater) and the ratio of electricity emergy used to characterise three kinds of wastewater treatment systems in terms of self-sufficiency, indigenousness and environmental effect, respectively (for bespoke system parameters). Results included a ratio of purchased inputs to free inputs for constructed wetlands of 3.4 compared with substantially higher ratios for activated sludge (1450) and cyclic-activated sludge systems (1583) (Zhou et al. Citation2009).
Moving from LCA-based methods to those that consider a wider range of benefits, we can refer back to the BeST (CIRIA Citation2017). With regard to ecosystem services, this considers supporting, provisioning, regulating and cultural services provided by GI interventions such as SuDS across the social-financial-environmental triple bottom line. Ecologically focused benefit categories that also focus on services for people include air quality, biodiversity and ecology, carbon reduction and sequestration, groundwater recharge and water quality. BeST has been applied to a range of case studies in the UK, such as in the Yorkshire Water area for conventional drainage and SuDS approaches (four combinations), respectively, to prevent CSOs. Conventional approaches did not cover cultural services such as recreation or amenity, regulating services such as flood or carbon management, provisioning services such as treating wastewater or supporting services such as biodiversity/ecology enhancement. The resulting CBA revealed that publically-run SuDS represented the most positive balance between costs and benefits.
Some of the current difficulties around GI implementation and specification within engineering design, particularly for England and Wales, stem from a lack of guidance and standards. However, the recent release of the Building with Nature Benchmark for GI (Sinnett, Smith, and Burgess Citation2015; Gloucester Wildlife Trust and UWE, Bristol, Sinnett et al. Citation2017) could help resolve such issues as it covers themes of wildlife, water and wellbeing (in three levels of achievement – candidate, achieved and excellent). The benchmark highlights that GI needs to respond to the local context and that currently the quality and appropriateness of GI decline through the planning process, with a corresponding lack of enforcement on delivery quality (Sinnett et al. Citation2017, Citation2018). As it provides a suite of 24 flexible standards for application across spatial scales, it would be relatively straightforward to embed the benchmark in engineering assessment. This could be an exciting new stage in its development, which is currently at the testing phase in ‘front runner’ urban developments in the UK to ensure that well-thought-out urban developments with GI would be assessed as qualifying for the benchmark and those that are not, would not. However, a limitation identified was whether applicants had submitted adequate information on the role of GI in reducing health inequalities and overcoming GI access barriers for vulnerable groups (Sinnett et al. Citation2018). In addition, benchmarking and certification could present a challenge if they require payment of a fee, which could be off-putting to urban developers (Parsons et al. Citation2010) or exclusionary to poor neighbourhoods (where residents might have to directly fund retrofit GI), whereas wealthy neighbourhoods might already have the resources needed. There could also be eligibility differences between these neighbourhoods in applying for support or co-funding, which could raise inclusivity issues (Staddon et al. Citation2017a).
Between the case studies identified for the agenda-setting scoping study conducted by the authors (Staddon et al. Citation2017a) and the work undertaken by Sinnett et al. (Citation2018), we can identify examples of what ‘good ecologically focused’ and benchmark GI might look like, with the later showing that high-quality GI can be achieved outside that envisaged only for exemplary urban developments. We took ‘good ecologically focused’ GI to mean consideration of performance and sensitivity in relation to the surrounding natural environment, which is an aspect of context-appropriate GI. This was demonstrated particularly well in two case studies from South Africa, one fully implemented and one at an experimental scale:
Constructed wetlands in Krueger National Park (KNP) (Staddon et al. Citation2017a) – with a permanent staff population of 2200 and a transient annual tourist population of 1.5 M, nature-based wastewater treatment infrastructure is both ecologically sympathetic and compliant with Department of Water and Sanitation regulations for the release of treated water back into aquatic systems and for human consumption. Ponds combined with sub-surface flow constructed wetlands ensure that 365 megalitres are treated and returned each year through systems that echo the surrounding environment and also aim to improve biodiversity. Aiming to achieve the ‘Green Drop Certification’, KNP is working with a range of key performance indicators in the context of a variable natural and human environment, where wildlife and seasonal factors (elephants, floods), and human factors (illegal sewage discharge, landfill leachate) require innovative and cost-effective interventions;
Floating treatment wetlands in Johannesburg (Staddon et al. Citation2017a) – experimental floating treatment wetlands with custom-designed plant mixes reflecting the local area were evaluated over a two-year duration at two city sites. Results showed that water quality (metal and non-metal removal) and biodiversity were enhanced. Additional benefits were also identified in relation to financial and employment opportunities in local low-income communities, demonstrating social inclusiveness.
In relation to benchmark-qualifying GI in urban development at the outline planning stage, these were documented as representing (Sinnett et al. Citation2018):
Local, ecological and heritage designations, broad ecological networks, landscape character, flood plain and surface waters;
Connections between and contributions across policy and approach;
How the GI would contribute to climate change adaptation, as well as mitigation for ecological networks, air, soil and water quality and water management;
Consideration of long-term maintenance and management (usually provided at a later stage in the planning process), including funding and governance of these aspects;
Comprehension of the meaning of community participation in the decision-making process, implementation and long-term ownership.
This final point highlights that whilst the discussion in this section shows momentum has gathered in considering the ecological aspects of GI and its incorporation in engineering assessment (‘greening’), which will continue to enable environmental impacts and consequences of infrastructure provision to be included, the more people-focused aspects of GI are only now beginning to become visible. Whilst this is an encouraging step towards including multi-system resilience indices and metrics to embed sustainable development within such assessments, greater depth is required when considering representation of human and social perspectives, which we explore in the following section.
4. The future? Humanising engineering assessment
In the previous two sections, we have focused on how engineering and resilience assessments to date have incorporated primarily economic and environmental approaches, through methods such as CBA, PES and LCA. To consider the third pillar of sustainable development and more fully engage with the SDGs, our attention now turns to the representation of the social (non-individual) and human (individual) within such assessments. Rohilla, Jainer, and Matto (Citation2017) assert that the most significant social benefits that appropriate, inclusive, accessible GI can provide cover the three areas of enhanced levels of physical activity and health; promotion of psychological health and mental well-being; and facilitation of social interaction, inclusion and community cohesion. Many agenda setting scoping studies referred to human dimensions of resilience and engineering assessment, acknowledging that stakeholders, organisational and social dimensions should be included (Cousins et al. Citation2017; Hickford et al. Citation2017; Kupers and Foden Citation2017; Look and Field Citation2017; Naderpajouh et al. Citation2017; Neef et al. Citation2017; Owen et al. Citation2017). However, they were generally not explicit in their coverage of how this should be undertaken and represented in engineering assessment.
