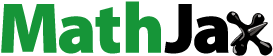
ABSTRACT
Copper is a common roofing material used in urban environments, yet it poses a threat to the ecosystem. Space requirements for stormwater treatment in urban areas are often problematic. This study investigated the treatment efficiency of a filter system containing zeolite as filter medium and treatment of copper roof runoff in field conditions. Emphasis was placed on copper and zinc treatment, while the release of sodium and aluminium was also evaluated. The filter system was monitored over a period of 16 months (7 sampling events). The filter reduced the total and dissolved copper by 52–82% and 48–85%, respectively. Although the average observed treatment efficiency of copper and zinc was high, considerable decline in filter efficiency was noticed, indicating potential saturation of the filter medium. Moreover, the copper concentrations in the outlet were still high, 350–600 μg/l, and significantly higher than the concentrations recommended by the relevant authorities.
Introduction
The continual trend towards urbanisation is leading to increased stormwater runoff and deterioration of its quality (Walsh et al. Citation2005). The most important stormwater quality parameters include total solids, total suspended solids, dissolved oxygen, metals, and PAHs (Makepeace, Smith, and Stanley Citation1995; Müller and Österlund et al. Citation2020). As pollutants, metals are of particular interest due to their toxicity. Copper is a natural element in the environment but is toxic in high doses, especially to fish and algae (Flemming and Trevors Citation1989; Kayhanian and Stransky et al. Citation2008).
Copper, alongside steel, zinc, and aluminium are the most commonly used metals for roofing and roof runoff was identified as a significant source of pollution of the receiving waterbodies (De Buyck and Van Hulle et al. Citation2021). In Stockholm, the total area of copper roofs exceeded 600,000 m2 in 1999, and there were 142 copper roofs of areas in excess of 1000 m2 (Ekstrand, Östlund, and Hansen Citation2000). When applying corrosion and runoff rates, 1200 kg of copper was estimated to be released to the environment from copper roofs alone (Ekstrand, Östlund, and Hansen Citation2000). In a literature review of pollutant concentration from roof runoff, (De Buyck and Van Hulle et al. Citation2021) found the average concentration of copper in runoff originating from copper roofs to be 2.8 mg/l. Most of the copper found in the stormwater runoff from copper roofs is dissolved (Müller and Österlund et al. Citation2019), hence stormwater treatment methods based on sedimentation processes are not efficient for copper roof runoff. Additionally, one of the limiting factors when installing a stormwater treatment facility in the urban environment is the available area (Cettner, Ashley, et al., Citation2014); therefore, there is an increased interest in analysing stormwater treatment methods that could be installed underground to reduce the area footprint. One such method are stormwater treatment systems based on filtration (Reddy, Xie, and Dastgheibi Citation2014a).
Naturally occurring zeolites such as clinoptilolite, chabazite and phillipsite, and artificial zeolite minerals are known for their cation exchange properties and have been used for removal of metal cations from municipal and industrial wastewater (Hedström Citation2001). A number of studies have addressed zeolites in the context of urban runoff treatment in both laboratory and field investigations. In single-component systems under laboratory conditions, zeolites were shown to be effective at removing lead, copper and zinc (Gray Citation2012; Reddy, Xie, and Dastgheibi Citation2014b). (Pitcher, Slade, and Ward Citation2004) investigated in a laboratory study the ability of zeolites to decrease the concentration of metals in roadway runoff, and observed that zeolites were able to substantially decrease the metal concentration, albeit increasing the pH in treated water. In a series of column experiments, (Färm Citation2002) found that mixtures of a silicate rock and zeolite were effective at reducing heavy metals in water, but that performance was significantly impacted by the hydraulic load. In a field study, (Athanasiadis, Helmreich, and Horn Citation2007) found the copper concentration to be reduced by 96% in a clinoptilolite filter system installed to treat copper roof runoff. Zeolites are also used for removal of bacteria. In a biofilter column study, (Li, McCarthy, and Deletic Citation2016) concluded that the inclusion of zeolite in traditional biofilters improved the removal of E. coli by more than 50%. Furthermore, zeolites have been suggested to present a low-cost solution for stormwater treatment due to their availability (Babel and Kurniawan Citation2003; Ziyath and Mahbub et al. Citation2011). Although zeolite filter systems have been studied previously, there is little information available about the long-term efficiency of these systems, which could be an issue given the ion-exchange properties of filter materials.
In order to understand the functioning and evaluate effectiveness of stormwater treatment systems, it is necessary to preform both the laboratory and field experiments. Various treatment systems based on filtration have been the subjects of either batch or column experiments where the capacity of systems to treat various pollutants is investigated (Reddy, Xie, and Dastgheibi Citation2014a; Behroozi and Arora et al. Citation2020; Okaikue-Woodi, Cherukumilli, and Ray Citation2020; Turco and Brunetti et al. Citation2020). However, it is impossible to fully replicate filed conditions, no matter the level of detail of a laboratory experiment. Furthermore, in a review focusing on removal of stormwater contaminants by filtration systems, it was noted that few studies examine stormwater filtration treatment at a pilot scale (Okaikue-Woodi, Cherukumilli, and Ray Citation2020).
