Abstract
Three azomethine polymers with bent-core mesogens in their main chains were synthesized via polycondensation, and the effect of the central bent-core structure (Ar=1,2-phenylene, 2,3-naphthylene, or 2,7-naphthylene) was studied. Although polymers contain bent-shaped mesogens in their main chains, no polymer showed good solubility in common organic solvents but all polymers exhibited liquid crystalline and semi crystalline properties. Two of the three polymers showed thermal degradation before melting due to the high melting point. The polymer with 2,3-naphthylene had a 229°C melting point. The optical texture of the smectic phase could be observed using quenched samples from the melt state. During heating, the smectic layer structure could be detected via X-ray diffraction.
Keywords:
Introduction
In 1996, Takezoe and colleagues [Citation1] reported the first obvious example of the chiral smectic phase derived from an achiral-banana-shaped molecule. In 1997, Clark and colleagues [Citation2] proposed the layer structure of the polar smectic C phase in bent-core mesogens. Since then, over nearly 15 years, a considerable number of studies have been conducted on the structure–property relationships of bent-core molecules [Citation3–7]. By using these achiral molecules, intriguing electro-optical characteristics can be obtained, such as ferroelectric, antiferroelectric, biaxial, and flexoelectric properties.
The polymers with bent-core mesogens in the main chain can be applied to the emerging information display technology areas because the unique electro-optical properties shown by the single mesogenic molecules can manifest in the polymers. Although a considerable number of studies have been conducted on the structure–property relationships of bent-core compounds, the research on polymers containing bent-core mesogens in the main chain is still limited [Citation8–14]. In 2003, Fodor-Csorba and colleagues [Citation9] reported the first synthesis of the main-chain liquid crystalline polymers (LCP) with banana-shaped mesogens by alternating diene metathesis polycondensation, and they later found that these polymers form a nematic phase [Citation10]. In 2004, the authors of the present study reported the first synthesis and properties of main-chain polymers containing bent-core mesogens with an obtuse-subtended angle, which could form B1 and B2 mesophases, but the high viscosity and high phase transition temperature restricted the further investigation of the electro-optical properties [Citation11]. Recently, these authors focused on polymers containing V-shaped bent-core mesogens with acute-angled configurations in their main chains and found that in general these polymers with a lateral fluorine or chlorine substituents were semicrystalline in the solid state and could form smectic C phases in the melt state [Citation12].
Reported in this work are the synthesis and smectogenic property of azomethine polymers with bent-core mesogens in their main chains. To assess the effects of the changes in the central units, the polymers containing bent-core mesogens with azomethine linkages and dioxydodecamethylene flexible spacers in their main chains were synthesized; their central units were varied with 1,2-dioxyphenylene, 2,3-dioxynaphthylene, and 2,7-dioxynaphthylene (Scheme 1). Their mesomorphic properties were investigated using differential scanning calorimetry (DSC), polarizing optical microscopy (POM), and X-ray diffraction (XRD).
Experimental Section
Synthesis
The synthetic route to the poly(azomethine)s is shown in Scheme 1. The monomers were prepared according to synthetic procedures similar to those reported in the literature, with minor modifications [Citation11,Citation12]. As the synthetic procedures that were used to prepare the polymers were essentially the same, one representative polymer, 3a, is given in the following: 2,7-naphthylene bis(4-formylbezozte) (0.60 g, 1.42 mmol) and 1,12-bis(4-aminophenoxy)dodecane (0.54 g, 1.42 mmol) were dissolved in 30 mL dry dichloromethane and were evaporated for drying at 80°C. The residue was dissolved in a mixture (50 mL) of 1-methyl-2-pyrrolidinone (NMP) and hexamethylphosphoramide (HMPA) (4:1 v/v). The solution was heated to 120°C with stirring for 12 h. After reaction, the mixture was cooled to ambient temperature and then poured into methanol. The precipitated polymer was thoroughly washed with water and methanol in turns [Yield: 85.6%; IR (KBr Pellet, cm−1): 3065 (aromatic C–H, st); 2934, 2865 (aliphatic C–H, st); 1735 (, st); 1622, 1599 (aromatic
, st); 1680 (
, st); 1245, 1134, 1065 (C–O, st)].
Instruments
IR and NMR spectra were obtained with a Jasco 300E FT/IR and a Bruker DPX 200 MHz NMR spectrometer. The transition behaviors were characterized via DSC (Netzsch DSC 200 F3 Maia). DSC measurements were performed in a N2 atmosphere, with 10°C/min heating and cooling rates. Optical texture observation was carried out using a polarizing microscope (Axioskop 40 Pol) with a heating stage (Mettler FP82HT). XRD was performed in transmission mode with synchrotron radiation () for polymers 3b and3c at the Pohang Accelerator Laboratory in South Korea. The sample sealed with a window of a 7-μm-thick Kapton film on both sides was held in an aluminum sample holder. The data are presented as a function of q=4π sin
(θ: scattering angle). The sample was heated with two cartridge heaters, and its temperature was monitored with a thermocouple placed close to the sample. Background scattering correction was performed by subtracting the scattering from the Kapton film. The XRD patterns for polymer 3a were measured using a Rigaku SWXD (X-MAX/2000-PC) at a given temperature.