Few studies offered any depth or articulated tools or methods for incorporating social dimensions into engineering and resilience assessments. Rather, we saw more technocratic representations and framings of ‘human factors’ (in the context of aviation and maritime transport engineering; Owen et al. Citation2017), asserting these factors should be considered to understand interactions between people and their surroundings. This was to optimise well-being and system performance through human adaptive capacities (though recognising human limits), but in a more user-centred rather than participatory sense (understanding versus co-production). Others assert that institutional aspects (human-made interactions, formal and informal structures, practices, rules, and norms (Schramm et al. Citation2017)) are omitted from most resilience heuristics, recommending more integrated approaches (following Cutter, Burton, and Emrich Citation2010), but failing to articulate exactly how to embed such social aspects into engineering assessment. Finally, we also find scholars highlighting the importance of integrated systems approaches (including social systems), with resilience thinking and engineering education as being core to working intelligently and creatively across disciplines, but not seeing the engineer as a technical expert in all areas (Parsons et al. Citation2017). In light of these findings and reflecting on past and present approaches to engineering assessment, we suggest that urban water engineers and managers have attempted to become comprehensive experts in all areas related to urban water infrastructure assessment. Yet, Vanclay (Citation2002) warns against this type of approach highlighting that training in the social sciences is required if even considering the construction of a checklist of impacts, due to a required understanding of social change processes that influence social impacts at any given time – a point we will return to later when discussing the inclusion of social impact assessment practitioners (section 4.2).
If indicators are to more coherently include human and social dimensions, the next logical question to ask might be: will there be a measurable difference in the quality of life in the community (vulnerable or otherwise) as a result of the proposed intervention? To enable exploration of this question, we need to engender an understanding within the engineering community of the types of approaches that could be used to further diversify assessments and enhance capacity in this area. This is perhaps most important in relation to the rise of GI implementation, as concerns over appropriateness and inclusiveness have already been raised where inappropriately planned GI has forced people from disadvantaged backgrounds to relocate or locked-out the benefits to them of improved ecosystem services (e.g. Frantzeskaki et al. Citation2017; Haase et al. Citation2017; Wolch, Byrne, and Newell Citation2014; Byrne Citation2012; Abercrombie et al. Citation2008). Next, we present some potential approaches that could be used to begin to build capacity in relation to social assessment and thinking beyond CBA/LCA to enable the engineering community to consider broadening coverage of human and social dimensions such as appropriateness and inclusivity.
4.1. Defining social appropriateness and inclusivity
Socially appropriate and resilient GI could be taken to mean infrastructure that is sensitive to and adaptable within its social as well as natural context (i.e. shares and complements characteristics within its local setting) and is tailored to local needs and capacities (Kupers and Foden Citation2017; Spano et al. Citation2017; Staddon et al. Citation2017a). Similarly, inclusivity ensures all socio-economic, minority, psychological and potentially disadvantaged groups (people with disabilities, people without car access, young people, homeless people, those who may be discriminated against on the basis of race, gender, nationality, religion, age, sexual orientation and so on) are represented in a public deliberation process where GI is being considered within urban development (Spano et al. Citation2017; Staddon et al. Citation2017a). If GI is part of an urban resilience strategy, critical assessment of potential impacts on well-being and social equality must apply to any planned intervention. This inherently requires consideration of the role of institutions and institutional arrangements alongside physical infrastructures and how well people cope with resultant challenges (Naderpajouh et al. Citation2017). Cutter, Burton, and Emrich (Citation2010) emphasise complementary quantitative and qualitative indicators and analysis are needed and suggest the previously mentioned BRIC indicators in five categories of social resilience, economic resilience, institutional resilience, infrastructure resilience and community capacity. The BRIC assessment is regional, with county-level resolution and spatially presented results. They also highlight composite indicators are tools to facilitate public communication, raising awareness for data-driven decision-making and trend analysis associated with policymaking based on a range of issues, although there is also inability to formalise some value systems into composite indicators (Nardo et al. Citation2005). The City Resilience Framework is similar in that it identifies 12 indicators to differentiate a resilient from non-resilient city, though how such indicators are aligned with or represent social impacts of engineered systems and how they can be down-scaled into engineering assessments is still uncertain (Arup & Rockefeller Foundation Citation2015). To explore this point further, we consider some non-engineering approaches to the assessment of value and impact analysis.
4.2. Social impact assessment
In the context of Comparative Value Assessment (CVA), metrics can orientate across utility (usefulness, purpose, benefit), quality (requirements, reliability, effectiveness), time (productivity, efficiency) and social (reputation, culture, integrity, meaning, social good), where the aim is to protect these values by understanding how they are created, where they are situated and how they might be vulnerable to external threats. Infrastructure-related value items, therefore, require defining, mapping and assessing, alongside prioritisation of needs and development of adaptive capacity. Look and Field (Citation2017) outline a clear methodology to do this for general infrastructure systems, but it is not specific to water management or GI projects. In addition, we can consider other methodologies that fit under the broad banner of social impact assessment (SIA), which is in a general sense the process of identifying the future consequences of a current or proposed intervention with respect to individuals, organisations and social macro-systems (Becker Citation2001; Mahmoudi et al. Citation2013). SIA (which can also include the terms analysis and measurement) can be categorised into three different logic models and assessment approaches, defined in (Nicholls Citation2015; Karami et al. Citation2017; Aledo-Tur and Dominguez-Gomez Citation2017). Distinction is made between a (strongly numerical) positivist (technocratic) epistemology and constructivist models that focus on socially constructed realities across multiple value systems, rather than empirically constructed realities with a primarily Western value system (Aledo-Tur and Dominguez-Gomez Citation2017).
Table 1. Different logic models for social impact assessment*.