The aim of this study, therefore, was to evaluate the efficiency of a zeolite filter system treating copper roof runoff in a field experiment. Focus was put on the treatment efficiency of the zeolite filter with respect to copper, zinc, nutrients and bacteria, the possible release of sodium and aluminium from the filter, and the change in treatment efficiency as the filter aged over a period of 16 months. Uncertainties in both the chemical analyses and the event mean concentrations were taken into account in the data analysis.
Methods
Description of the field site and treatment system
The filter system investigated () was located in Stockholm, Sweden, and treated runoff from a catchment comprising a copper roof of an area of approximately 4000 m2 (90% of which was covered with copper, 10% with glass). A small section of a park, with an area of 800 m2, also formed part of the catchment. The copper roof was fitted in 2017 as a part of a building renovation.
The roof and park runoff was conveyed initially to a collection tank by gravity. When reaching a pre-set water level in the collection tank, the water was pumped to an underground storage tank with a volume of 76.2 m3 which was connected to an influent well. From there, water was pumped upwards through the filter units once the water level in the influent well reached a critical level. The pump flow from the influent well tank through the zeolite filter units was set at a fixed value of 7 l/s, corresponding to a hydraulic load of 6.7 m3 m–2h–1. After the filter units, the treated water was then discharged by gravity pipes to a manhole, and from there to the sea. No base flow was detected in the outlet manhole when the pump was not running.
The roof runoff was treated by means of a commercial filter installation with five cylindrical filter units in parallel, each with a diameter of 1 m and a height of 0.5 m, with an opening of a diameter of 0.2 m in the middle of the cylinder. Each filter unit contained 66 kg of zeolite. The zeolite used in the filter was synthetic zeolite with a chemical formula Na2O*Al2O3*2.4SiO2*nH2O and grain size between 1 and 8 mm. (3P Technik Filtersysteme GmbH – Hydrosystem 1000 ®, 2020). Cation exchange capacity of the zeolite was 45 cmol+/kg (Helmreich and Horn Citation2010).
Filter clogging is a known phenomenon that over time reduces the performance of stormwater treatment systems that use filtration media (Kandra and McCarthy et al. Citation2014). If not maintained, clogging can limit the lifetime of treatment systems (Knowles and Dotro et al. Citation2011). In order to prevent clogging and ensure the hydraulic capacity of the filter system, filter units were backflushed on 17 April 2019.
Precipitation
Daily precipitation data was collected from a Swedish Meteorological and Hydrological Institute weather station (station number 98,210), located 2 km away from the field site, using a Geonor weighing gauge. While the filter was operational (July 2018 – March 2020), cumulative precipitation amounted to 830 mm ()
Sampling procedure
Seven sampling events took place between December 2018 and March 2020 () to assess the treatment efficiency of the zeolite filter. Sampling events did not follow specific rain events, but were intended to be carried out at regular intervals; and all the water stored in the collection and storage tank was emptied out during each sampling event.
Table 1. Number of samples taken at each sampling event and total sampling time
At each sampling event, samples were taken from both the influent well and the outlet manhole (). Five time-proportional manual samples were generally taken at both sampling points during each sampling event. Fewer samples were collected during the first two events as only a small volume of water was stored in the storage tank (). Seven inflow samples and six outflow samples were taken at the third sampling event in May 2019. The sampling procedure was standardised to five inflow and five outflow time-proportional samples during the subsequent sampling events.
The pumping time during the sampling events depended on the amount of water stored in the storage tank, and pumping continued until the water level in the influent well reached a critical minimum value.
An ISCO 2150 area velocity flow sensor was placed at the end of the pipe at the outlet manhole in order to measure the flow velocity and flow depth. There were noticeable discharge peaks from the effluent pipe during the sampling events due to the pumping system settings described above. To evaluate the water quality during these flow peaks at the outlet, additional effluent peak samples were collected in order to assess whether the treatment level was influenced by the varying flow observed in the effluent pipe ().
Influent samples were taken using a Rüttner water sampler (KC Denmark) at the influent well (). Each influent sample was poured from the sampler into a pre-washed 2000 ml plastic bottle. The effluent samples were collected manually by placing a 2000 ml sampling bottle below the outlet pipe. After each sampling event, samples were poured from the 2000 ml bottles into smaller bottles for later analysis. Before each pouring operation, the 2000 ml bottle was shaken to resuspend any sediment so as to ensure representative subsamples were taken each time. Water samples to be analysed for dissolved organic carbon (DOC) and dissolved metals were filtered through 0.45 μm filters in-situ directly after sampling. Subsamples for total dissolved metals TSS and turbidity were stored in a refrigerated room overnight and analysed the following day. Samples for total phosphorus analysis were stored in a freezer unit. Samples for analysis of bacteria were stored in bottles prepared by the laboratory, and analyses commenced within 6 hours.