Some approaches are evolving. Social Return on Investment (SROI), for example, originated as a positivist approach, but due to a realisation that more accurate numerical models of SIA were not forthcoming, it morphed into a set of principles that include power relations and materiality judgements alongside CBA in a consultative process to assess blended value (Esteves, Franks, and Vanclay Citation2012; Nicholls Citation2015). Similar approaches include the Balanced Scorecards/dashboards used in the corporate domain. Interpretive methods encapsulate key social impacts in complex or integrative contexts by welcoming collaborative learning, subjectivity and dialogue, which contribute to vocalising the beneficiary (‘user’) in the assessment. A critical question to ask when using these approaches, however, is who is best placed to generate or elucidate the most accurate social impact data? This could include ‘users’ (external stakeholders) and ‘producers’ (organisations requiring external input for an intended purpose) and will usually require trust amongst them (Nicholls Citation2015). Another critical question orients around the familiarity of a challenge or situation. Nicholls (Citation2015) illustrated this ‘uncertainty’ dimension alongside the trust dimension and mapped different SIA methods onto these spectra (). In the top right hand corner, ‘developmental evaluation’ represents contexts where no current SIA may exist and therefore attention is required to develop a new approach. This is arguably the current situation for inclusivity and appropriateness and human and social impact of GI SIA and indeed integrated SET GI assessment. It is necessary to go deeper into the concept of participation, because it is one of the variables that better illustrates the epistemological differences. In addition, understanding participation is essential to correctly co-design objectives, methodologies and fieldwork strategies. Participatory approaches, co-production and the inclusion of different knowledge (expert, lay, local) provide a space to focus on and rebalance power dynamics. This emphasises the value of all knowledge, not just those of a privileged, scientific or generalizable nature, but also those that are narrative, emotive or local (McEwen and Jones Citation2012). Exploring this further is beyond the context of this paper, but consideration of SIA practices in engineering assessments would begin to widen awareness of how crucial such participatory approaches are to increasing inclusivity and enhancing urban resilience.
Figure 1. Mapping Social Impact Assessment methods onto uncertainty and trust spectra*.
*modified from Nicholls (Citation2015).
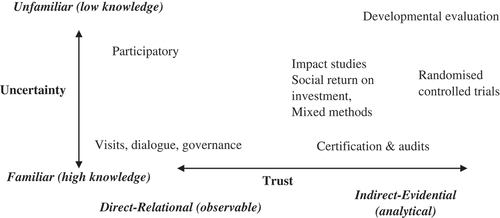
Whilst the CIRIA BeST was created for use in developmental evaluation (and includes ‘user defined’ indicators), the ‘social’ impact indicators it covers are limited, potentially as mainly producers rather than users were consulted in its creation (how many householders living with or in close proximity to GI were consulted for example). As previously mentioned, this is likely due to the nature of the professions of those who designed it and although co-produced with other experts, may have lacked participatory deliberative processes with local communities to truly address power relations and elucidate important indicators for inclusion (Vanclay, Citation2002). Following the principles of inclusivity and appropriateness requires participatory dialogue through a process that engages groups truly representative of the wider population, with that process not dominated by ‘experts’, participants with strong views or that disproportionately come from socio-economically advantaged backgrounds in order for power relations to be explored. If not carefully planned, such processes risk replicating inequalities and resource differentials that characterise wider society (Staddon et al. Citation2017a). Two 100 Resilient Cities GI inclusive examples are located in Bristol and New York, where the ‘Green and Black’ initiative includes and empowers young Black and Minority Ethnic (BAME) communities on environmental issues and environmental stewards are encouraged through school programmes, respectively (Staddon et al. Citation2017a).
‘Good’ SIA practice is participatory, provisions for those directly impacted, increases understanding of the capacity to respond to change (i.e. resilience) and avoids negative whilst enhancing positive impacts for vulnerable and disadvantaged people. We should, of course, acknowledge that vulnerability and resilience are interconnected, with vulnerability focusing on sensitivity and resilience focusing on adaptation, with literatures on social vulnerability highlighting differentiated capacities for coping with change in relation to threats or hazards (Brown Citation2015; Coirolo and Rahman Citation2014). In a socio-ecological context, vulnerability places a population at the centre of socio-environmental problems, whereas resilience may not. In a SET context, with the added complexity of infrastructural influences on people’s vulnerability, differentiated capacities for coping and adapting can lead to an overemphasis on the vulnerable as ‘victims’. In this context, the status of ‘victim’ may confer a widely accepted moral authority, which may or may not be appropriate depending on motivations for change. For example, institutional bias towards engineered measures rather than behavioural approaches may be legitimised and reinforced by decision-makers’ contact with the most vocal advocates of such measures (Harries and Penning-Rowsell Citation2011).
This does highlight, however, the political aspects of both vulnerability and resilience where power differentials play out, which are of importance in promoting social justice, fairness, equality, inclusivity and appropriateness (Huynh and Stringer Citation2018). The engineering approach currently focuses largely on functional social justice, undifferentiating reasons for vulnerability (e.g. those who chose to live in high-risk areas versus those that do not), falling short of incorporating equality and environmental sustainability and ultimately social justice (Harries and Penning-Rowsell Citation2011). Focusing on a broader interpretation of social justice within a resilience-focused and vulnerability-informed framework could help facilitate a more positive and balanced people-centred approach to resilience in engineering assessment than that which currently exists.
There is a vast literature on citizen science, participatory approaches and public engagement methodologies that can better inform GI engineering assessment processes (e.g. National Coordinating Centre for Public EngagementFootnote1 website, Sense About ScienceFootnote2 website and Waterwise’s recent Behaviour Change HandbookFootnote3). Managing the social impacts associated with planned GI interventions is key and the SIA profession can be better represented by including SIA practitionersFootnote4 from the concept stage of an urban development considering (new or retrofit) GI implementation (Esteves, Franks, and Vanclay Citation2012). To make SIA more explicit in projects, including those focusing on drought and flood impacts, Mahmoudi et al. (Citation2013) produced a framework to combine SIA and risk assessment yielding a risk and social impact assessment (RSIA). They also discuss other possible hybrids including SIA and environmental impact assessment and SIA and health impact assessment (the mental health and well-being aspects of which have been examined in relation to rainwater harvesting, SuDS and GI in the UK context by Fewtrell and Kay (Citation2008)). Further to this, they emphasise the inclusion of social norms, values and beliefs that may be real or perceived at scales ranging from personal through familial to community. They also categorise high and low impacts into intended positive, intended negative, unintended positive and unintended negative and emphasise that they should be identified across time (before, during, after) not just in a snapshot moment. Finally, they draw attention to SIA being the management of an impact or consequence, not just its identification.
A further study by Karami et al. (Citation2017) on systems dynamics (SD) and SIA, asserts that SIAs would be more effective if utilised in project design processes, suggesting that retrospective SIAs and social simulations could inform predictive assessments. They use an integrated SIA-SD approach highlighting SD assists in combining multiple datasets, understanding dynamic complexity, policy resistance/negative feedback, identifying recurring and/or long-term social problems and focusing on the problem rather than the system. Using floodwater spreading case study in Iran to incorporate quantitative and qualitative data, they present a causal comparative SIA-SD model of villages with and without the floodwater spreading scheme. The SIA consisted of six criteria including perceived well-being, social capital, quality of life, social structure development, rural and agricultural economic conditions and conservation of community resources, all with a varying range of items included within each criteria category (given in full in Karami et al. Citation2017). Referring to Ahmadvand and Karami (Citation2009) and through that paper to Simonovic (Citation1999) revealed that although criteria were both quantitative and qualitative, their derivation was still through expert recommendation after conducting interviews with residents, rather than a fully iterative participatory process and were not particularly representative of socio-cultural or cultural political criteria or inclusivity or appropriateness. However, through the SIA-SD model, they produced a range of scores across the six criteria indicating that the floodwater spreading project had yielded benefits to the included villages.
SIA is not the only approach that may be useful in catalysing hybridisation (inclusion of more than two components) of GI engineering assessment. Methodologies from across the disciplines could be examined for their applicability to the challenge of embedding appropriateness and inclusivity, for example, harm-benefit analysis.
4.3. Harm-benefit analysis
The field of harm-benefit analysis (HBA), which is primarily used in the area of animal experimentation, assesses whether (usually medical) interventions or procedures cause suffering or severe suffering to the animals involved and if the reasonably foreseeable benefits outweigh the welfare costs (‘harms’) (ASC, Citation2017). Recognised as utilitarian and quantitative, HBA, like engineering assessment, has been subject to recent review to consider whether public engagement should be more explicitly embedded in the process and how should benefits accrued over the long term e judged. One of the recommendations of the ASC (Citation2017) review was enhanced dialogue on societal views regarding certain types of harm, which could be seen as parallel to some of the concerns raised around some of the negative aspects of GI in developments.
Terminology used in this field includes contingent harms (those that are inherent and inescapable e.g. as the result of not being able to express natural behaviours), cumulative effects and severity (so not just making a snapshot assessment), project-related harms (caused by interventions) and a severity limit (highest level of pain, distress or lasting harm that may be experienced by an animal undergoing an authorised procedure). In the context of cumulative suffering, it is customary to give precedence to identifying and reducing negative over positive effects. Perhaps inclusivity and appropriateness-related costs should be identified before such benefits in the assessment of GI in urban developments? Innovative HBA indicators and methods are being developed to examine long-term experiences, so this field could prove useful to explore in relation to humanising engineering and resilience assessments over the lifetime of an urban development. A full review of the methods used within HBA is beyond the scope of this paper, but we have contributed to starting the conversation regarding incorporation of unconventional indicators and approaches within engineering and resilience assessment.
To further consider the role of SIA and HBA, in the following section we provide some initial suggestions on how to embed social appropriateness and inclusivity in engineering assessments.
4.4. Embedding social appropriateness and inclusivity in engineering assessment
Informed by the SIA and HBA literatures and in considering the other literatures presented within this paper (Zuniga-Teran et al. Citation2017; Wutich et al. Citation2017; Sinnett et al. Citation2017; Karami et al. Citation2017; Winz, Trowsdale, and Brierley Citation2014; Alcock et al. Citation2014; Esteves, Franks, and Vanclay Citation2012; Tzoulas et al. Citation2007), the authors have crafted a set of existing and potential future inclusivity and appropriateness indicators in . Existing indicators are those that are already incorporated, future ones are those that could be added subject to development with appropriate participatory processes and that consider the many properties of resilience (using the widest definition represented across the literatures examined herein). This is not intended to serve as a comprehensive list but rather as an initial set of initiatives to inform the debate. They are designed to stimulate dialogue and engage readers in the kind of impact categories that are currently missing from engineering and resilience assessments and that may need fuller exploration through participatory processes. A fully inclusive deliberative process should be followed, employing the skills of SIA/HBA professionals in each urban development considering the implementation of GI, in order for appropriate and inclusive assessment indicators to be co-defined and then assessed in each specific context.
Table 2. Existing and potential future social, inclusivity and appropriateness impact indicators for engineering and resilience assessment.
However, to provide some examples of where inclusivity and appropriateness have been apparent, we reflect on their representation in GI-focused urban development case studies from the previously discussed agenda setting scoping studies. Whilst not necessarily ranking high or low in relation to any particular indicator suggested or discussed herein, they provide general insight on those aspects that could be explored through participatory processes. For example, Tucson’s Sweetwater Wetlands, which treat wastewater for reuse in golf courses, parks and school fields, reduce potable water use as part of a reclaimed water system in the city. Taking a harm-avoidance approach, Tucson Water implemented a mosquito abatement program to reduce the risk of vector-borne disease transmission-offsetting amenity, recreation and educational benefits (though some of the actions such as applying bacterial larvicide to ponds and controlled burns of mosquito habitat may not be the most environmentally beneficial). This highlights appropriateness, where GI has been considered in relation to the surrounding environment and some of the potential human or social impacts that may result from implementing the GI intervention. Some of the suggested potential indicators from that could be of relevance here include (i) Disruption (to daily living) and (ii) Fear reduction (of being bitten and consequent physical and mental health impacts).
In relation to inclusion, the Avalon Green stormwater alleys project in Los Angeles, California, demonstrated exemplary continuous participatory engagement activities with communities in the neighbourhoods adjacent to the alleys, which began six years before any implementation to build trust and balance power differentials. The installation of community-designed murals alongside infiltration trenches and wells ensured that sense of place and cultural identity were embedded in the GI project – representing suggested potential indicators (i) Autonomy, (ii) Connection and (iii) Culture, from . It is hoped the project will reduce crime and retain a sense of community, ultimately resulting in a safer neighbourhood (no data as yet available).
Finally, in Mendoza, Argentina, the cultural and heritage legacy left by the Huarpes (original inhabitants of the river basin) in the form of an irrigation network that had been repurposed as a stormwater network (which consequently became overwhelmed), is being re-shaped using GI to return it to its original purpose and avoid geographies of environmental injustice. Additionally, the current stormwater system is inadequate and a call has been made for consensual regulation to avoid social fragmentation, loss of collective identity and to address geographical inequity, as runoff currently flows to the lower, more socially vulnerable or less resilient sectors of the city. This example highlights the suggested potential indicators from of (i) Aspirations, (ii) Culture, (iii) Equity and (iv) Integrity.
5. Conclusion
In this paper, we chart the evolution of engineering and resilience assessments for complex urban water systems. Beginning with a review of the past focus on grey infrastructure and monetising (e.g. cost-benefit analysis), to the present focus on green infrastructure and greening (e.g. life-cycle analysis, payment for ecosystem services) we suggest a potential future focus should be on grey-green infrastructure and humanising (e.g. social impact assessment, harm-benefit analysis).