E. coli and intestinal enterococci in the influent and effluent of the zeolite filter were analysed in the samples taken at sampling events in September 2019, November 2019 and March 2020. Samples for bacterial analysis were taken at the same time as the time-proportional samples for metal analysis.
Water quality analyses
Total suspended solids were analysed by filtration through glass fibre filters according to EN 872:2005 (Citation2005). pH was measured in-situ using a WTW pH3110 set, which was calibrated in-situ prior to each sampling event. Turbidity was analysed using a HACH 2100 N turbidity meter in the laboratory.
Total and dissolved metal concentrations were analysed using ICP-SFMS according to standards (ISO 11885:2007 Citation2007Citation2007) and (ISO 17294:2004 Citation2004) respectively.
E. coli was analysed using the SS 02 81 67 (Citation1996) standard, and intestinal enterococci were analysed using ISO 7899–2:2000 (Citation2000). Total and dissolved organic carbon (TOC and DOC) were analysed using the guidelines for determination of total organic carbon and dissolved organic carbon (EN 1484:1997 Citation1997).
Phosphorus concentrations were analysed according to EN 1189:1997 (Citation1997).
Statistical analyses
Welch’s t-test was used to assess whether there was a significant difference between the total and dissolved copper concentrations in the time-proportional samples and the peak flow samples. This is an appropriate t-test for samples with unequal variance and/or unequal sample size (Ruxton Citation2006).
In instances where the concentrations of total and dissolved metals were found to be below the limit of detection, half the value of the limit of detection was used in the calculation and analysis as the total number of samples where this occurred accounted for less than 15% of the total samples (US EPA Citation2006).
A linear regression model was used to determine the relationship between treatment efficiency with regard to copper and zinc and the number of accumulated filter bedload volumes treated. The rational method was used to calculate the accumulated filter bedload volumes treated by the zeolite filter at each sampling event, assuming a runoff coefficient of 0.9 (Farreny and Morales-Pinzón et al. Citation2011) and the precipitation data shown in . The number of bedload volumes treated was calculated according to the following equation:
where is the number of bedload volumes treated,
is the cumulative volume of water treated by the filter system at different sampling events and
is the filter volume.
One factor in all sampling campaigns is uncertainty. Two kinds of uncertainty were identified during the analysis; the analytical uncertainties from the laboratory analyses, and the uncertainty relating to the variability of samples taken. The laboratory uncertainty of each metal concentration analysed was determined while taking into account instrument instability, uncertainty in balances, volumetric equipment and errors in calibration standards (Joint Committee For Guides In Metrology Citation2008). The uncertainty provided by the laboratory covered a 95% confidence interval. Both uncertainties were determined and compared in order to determine the average uncertainty for total and dissolved metal concentrations for each calculated event mean concentration, and the larger one was used.
When calculating average analytical uncertainty for a sampling event, the mean concentration and uncertainties were obtained by means of equation 2.
where was the analytical uncertainty sampling event i where a total number of samples n was taken and
is the average analytical uncertainty for the sampling event i.
Results
Quality of the influent and effluent
For total and dissolved copper, the statistical Welch test showed that there was no significant difference between the peak and time-proportional samples taken as part of one sampling event, for any of the sampling events. This implied that both time-proportional and peak effluent samples could be treated the same when it came to analysing the results. Total and dissolved concentrations of copper, zinc, sodium and aluminium are presented in
The average total influent concentrations of copper ranged from 916 μg/l to 2124 μg/l, with average uncertainty of 157 μg/l. A rather high copper reduction was observed after the filter. Total copper concentrations were still high in the effluent, however, varying between 360 and 600 μg/l across sampling events. The largest proportion of total copper, in both the influent and the effluent, was in the dissolved phase, standing at 93% and 80%, respectively.
The average concentration of total zinc in the influent samples was 186–464 μg/l. Total zinc concentration in the effluent ranged from 19 to 121 μg/l, while the dissolved fraction accounted for 19 to 83 μg/l. In a similar manner to copper, most of the zinc was found in the dissolved phase, averaging 93% in both the influent and effluent.
As for copper and zinc, most of the sodium in the influent samples – 93 to 99% – was found in the dissolved phase. On average, the total sodium concentration in the effluent was four times higher than in the influent. This could be related to the ion-exchange process where copper and zinc ions are adsorbed to the zeolite surfaces and sodium is released to the water phase, as described by (Doula, Ioannou, and Dimirkou Citation2002), for instance.