We consider and define the concepts of inclusivity and appropriateness and their value in shifting towards fairer and more socially just GI implementation. This shift should enable embedding of all three pillars of sustainability, leading to full diversification (inclusion of more than two components) rather than just hybridisation (inclusion of two components) of engineering and resilience assessments. In a complex socio-ecological-technical system, such as that of water management and governance, each of these pillars is crucial – if one sub-system fails, the whole system is open to failure and thus resilience reduced. Consequently, inclusivity to represent all sectors of society is vital for social sub-system. Appropriate GI is also key for long-term SET system function, without which capacities for adapting, coping and learning may not be effective.
In considering past and present approaches, we have highlighted that omitting complex social dimensions from assessment can lead to reduced appropriateness and inclusivity in the design, provision, management and governance of water services. This is particularly the case in relation to stormwater management, which has been a focus of GI implementation to date. The embedding of humanising dimensions within engineering assessments enables the ‘whos’ to be more explicitly considered in the co-production of GI interventions. In turn, this could improve appropriateness and inclusivity, subsequently increasing capacities that more comprehensively enhance urban resilience. Embedding the ‘whos’ in such assessments via participatory and deliberative processes, therefore, becomes a critical aspiration for the future. Crucial for the success of this aspiration is for engineers to engage social impact assessment professionals in their GI design and engineering processes.
The social dimension is only beginning to feature in engineering assessment and more explicit focus is required in this area. Through the literature and case studies covered we have synthesised and suggested some potential indicators that could be further explored to enhance capacity, such as adaptability, aspiration, connection (emotional as well as physical), culture, equity, integrity, self-image and stability. Throughout the paper, we also provide international examples of GI projects that represent each stage of engineering assessment, with a view to demonstrating how a shift to a more socially appropriate and inclusive GI philosophy is being catalysed across the globe. The next step is to bring together a range of professions to more fully develop SIA indicators relating to inclusivity and appropriateness and see how they operate in the real world of GI for urban resilience.
Acknowledgements
This research was performed as part of the Resilience Shift, a Lloyd’s Register Foundation initiative delivered by Arup.Footnote5
Disclosure statement
No potential conflict of interest was reported by the authors.
Additional information
Funding
Notes
3. TO BE PUBLISHED IN LATE 2018 – reference will be added ASAP.
4. The International Association for Impact Assessment (http://www.iaia.org).
5. Lloyd’s Register Foundation is a charitable foundation, helping to protect life and property by supporting engineering-related education, public engagement and the application of research.
References
- 100 Resilient Cities. 2017. Accessed 12 June 2017. www.100resilientcities.org/resilience#/-_/
- Abercrombie, L. C., J. F. Sallis, T. L. Conway, L. D. Frank, B. E. Saelens, and J. E. Chapman. 2008. “Income and Racial Disparities in Access to Public Parks and Private Recreation Facilities.” American Journal of Preventive Medicine 34 (1): 9–15. doi:10.1016/j.amepre.2007.09.030.
- Ahern, J. 2011. “From Fail-Safe to Safe-To-Fail: Sustainability and Resilience in the New Urban World.” Landscape and Urban Planning 100 (4): 341–343. doi:10.1016/j.landurbplan.2011.02.021.
- Ahmadvand, M., and E. Karami. 2009. “A Social Impact Assessment of the Floodwater Spreading Project on the Gareth-Bygone Plain in Iran: A Causal Comparative Approach.” Environmental Impact Assessment Review 29: 126–136. doi:10.1016/j.eiar.2008.08.001.
- Alcock, I., M. P. White, B. W. Wheeler, L. E. Fleming, and M. H. Depledge. 2014. “Longitudinal Effects on Mental Health of Moving to Greener and Less Green Urban Areas.” Environ Sci Technol 48 (2): 1247–1255. doi:10.1021/es403688w.
- Aledo-Tur, A., and J. A. Dominguez-Gomez. 2017. “Social Impact Assessment (SIA) from a Multidimensional Paradigmatic Perspective: Challenges and Opportunities.” Journal of Environmental Management 195: 56–61. doi:10.1016/j.jenvman.2016.10.060.
- Animals in Science Committee (ASC). 2017. “Review of Harm-Benefit Analysis in the Use of Animals in Research.” Report of the Animals in Science Committee Harm-Benefit Analysis Sub-Group chaired by Professor Gail Davies, November. Accessed 19 January 2018. https://www.gov.uk/government/publications/harm-benefit-analysis-animals-in-science-committee-review
- Arup & Rockefeller Foundation. 2015. “City Resilience Framework.” Accessed 10 January 2018. https://assets.rockefellerfoundation.org/app/uploads/20160105134829/100RC-City-Resilience-Framework.pdf
- Ashley, R. M., L. Lundy, S. Ward, P. Shaffer, L. Walker, C. Morgan, A. J. Saul, T. Wong, and S. Moore. 2013. “Water-Sensitive Urban Design - Opportunities for the UK.” Proceedings of the Institution of Civil Engineers Municipal Engineer 2 (166): 65–76. doi:10.1680/muen.12.00046.
- Becker, H. A. 2001. “Social Impact Assessment.” European Journal of Operational Research 128: 311–321. doi:10.1016/S0377-2217(00)00074-6.
- Brown, K. 2015. Resilience, Development and Global Change. London: Routledge.
- Brown, R. R., and N. Keath. 2008. “Drawing on Social Theory for Transitioning to Sustainable Urban Water Management: Turning the Institutional Super-Tanker.” Australian Journal of Water Resources 12 (2): 73–83, 11. doi:10.1080/13241583.2008.11465336.
- Browne, A. L., M. Pullinger, W. Medd, and B. Anderson. 2014. “Patterns of Practice: A Reflection on the Development of Quantitative/Mixed Methodologies Capturing Everyday Life Related to Water Consumption in the UK.” International Journal of Social Research Methodology 17 (1): 27–43. doi:10.1080/13645579.2014.854012.
- Butler, D., S. Ward, C. Sweetapple, M. Astaraie-Imani, K. Diao, R. Farmani, and G. Fu. 2016. “Reliable, Resilient and Sustainable Water Management: The Safe and SuRe Approach.” Global Challenges 1 (1): 63–77. doi:10.1002/gch2.1010.
- Byrne, D. M., H. A. C. Lohman, S. M. Cook, G. M. Peters, and J. S. Guest. 2017. “Life Cycle Assessment (LCA) of Urban Water Infrastructure: Emerging Approaches to Balance Objectives and Inform Comprehensive Decision-Making.” Environmental Science: Water Research and Technology 3 (6): 1002–1014.
- Byrne, J. 2012. “When Green Is White: The Cultural Politics of Race, Nature and Social Exclusion in a Los Angeles Urban National Park.” Geoforum 43: 595–611. doi:10.1016/j.geoforum.2011.10.002.
- Casal-Campos, A., G. Fu, D. Butler, and A. Moore. 2015. “An Integrated Environmental Assessment of Green and Grey Infrastructure Strategies for Robust Decision Making.” Environmental Science and Technology 49 (14): 8307–8314. doi:10.1021/es506144f.
- CIRIA. 2017. “B£ST: Benefits of SuDS Tool.” Accessed 12 December 2017. http://www.susdrain.org/resources/best.html
- Coirolo, C., and A. Rahman. 2014. “Power and Differential Climate Change Vulnerability among Extremely Poor People in Northwest Bangladesh: Lessons for Mainstreaming.” Climate and Development 6 (4): 336–344. doi:10.1080/17565529.2014.934774.
- Cousins, A., L. Ellis, S. England, I. Gray, H. Hay, S. Johnson, and C. Wenger. 2017. “Agenda Setting Scoping Studies Summary Report.” The Resilience Shift, June 10. http://resilienceshift.org/publications/
- Cutter, S. L., C. G. Burton, and C. T. Emrich. 2010. “Disaster Resilience Indicators for Benchmarking Baseline Conditions.” Journal of Homeland Security and Emergency Management 7 (1). doi:10.2202/1547-7355.1732.
- de Haan, F. J., B. C. Rogers, N. Frantzeskaki, and R. R. Brown. 2015. “Transitions through a Lens of Urban Water.” Environmental Innovation and Societal Transitions 15: 1–10. doi:10.1016/j.eist.2014.11.005.
- de Haan, F. J., B. C. Rogers, R. R. Brown, and A. Deletic. 2016. “Many Roads to Rome: The Emergence of Pathways from Patterns of Change through Exploratory Modelling of Sustainability Transitions.” Environmental Modelling and Software 85: 279–292. doi:10.1016/j.envsoft.2016.05.019.
- de Sousa, M. R. C., F. A. Montalto, and S. Spatari. 2012. “Using Life Cycle Assessment to Evaluate Green and Grey Combined Sewer Overflow Control Strategies.” Journal of Industrial Ecology 16 (6): 901–913. doi:10.1111/j.1530-9290.2012.00534.x.
- Dong, X., H. Guo, and S. Zeng. 2017. “Enhancing Future Resilience in Urban Drainage System: Green versus Grey Infrastructure.” Water Research 124: 280–289. doi:10.1016/j.watres.2017.07.038.
- Dunn, G., R. R. Brown, J. J. Bos, and K. Bakker. 2017. “Standing on the Shoulders of Giants: Understanding Changes in Urban Water Practice through the Lens of Complexity Science.” Urban Water Journal 14 (7): 758–767. doi:10.1080/1573062X.2016.1241284.
- Esteves, A. M., D. Franks, and F. Vanclay. 2012. “Social Impact Assessment: The State of the Art.” Impact Assessment and Project Appraisal 30 (1): 34–42. doi:10.1080/14615517.2012.660356.
- Farrelly, M., and R. Brown. 2011. “Rethinking Urban Water Management: Experimentation as a Way Forward?” Global Environmental Change 21 (2): 721–732, 12. doi:10.1016/j.gloenvcha.2011.01.007.
- Fewtrell, L., and D. Kay. 2008. Health Impact Assessment for Sustainable Water Management. London: IWA Publishing.
- Fletcher, T. D., W. Shuster, W. F. Hunt, R. Ashley, D. Butler, S. Arthur, S. Trowsdale, S. Barraud, A. Semadeni-Davies, and J. L. Bertrand-Krajewski. 2015. “SUDS, LID, BMPs, WSUD and More – The Evolution and Application of Terminology Surrounding Urban Drainage.” Urban Water Journal 12 (7): 525–542. doi:10.1080/1573062X.2014.916314.
- Frantzeskaki, N., D. Haase, M. Fragkias, and T. Elmqvist. 2017. “Editorial Overview: System Dynamics and Sustainability: Urban Transitions to Sustainability and Resilience.” Current Opinion in Environmental Sustainability 22: 4–8. doi:10.1016/j.cosust.2017.05.001.
- Haase, D., S. Kabisch, A. Haase, E. Andersson, E. Banzhaf, F. Baro, M. Brenck, et al. 2017. “Greening Cities – To Be Socially Inclusive? about the Alleged Paradox of Society and Ecology in Cities.” Habitat International 64: 41–48. doi:10.1016/j.habitatint.2017.04.005.
- Hall, P. J., C. Carter, E. C. Bill, M. O’Connor, and M. C. Simpson. 2017. “Resilience Return on Investment (RROI). Agenda Setting Scoping Studies Summary Report.” The Resilience Shift, June 30. http://resilienceshift.org/publications/
- Harries, T., and E. C. Penning-Rowsell. 2011. “Victim Pressure, Institutional Inertia and Climate Change Adaptation: The Case of Flood Risk.” Global Environmental Change 21 (1): 188–197. doi:10.1016/j.gloenvcha.2010.09.002.
- Hickford, A., S. Blainey, A. Ortega Hortelano, and R. Pant. 2017. “A Review of Resilience in Interdependent Transport, Energy and Water Systems. Agenda Setting Scoping Studies Summary Report.” The Resilience Shift, February 26. http://resilienceshift.org/publications/
- Hoang, L., and R. A. Fenner. 2016. “System Interactions of Stormwater Management Using Sustainable Urban Drainage Systems and Green Infrastructure.” Urban Water Journal 13 (7): 739–758. doi:10.1080/1573062X.2015.1036083.
- Huynh, L. T. M., and L. C. Stringer. 2018. “Multi-Scale Assessment of Social Vulnerability to Climate Change: An Empirical Study in Coastal Vietnam.” Climate Risk Management 20: 165–180. doi:10.1016/j.crm.2018.02.003.
- Jeong, H., O. A. Broesicke, B. Drew, D. Li, and J. C. Crittenden. 2016. “Life Cycle Assessment of Low Impact Development Technologies Combined with Conventional Centralized Water Systems for the City of Atlanta, Georgia.” Frontiers of Environmental Science & Engineering 10 (6). doi:10.1007/s11783-016-0851-0.