In the influent, aluminium was mostly present in the particulate phase, accounting for 87–99% of the total aluminium concentration, which ranged from 30 to 50 μg/l. One exception was the sampling event in June 2019, where the particulate phase accounted for just 41%; but that was likely to be due to the method used for accounting for values below the limit of detection, as the limit of detection for the method used to determine dissolved metals was more sensitive than the limit of detection for total metals. The concentrations of total aluminium in the effluent were noticeably higher than in the influent; 138% higher on average, so 70–250 μg/l. In the effluent, the particulate phase contributed to 24–76% of total aluminium concentrations.
The phosphorus analysis showed that the concentration of phosphorus in the water was low for all the sampling events. The values in both the influent and the effluent were below the limit of detection (0.01 mg/l) during the first three sampling events. On the other occasions, the values were approximately 0.02 mg/l and there was no noticeable difference between the influent and effluent values.
Both TOC and DOC in influent and effluent were below the limit of detection of 0.5 mg/l for the first three sampling events. TOC ranged from 2.3 to 7.8 mg/l and 0.7 to 4.6 mg/l in influent and effluent, respectively, for the subsequent sampling events. The DOC values were between 2 and 4.8 mg/l for the influent and 1.4 and 4 mg/l for the effluent. The filter system was able to reduce TOC values by 16–68%.
The turbidity in the influent varied from 2.6 to 13.4 NTU and in the effluent, the turbidity ranged between 1.2 and 7.4 NTU. The turbidity reduction varied between 20% and 68% with an average of 44%.
Total suspended solids (TSS) in the influent ranged from 3.4 to 19.3 mg/l. Influent TSS was below the 2 mg/l limit of detection for the first sampling event. The values for TSS were below the limit of detection in the effluent, except for the sampling events in March and May 2019, when the effluent TSS concentrations averaged 5 mg/l and 2.2 mg/l, respectively. TSS was not measured during the last sampling event in March 2020.
While E. coli concentrations were below the limit of detection in both the influent and the effluent, a fourfold increase in the intestinal enterococci concentration in the effluent was identified during the sampling event in September 2019. In the influent, the concentration of intestinal enterococci was close to 220 cfu/100 ml; while in the effluent this concentration was almost 960 cfu/100 ml. The analyses of intestinal enterococci were below the limit of detection in both the influent and effluent in November 2019 and March 2020.
The treatment process in zeolite filters increased the pH of the water (). The average influent pH was 6.3, and the average effluent pH was 7.4. The ion-exchange process could be one reason for the pH increase in the effluent samples (Kocasoy and Şahin Citation2007), where protons are adsorbed to the positive zeolite surfaces. There was a noticeable decline in pH in the effluent during the 16-month study period (), which supports the explanation that there was a gradual reduction in the available cation exchange sites over time, also resulting in declining copper and zinc treatment over time.
Treatment efficiency regarding Copper and Zinc
The average treatment efficiencies of total copper and zinc were 66 and 72%, respectively (). Total and dissolved copper reduction amounted to 52–82% and 48–85%, respectively. For total and dissolved zinc, the values were in the ranges 50–94% and 48–94%, respectively ().
Table 2. Treatment efficiency (%) of the zeolite filter under investigation for total and dissolved copper and zinc
The influential factor in filter ageing is not the amount of time that the filter has been in operation, but the volume of the water treated. therefore presents the treatment efficiency of copper and zinc in relation to the number of filter bedload volumes treated over the 16-month evaluation period. There was a trend in the decrease of copper and zinc treatment efficiency over the period studied; more so for zinc than for copper. The coefficient of determination (R2) was 0.35 and 0.42, respectively, for copper and zinc treatment efficiency over the bedload volumes treated. Backflushing the filter units had no appreciable impact on treatment performance.
Discussion
Quality of influent and effluent
The copper concentrations analysed in the influent (916 μg/l–2124 μg/l) were in line with what previous studies have reported. (Athanasiadis, Helmreich, and Horn Citation2007) reported event mean concentration of 1623 μg/l in copper roof runoff. Also in line with the study by (Athanasiadis, Helmreich, and Horn Citation2007), most of the copper was in the dissolved phase. The high zinc concentrations found in the runoff in the present study are likely to have originated from the gutters and other building elements made of zinc galvanised metals or other alloys containing zinc, as described by (Müller and Österlund et al. Citation2020).
The aluminium in the influent could be explained by the transport of soil particles from the small park area, which was included in the drainage catchment of the filter system. Compared to the rest of the sampling events, significantly higher particulate aluminium concentrations in the inflow samples were identified during the sampling event in May 2019 (). One possible explanation for this is that a rain event (10 mm of precipitation) occurred one day before the sampling event, following a period of 39 antecedent dry days.
A fairly large proportion of the effluent aluminium concentration was in the solid phase (24–78%), indicating either degradation of the filter material – since aluminium is one of the major constituents of zeolites (Ćurković, Cerjan-Stefanović, and Filipan Citation1997) – or small influent aluminium particles passing through the zeolite filter.