- Jepson, W., J. Budds, L. Eichelberger, L. Harris, E. Norman, K. O’Reilly, A. Pearson, et al. 2017. “Advancing Human Capabilities for Water Security: A Relational Approach.” Water Security 1: 46–52. ISSN: . doi:10.1016/j.wasec.2017.07.001.
- Karami, S., E. Karami, L. Buys, and R. Drogemuller. 2017. “System Dynamic Simulation: A New Method in Social Impact Assessment (SIA).” Environmental Impact Assessment Review 62: 25–34. doi:10.1016/j.eiar.2016.07.009.
- Kupers, R., and M. Foden. 2017. “Learning for Resilience and Complex Systems Thinking. Agenda Setting Scoping Studies Summary Report.” The Resilience Shift, June 15. http://resilienceshift.org/publications/
- Levy, Z., R. Smardon, J. Bays, and D. Meyer. 2014. “A Point Source of a Different Color: Identifying a Gap in United States Regulatory Policy for “Green” CSO Treatment using Constructed Wetlands.” Sustainability 6 (5): 2392–2412. doi:10.3390/su6052392.
- Liu, W., W. Chen, Q. Feng, C. Peng, and P. Kang. 2016. “Cost-Benefit Analysis of Green Infrastructures on Community Stormwater Reduction and Utilisation: A Case Study of Beijing, China.” Environmental Management 58: 1015–1026. doi:10.1007/s00267-016-0765-4.
- Lloyd’s Register Foundation. 2015. “Foresight Review of Resilience Engineering: Designing for the Expected and Unexpected.” No. 2015.2. London, UK.
- Look, R., and C. Field. 2017. “Resilient Foundation through Systems Thinking. Agenda Setting Scoping Studies Summary Report.” The Resilience Shift, June 16. http://resilienceshift.org/publications/
- Mahmoudi, H., O. Renn, F. Vanclay, V. Hoffmann, and E Karami. 2013. “A Framework for Combining Social Impact Assessment and Risk Assessment.” Environmental Impact Assessment Review 43: 1–8. doi:10.1016/j.eiar.2013.05.003.
- Malekpour, S., R. R. Brown, and F. J. de Haan. 2017. “Disruptions in Strategic Infrastructure Planning – What Do They Mean for Sustainable Development?” Environment and Planning C: Politics and Space 35 (7): 1285–1303.
- McEwen, L., and O. Jones. 2012. “Building Local/Lay Flood Knowledges into Community Flood Resilience Planning after the July 2007 Floods, Gloucestershire, UK.” Hydrology Research 43 (5): 675–688. doi:10.2166/nh.2012.022.
- Naderpajouh, N., D. Yu, D. P. Aldrich, and I. Linkov. 2017. “Towards an Operational Paradigm for Engineering Resilience of Interdependent Infrastructure Systems. Agenda Setting Scoping Studies Summary Report.” The Resilience Shift, June 15. http://resilienceshift.org/publications/
- Nardo, M., M. Saisana, A. Saltelli, S. Tarantola, A. Hoffman, and E. Giovannini. 2005. “Handbook on Constructing Composite Indicators: Methodology and User Guide.” No. 2005/3. Paris: OECD Publishing
- Neef, M., H. Duijnhoven, A. Herder, and T. van Schie. 2017. “Engineering Resilient Urban Cyber Landscapes. Agenda Setting Scoping Studies Summary Report.” The Resilience Shift, June 15. http://resilienceshift.org/publications/
- Nicholls, A. 2015. “Synthetic Grid: A Critical Framework to Inform the Development of Social Innovation Metrics.” CRESSI Working Papers No. 14/2015. ISBN: 978-0-9933861-3-8. Accessed 9 January 2018. http://eureka.sbs.ox.ac.uk/5944/1/CRESSI_Working_Paper_14_D3.1_10Sept.pdf
- NOAA. 2015. “A Guide to Assessing Green Infrastructure Costs and Benefits for Flood Reduction.” Economics of Green Infrastructure series, NOAA Office for Coastal Management. www.coast.noaa.org
- Owen, D., S. Gill, H. Courtney, N. McDonald, P. Liston, and M. Carrera. 2017. “Exploring Resilience in Aviation and Maritime Transport – And How Human Factors Can Help. Agenda Setting Scoping Studies Summary Report.” The Resilience Shift, June 16. http://resilienceshift.org/publications/
- Parsons, A., J. Pearson, M. Mayfield, G. Punzo, P. Collins, G. Brighty, G. Cuomo, and S. Jeavons. 2017. “Flood Resilience: Consolidating Knowledge between and within Critical Infrastructure Sectors. Agenda Setting Scoping Studies Summary Report.” The Resilience Shift, June 15. http://resilienceshift.org/publications/
- Parsons, D., S. Goodhew, A. Fewkes, and P. De Wilde. 2010. “The Perceived Barriers to the Inclusion of Rainwater Harvesting Systems by UK House Building Companies.” Urban Water Journal 7 (4): 257–265. doi:10.1080/1573062X.2010.500331.
- Rohilla, S. K., S. Jainer, and M. Matto. 2017. Green Infrastructure: A Practitioner’s Guide. New Delhi: Centre for Science and Environment. Accessed 9 January 2018. http://cseindia.org/userfiles/Green-Infrastructure-guide.pdf
- Sachs, J. D. 2012. “From Millennium Development Goals to Sustainable Development Goals.” The Lancet 379: 2206–2211. doi:10.1016/S0140-6736(12)60685-0.
- Schramm, E., H. Kerber, J. H. Trapp, M. Zimmermann, and M. Winker. 2017. “Novel Urban Water Systems in Germany: Governance Structures to Encourage Transformation.” Urban Water Journal. doi:10.1080/1573062X.2017.1293694.
- Science for Environment Policy. 2017. Taking Stock: Progress in Natural Capital Accounting. Bristol: In-depth Report 16 produced for the European Commission, DG Environment by the Science Communication Unit, UWE. ISBN: 978-92-79-55106-2. ISSN: . doi:10.2779/304410. http://ec.europa.eu/science-environment-policy
- Simonovic, S. P. 1999. “Social Criteria for Evaluation of Flood Control Measures: Winnipeg Case Study.” Urban Water Journal 1: 167–175. doi:10.1016/S1462-0758(99)00017-5.
- Sinnett, D., G. Jerome, N. Smith, S. Burgess, and R. Mortlock. 2018. “Raising the Standard: Developing a Benchmark for Green Infrastructure.” The International Journal of Sustainable Development & Planning 13 (2): 226–236. http://eprints.uwe.ac.uk/32295
- Sinnett, D., G. Jerome, S. Burgess, N. Smith, and R. Mortlock. 2017. “Building with Nature - a New Benchmark for Green Infrastructure.” Town and Country Planning 86 (10): 427–431. ISSN: . http://eprints.uwe.ac.uk/33406
- Sinnett, D., N. Smith, and S. Burgess. 2015. Handbook on Green Infrastructure. Edward Elgar. ISBN: 9781783473991. http://eprints.uwe.ac.uk/25928
- Spano, M., F. Gentile, C. Davies, and R. Lafortezza. 2017. “The DPSIR Framework in Support of Green Infrastructure Planning: A Case Study in Southern Italy.” Land Use Policy 61: 242–250. doi:10.1016/j.landusepol.2016.10.051.
- Spatari, S., Z. Yu, and F. A. Montalto. 2011. “Life Cycle Implications of Urban Green Infrastructure.” Environmental Pollution 159 (8–9): 2174–2179. doi:10.1016/j.envpol.2011.01.015.
- Staddon, C. 2010. Managing Europe’s Water Resources: 21st Century Challenges. Farnham: Ashgate. ISBN: 0754673219.
- Staddon, C., R. Sarkozi, and S. Langberg. 2017b. “Urban Water Governance as a Function of the “Urban Hydrosocial Transition”.” In Freshwater Governance for the 21st Century, edited by E. Karar, 81–102. Springer. ISBN: 9783319433486. http://eprints.uwe.ac.uk/30383
- Staddon, C., L. De Vito, A. Zuniga-Teran, Y. Schoeman, A. Hart, and G. Booth. 2017a. “Contributions of Green Infrastructure to Enhancing Urban Resilience. Agenda Setting Scoping Studies Summary Report.” The Resilience Shift, June 30. http://resilienceshift.org/publications/
- Sweetapple, C., R. Farmani, G. Fu, and D. Butler. 2017. Towards a Comprehensive, General Resilience Assessment for Intervention Development in Water Distribution Systems. Dresden, Germany: Dresden Nexus Conference. May 17–19.
- The Nature Conservancy. 2018. “Technical Studies: Ecosystem Services Analysis.” Accessed 16 February 2018. https://www.nature.org/ourinitiatives/habitats/riverslakes/ecosystem-services-analysis.xml?redirect=https-301
- Tzoulas, K., K. Korpela, S. Venn, V. Yli-Pelkonen, A. Kaźmierczak, J. Niemela, and P. James. 2007. “Promoting Ecosystem and Human Health in Urban Areas Using Green Infrastructure: A Literature Review.” Landscape and Urban Planning 81 (3): 167–178. doi:10.1016/j.landurbplan.2007.02.001.
- UN. 2017 “Sustainable Development Goals: 17 Goals to Transform Our World.” http://www.un.org/sustainabledevelopment/sustainable-development-goals/
- USEPA. 2014. “The Economic Benefits of Green Infrastructure: A Case Study of Lancaster, PA.” EAP 800-R-14-007.
- Vanclay, F. 2002. “Conceptualising Social Impacts.” Environmental Impact Assessment Review 22: 183–211. doi:10.1016/S0195-9255(01)00105-6.
- Vogel, J. R., T. L. Moore, R. R. Coffman, S. N. Rodie, S. L. Hutchinson, K. R. McDonough, A. J. McLemore, and J. T. McMaine. 2015. “Critical Review of Technical Questions Facing Low Impact Development and Green Infrastructure: A Perspective from the Great Plains.” Water Environment Research 87 (9): 849–862. doi:10.2175/106143015X14362865226392.
- Waas, T., J. Huge, A. Verbruggen, and T. Wright. 2011. “Sustainable Development: A Bird’s Eye View.” Sustainability 3 (10): 1637–1661. doi:10.3390/su3101637.
- Ward, S., and D. Butler. 2016. “Rainwater Harvesting and Social Networks: Visualising Interactions for Niche Governance, Resilience and Sustainability.” Water Journal 8 (11): 526. doi:10.3390/w8110526.
- White, M. P., L. R. Elliott, T. Taylor, B. W. Wheeler, A. Spencer, A. Bone, M. H. Depledge, and L. E. Fleming. 2016. “Recreational Physical Activity in Natural Environments and Implications for Health: A Population Based Cross-Sectional Study in England.” Prev Med 91: 383–388. doi:10.1016/j.ypmed.2016.08.023.
- Winz, I., G. Brierley, and S. Trowsdale. 2011. “Dominant Perspectives and the Shape of Urban Stormwater Futures.” Urban Water Journal 8 (6): 337–349. doi:10.1080/1573062X.2011.617828.
- Winz, I., S. Trowsdale, and G. Brierley. 2014. “Understanding Barrier Interactions to Support the Implementation of Sustainable Urban Water Management.” Urban Water Journal 11 (6): 497–505. doi:10.1080/1573062X.2013.832777.
- Wolch, J. R., J. Byrne, and J. P. Newell. 2014. “Urban Green Space, Public Health, and Environmental Justice: The Challenge of Making Cities ‘Just Green Enough’.” Landscape and Urban Planning 125: 234–244. doi:10.1016/j.landurbplan.2014.01.017.
- World Commission on Environment and Development. 1987. Our Common Future. Oxford: Oxford University Press.
- Wutich, A., J. Budds, S. Young, and C. Staddon. 2017. “Advancing Methods for Research on Household Water Insecurity: Studying Entitlements and Capabilities, Socio-Cultural Dynamics and Political Processes, Institutions and Governance.” Water Security. ISSN: . http://eprints.uwe.ac.uk/34068
- Yan, X., S. Ward, D. Butler, and B. Daly. 2017. “Performance Assessment and Life Cycle Analysis of Potable Water Production from Harvested Rainwater by a Decentralised System.” Journal of Cleaner Production. doi:10.1016/j.jclepro.2017.11.198.
- Zhou, J. B., M. M. Jiang, B. Chen, and G. Q Chen. 2009. “Emergy Evaluations for Constructed Wetland and Conventional Wastewater Treatments.” Communications in Nonlinear Science and Numerical Simulation 14 (4): 1781–1789. doi:10.1016/j.cnsns.2007.08.010.
- Zuniga-Teran, A. A., B. J. Orr, R. H. Gimblett, N. V. Chalfoun, D. P. Guertin, and S. E. Marsh. 2017. “Neighborhood Design, Physical Activity, and Wellbeing: Applying the Walkability Model.” International Journal of Environmental Research and Public Health 14 (1): 76. doi:10.3390/ijerph14010076.