One of the ways in which to increase cation exchange capacity is to pretreat the zeolites by rinsing them with a brine solution such as sodium chloride prior to installation (Hedström Citation2001). During filter operation, when positive ions in the runoff are filtered through the zeolite, the previously adsorbed sodium is released and exchanged for copper and zinc ions, for instance: this provides the likely explanation for the sodium concentrations in the effluent.
While guideline values for copper concentration in treated water in Sweden are sparse, the environmental authority of the municipality of Gothenburg has recommended a copper concentration in water discharged to receiving water of below 10 μg/l (Göteborgs Stad Citation2013). This recommended discharge limit is significantly lower than what was observed in the effluent in the present study, where effluent concentrations ranged from 350 to 600 μg/l.
The bacteria analysis showed that there were few or no bacteria present in the inflow. There was a significant concentration of intestinal enterococci in the outflow on one occasion. The values exceeded the recommended guidelines for bathing waters (European Parliament Citation2006). Both E. coli and intestinal enterococci were below the limit of detection in both the influent and the effluent for the sampling events in November 2019 and March 2020. This was possibly due to sample handling, as the ambient temperature was significantly colder (0°C and 8°C) than at the time of the sampling event in September 2019, when the outdoor temperature was 20°C.
Treatment efficiency with regard to Copper
Although the average reduction of both total and dissolved copper was relatively high during the evaluation period (69% and 73%, : Change in the treatment efficiency of total copper and zinc in relation to bedload volumes treated. Treatment efficiency (%) of the zeolite filter under investigation for total and dissolved copper and zinc), the effluent concentrations were still high and the treatment efficiency did not reach the levels reported in a previous study (Athanasiadis, Helmreich, and Horn Citation2007) or by the filter manufacturer 3 (P Technik Citation2020). (Athanasiadis, Helmreich, and Horn Citation2007) concluded that the treatment system could reach a treatment level of up to 95% in respect to total copper. Similar to the results presented here, copper was mostly present in the dissolved state in the study performed by (Athanasiadis, Helmreich, and Horn Citation2007). One explanation for the lower treatment efficiency could be the high hydraulic load on the filter in the present study. (Färm Citation2002) found that the filter systems containing zeolite performed well at hydraulic loads below 3 m3 m–2h–1, which is considerably lower than the filter load presented here (6.7 m3 m–2h–1).
Figure 5. Change in the treatment efficiency of total copper and zinc in relation to bedload volumes treated.
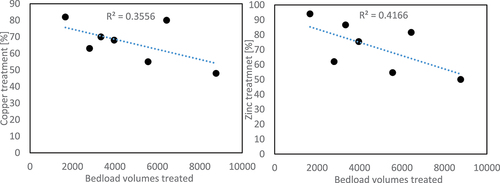
In the experiment performed by (Athanasiadis, Helmreich, and Horn Citation2007), roof runoff was passed through the filter by gravity. Due to area limitation, the system presented in this study included a pump with a relatively high flow rate, reducing the time during which water remains in contact with the filter medium.
Long-term efficiency projections
The filter material is supposed to provide efficient runoff treatment throughout its lifetime. For this filter system, the stated filter life was three years (3P Technik Citation2020). If the current deterioration rate were taken into account, it would be possible to predict the treatment rate at the end of its assumed lifecycle. For the purpose of this prediction, it was assumed that the annual rainfall would be equal to the average annual rainfall between 1999 and 2019 (575 mm/year (SMHI Citation2019)). With this, and with the linear regression line for copper (), the treatment level by the end of the filter lifecycle would be 25%. Using the regression line for zinc, the treatment level was projected to drop to 7% after an operating period of three years. If the average influent concentration were the same at the end of the projected period as during the sampling period, a prediction with linear regression would result in effluent concentrations of 1500 μg/l and 90 μg/l for copper and zinc, respectively, at the end of an operating period of three years.
Possible improvements to the treatment system set-up
The hydraulic loading rate and the contact time between the filter medium and stormwater are one of the key parameters that impact treatment efficiency in stormwater treatment systems in filter systems (Oter and Akcay Citation2007; Nehrenheim and Gustafsson Citation2008; Genç-Fuhrman, Mikkelsen, and Ledin Citation2016). One option for improving the treatment efficiency of the filter investigated in this study could be to decrease the flow rate of the pump in the influent tank, thereby decreasing the hydraulic load and increasing the contact time between the stormwater and the filter medium. The limiting factor in this case would be the volume of the storage unit, as a decrease in flow could potentially lead to flooding of the storage unit and damage to the premises. Hence, rain intensities and return periods of rains need to be considered as well so as to determine the risk of such flood events.
Conclusions
The aim of the study presented here was to evaluate the stormwater treatment system treating copper roof runoff with zeolite as a filter material in a field experiment. Focus was put on total and dissolved copper and zinc treatment over the period of 16 months. The sampling event mean concentrations for copper and zinc amounted to 916 μg/l–2124 μg/l and 186–464 μg/l, respectively. In the effluent, the average values for copper and zinc decreased to 916 μg/l–2124 μg/l and 19–121 μg/l, respectively. The treatment efficiency for total and dissolved copper varied between 52–82% and 48–94%, respectively. The treatment of zinc was in the range of 49–85% and 48–64% for total and dissolved zinc, respectively. While the treatment level was relatively high, the outlet concentration was still 35–60 times higher than the recommendation from the environmental authority of relevant environmental authorities. This alongside the decreasing performance during the monitored period suggest that the hydraulic loading of the filter system is too high to ensure proper pollutant removal.
Acknowledgement
The authors would like to thank Svante Nilsson, Mikael Östman and Håkan Andersson of the National Property Board of Sweden for their support during the sampling procedures, as well as Kerstin Nordqvist for her valuable input and help with the laboratory analysis.
Disclosure statement
No potential conflict of interest was reported by the author(s).
Additional information
Funding
References
- Athanasiadis, K., B. Helmreich, and H. Horn. 2007. “On-site Infiltration of a Copper Roof Runoff: Role of Clinoptilolite as an Artificial Barrier Material.” Water Research 41 (15): 3251–3258. doi:https://doi.org/10.1016/j.watres.2007.05.019.
- Babel, S., and T. A. Kurniawan. 2003. “Low-cost Adsorbents for Heavy Metals Uptake from Contaminated Water: A Review.” Journal of Hazardous Materials 97 (1–3): 219–243. doi:https://doi.org/10.1016/S0304-3894(02)00263-7.
- Behroozi, A., M. Arora, T. D. Fletcher, and A. W. Western. 2020. “Sorption and Transport Behavior of Zinc in the Soil; Implications for Stormwater Management.” Geoderma 367 (May). Elsevier B.V. doi: https://doi.org/10.1016/j.geoderma.2020.114243.
- Cettner, A., R. Ashley, A. Hedström, and M. Viklander. 2014. “Sustainable Development and Urban Stormwater Practice.” Urban Water Journal 11 (3): 185–197. doi:https://doi.org/10.1080/1573062X.2013.768683.
- Ćurković, L., Š. Cerjan-Stefanović, and T. Filipan. 1997. “Metal Ion Exchange by Natural and Modified Zeolites.” Water Research 31 (6): 1379–1382. doi:https://doi.org/10.1016/S0043-1354(96)00411-3.
- De Buyck, P. J., S. W. H. Van Hulle, A. Dumoulin, and D. P. L. Rousseau. 2021. “Roof Runoff Contamination: A Review on Pollutant Nature, Material Leaching and Deposition”. Reviews in Environmental Science and Biotechnology 20: 549–606.Springer: Netherlands. doi: https://doi.org/10.1007/s11157-021-09567-z.
- Doula, M., A. Ioannou, and A. Dimirkou. 2002. “Copper Adsorption and Si, Al, Ca, Mg, and Na Release from Clinoptilolite.” Journal of Colloid and Interface Science 245 (2): 237–250. doi:https://doi.org/10.1006/jcis.2001.7961.
- Ekstrand, S., P. Östlund, and C. Hansen. 2000. “Digital Air Photo Processing for Mapping of Copper Roof Distribution and Estimation of Related Copper Pollution.” Water, Air, & Soil Pollution 1 (3–4): 267–278. doi:https://doi.org/10.1023/A:1017576709802.
- EN 1189:1997. 1997. Water Quality - Determination of Phosphorus - Ammonium Molybdate Spectrometric Method. Brussels, Belgium: European committee for Standardisation.
- EN 1484:1997. 1997. Water Analysis - Guidelines for the Determination of Total Organic Carbon (TOC) and Dissolved Organic Carbon (DOC). Brussels, Belgium: European Committee for Standardization.
- EN 872:2005. 2005. Water Quality - Determination of Suspended Solids - Method by Filtration through Glass Fibre Filters. Brussels, Belgium: European Committee for Standardization.
- European Parliament. 2006. “Directive 2006/7/EC of the European Parliament and of the Council of 15 February 2006 Concerning the Management of Bathing Water Quality and Repealing Directive 76/160/EEC.” Official Journal of the European Union, L 064 (1882) 37–51. L 102/15.
- Färm, C. 2002. “Metal Sorption to Natural Filter Substrates for Storm Water Treatment—column Studies.” Science of the Total Environment. Elsevier 298 (1–3): 17–24. doi:https://doi.org/10.1016/S0048-9697(02)00142-0.
- Farreny, R., T. Morales-Pinzón, A. Guisasola, C. Tayà, J. Rieradevall, and X. Gabarrell. 2011. “Roof Selection for Rainwater Harvesting: Quantity and Quality Assessments in Spain.” Water Research 45 (10): 3245–3254. doi:https://doi.org/10.1016/j.watres.2011.03.036.
- Flemming, C. A., and J. T. Trevors. 1989. “Copper Toxicity and Chemistry in the Environment: A Review.” Water, Air, and Soil Pollution 44 (1–2): 143–158. doi:https://doi.org/10.1007/BF00228784.
- Genç-Fuhrman, H., P. S. Mikkelsen, and A. Ledin. 2016. “Simultaneous Removal of As, Cd, Cr, Cu, Ni and Zn from Stormwater Using High-efficiency Industrial Sorbents: Effect of pH, Contact Time and Humic Acid.” Science of the Total Environment. Elsevier B.V 566–567: 76–85. doi:https://doi.org/10.1016/j.scitotenv.2016.04.210.
- Göteborgs Stad. 2013. Miljöförvaltningens Riktlinjer Och Riktvärden För Utsläpp Av Förorenat Vatten till Recipient Och Dagvatten, 15. Gothenburg, Sweden.
- Gray, C. S, S. Burns, G. Jon. 2012. “The Use of Natural Zeolites as a Sorbent for Treatment.“ Paper presented at the GeoCongress, Oakland, California, March 2012.
- Hedström, A. 2001. “Ion Exchange of Ammonium in Zeolites: A Literature Review.” Journal of Environmental Engineering 20 (3): 269–276. doi:https://doi.org/10.1016/j.profnurs.2010.10.006.
- Helmreich, B., and H. Horn. 2010. Prüfung von Zwei Behandlungsanlage Für Zink- Bzw Kupferdachabflüsse nach den Prüfkriterien des LfU Bayern. Munich, Germany.
- ISO 11885:2007. 2007. Water Quality - Determination of Selected Elements by Inductively Coupled Plasma Optimal Spectrometry (ICP-OES). Geneva, Switzerland: International Organization for Standardization.
- ISO 17294:2004. 2004. Water Quality - Application of Inductively Coupled Plasma Mass Spectrometry (ICP-MS). Geneva, Switzerland: International Organization for Standardization.
- ISO 7899–2:2000. 2000. “Water Quality – Detection and Enumeration of Intestinal Entero- Cocci – Part 2 : Membrane Filtration Method (ISO 7899–2 : 2000) Del 2 : Membranfiltermetod.”
- Joint Committee For Guides In Metrology. 2008. “Evaluation of Measurement Data — Guide to the Expression of Uncertainty in Measurement.” International Organization for Standardization Geneva 50 (September): 134. doi:https://doi.org/10.1373/clinchem.2003.030528.
- Kandra, H. S., D. McCarthy, T. D. Fletcher, and A.Deletic. 2014. “Assessment of Clogging Phenomena in Granular Filter Media Used for Stormwater Treatment.” Journal of Hydrology 512 (May):518–527. Elsevier. doi: https://doi.org/10.1016/J.JHYDROL.2014.03.009.
- Kayhanian, M., C. Stransky, S. Bay, S. L. Lau, and M. K. Stenstrom. 2008. “Toxicity of Urban Highway Runoff with respect to Storm Duration.” Science of the Total Environment 389 (2–3): 386–406. doi:https://doi.org/10.1016/j.scitotenv.2007.08.052.
- Knowles, P., G. Dotro, J. Nivala, and J. García. 2011. “Clogging in Subsurface-flow Treatment Wetlands: Occurrence and Contributing Factors.” Ecological Engineering. Elsevier 37 (2): 99–112. doi:https://doi.org/10.1016/J.ECOLENG.2010.08.005.
- Kocasoy, G., and V. Şahin. 2007. “Heavy Metal Removal from Industrial Wastewater by Clinoptilolite.” Journal of Environmental Science and Health - Part A Toxic/Hazardous Substances and Environmental Engineering 42 (14): 2139–2146. doi:https://doi.org/10.1080/10934520701629617.
- Li, Y., D. T. McCarthy, and A. Deletic. 2016. “Escherichia Coli Removal in Copper-zeolite-integrated Stormwater Biofilters: Effect of Vegetation, Operational Time, Intermittent Drying Weather.” Ecological Engineering. Elsevier B.V 90: 234–243. doi:https://doi.org/10.1016/j.ecoleng.2016.01.066.
- Makepeace, D. K., D. W. Smith, and S. J. Stanley. 1995. “Urban Stormwater Quality: Summary of Contaminant Data.” Critical Reviews in Environmental Science and Technology 25 (2): 93–139. doi:https://doi.org/10.1080/10643389509388476.
- Müller, A., H. Österlund, J. Marsalek, and M. Viklander. 2020. “The Pollution Conveyed by Urban Runoff: A Review of Sources.” Science of the Total Environment 709: 136125. The Authors. doi: https://doi.org/10.1016/j.scitotenv.2019.136125.
- Müller, A., H. Österlund, K. Nordqvist, J. Marsalek, and M. Viklander. 2019. “Building Surface Materials as Sources of Micropollutants in Building Runoff: A Pilot Study.” Science of the Total Environment 680: 190–197. doi:https://doi.org/10.1016/j.scitotenv.2019.05.088.
- Nehrenheim, E., and J. P. Gustafsson. 2008. “Kinetic Sorption Modelling of Cu, Ni, Zn, Pb and Cr Ions to Pine Bark and Blast Furnace Slag by Using Batch Experiments.” Bioresource Technology 99 (6): 1571–1577. doi:https://doi.org/10.1016/j.biortech.2007.04.017.
- Okaikue-Woodi, F. E. K., K. Cherukumilli, and J. R. Ray. 2020. “A Critical Review of Contaminant Removal by Conventional and EmergingMedia for Urban Stormwater Treatment in the United States.” In Water Research, 116434. United Kingdom: Elsevier. doi:https://doi.org/10.1016/j.watres.2020.116434.
- Oter, O., and H. Akcay. 2007. “Use of Natural Clinoptilolite to Improve Water Quality: Sorption and Selectivity Studies of Lead(II), Copper(II), Zinc(II), and Nickel(II).” Water Environment Research 79 (3): 329–335. doi:https://doi.org/10.2175/106143006x111880.
- P Technik (2020) Hydrosystem 1000. Available at: www.3ptechnik.de/index.php?article_id=23&clang=4&model=3100130&t=true (Accessed 22 June 2020).
- Pitcher, S. K. K., R. C. T. C. T. Slade, and N. I. I. Ward. 2004. “Heavy Metal Removal from Motorway Stormwater Using Zeolites.” Science of the Total Environment 334–335: 161–166. doi:https://doi.org/10.1016/j.scitotenv.2004.04.035.
- Reddy, K. R., T. Xie, and S. Dastgheibi. 2014a. “Adsorption of Mixtures of Nutrients and Heavy Metals in Simulated Urban Stormwater by Different Filter Materials.” Journal of Environmental Science and Health - Part A Toxic/Hazardous Substances and Environmental Engineering 49 (5): 524–539. doi:https://doi.org/10.1080/10934529.2014.859030.
- Reddy, K. R., T. Xie, and S. Dastgheibi. 2014b. “Removal of Heavy Metals from Urban Stormwater Runoff Using Different Filter Materials.” Journal of Environmental Chemical Engineering 2 (1): 282–292. doi:https://doi.org/10.1016/j.jece.2013.12.020.
- Ruxton, G. D. 2006. “The Unequal Variance T-test Is an Underused Alternative to Student’s T-test and the Mann-Whitney U Test.” Behavioral Ecology 17 (4): 688–690. doi:https://doi.org/10.1093/beheco/ark016.
- SMHI (2019) Meteorogical observations - total precipitation (monthly) - station number 98210 Vasastaden. Available at: https://www.smhi.se/data/meteorologi/ladda-ner-meteorologiska-observationer/#param=precipitationMonthlySum,stations=all,stationid=98210 (Accessed 18 February 2020).
- Svensk Standard SS 02 81 67. 1996. Coliform Bacteria, Thermotolerant Coliform Bacteria and Escherichia Coli in Water – Determination with the Membrane Filtration Method (MF). Stockholm, Sweden: Swedish Standards Institution.
- Turco, M., G. Brunetti, S. A. Palermo, G. Capano, G. Grossi, M. Maiolo, and P. Piro. 2020. “On the Environmental Benefits of a Permeable Pavement: Metals Potential Removal Efficiency and Life Cycle Assessment.” Urban Water Journal. 17 (7): 619–627. Taylor and Francis Ltd. doi:https://doi.org/10.1080/1573062X.2020.1713380.
- US EPA. 2006. Data Quality Assessment: Practitioners Statistical Methods for Practitioners, 1–190. Washington, D.C., U.S.
- Walsh, C. J., A. H. Roy, J. W. Feminella, P. D. Cottingham, P. M. Groffman, and R. P. Morgan. 2005. “The Urban Stream Syndrome: Current Knowledge and the Search for a Cure.” Journal of the North American Benthological Society 24 (3): 706–723. The University of Chicago Press. doi:https://doi.org/10.1899/04-028.1.
- Ziyath, A. M., P. Mahbub, A. Goonetilleke, M. O. Adebajo, S. Kokot, and A. Oloyede. 2011. “Influence of Physical and Chemical Parameters on the Treatment of Heavy Metals in Polluted Stormwater Using Zeolite—A Review.” Journal of Water Resource and Protection 03 (10): 758–767. doi:https://doi.org/10.4236/jwarp.2011.310086.