Results and discussion
Three azomethine polymers were synthesized to generate smectic phases in the main-chain polymers with bent-core mesogens. A mixture of NMP/HMPA (4:1 v/v) was used as a co-solvent for the polycondensation [Citation11]. Once products were obtained as precipitates, no polymers were soluble in common organic solvents. Thus, the solution viscosities of the polymers could not be measured. As given in , the yields of the polymers were in the range of 80–88%. This indicates that the low-molecular-weight portions were somewhat removed by the precipitation process. The structures of the polymers with ester, azomethine, and ether linkages were characterized via IR spectroscopy. The spectral data were in accordance with the expected formula. All the obtained polymers reported herein were studied via POM, DSC, and XRD at variable temperatures to characterize their liquid-crystalline behavior.
Table 1 Yields, thermal transition temperatures, and enthalpy changes of the polymersa.
shows the DSC thermograms of the polymers. In , the transition temperatures and enthalpy changes defined by DSC are presented. It was interesting that although the polymers contain bent-shaped mesogens in their main chains, all of them were semicrystalline. Two of the three polymers (except polymer 3a) showed distinctive melting temperatures (Tm). Polymer 3a with Ar = 2,7-naphthylene exhibited no transition during heating because thermal degradation occurred before the melting. Polymers 3b and 3c demonstrated the multiple melting phenomena showing a 195°C solid-to-solid transition temperature (Tk− k). The melting temperature (Tm) of polymer 3b was defined as 229°C, but the Tm of polymer 3c could not be determined due to the thermal decomposition. Note that the for polymer 3b with 2,3-naphthylene was comparable with those of analogue polymers with fluorine or chlorine substituent [Citation12]. This indicates that the 2,3-naphthlene central unit has an advantage for molecular packing in the solid state over the 1,3-phenylene central unit due to the so-called interlocking effect, which is not contradictory to the previous report that the analogous polymers generate oscillation of the chain backbone along the director direction [Citation14]. No isotropization temperature could be defined for all the polymers, however, because thermal degradation occurred before the isotropization temperature was reached. Nevertheless, it was confirmed that all the polymers were liquid crystalline. Based on the results of the microscopic observation and XRD measurement, the phase sequence determined via DSC on the heating and cooling cycles was confirmed.
– show the cross-polarizing optical micrographs. For polymer 3a (Ar=2,7-naphthylene), the smectic optical texture can be observed only by quenching the sample from the melt state. Meanwhile, for polymer 3b (Ar=2,3-naphthylene) and polymer 3c (Ar=1,2-phenylene), a smectic broken fan texture was identified after shearing at 250°C.
Figure 2. Cross-polarizing optical micrographs of polymer 3a (magnification×250): (a) T=240°C; and (b) quenched sample of the melt.
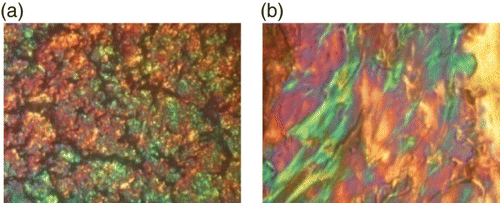
Figure 3. Cross-polarizing optical micrographs of polymer 3b (magnification×250): (a) T=150°C; (b) T=200°C; (c) T=220°C; and (d) after shearing at 250°C.
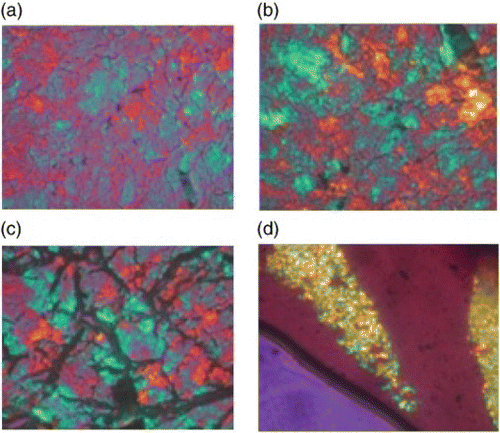
Figure 4. Cross-polarizing optical micrographs of polymer 3c (magnification×250): (a) T=150°C; (b) T=200°C; (c) T=250°C; and (d) after shearing at 250°C.
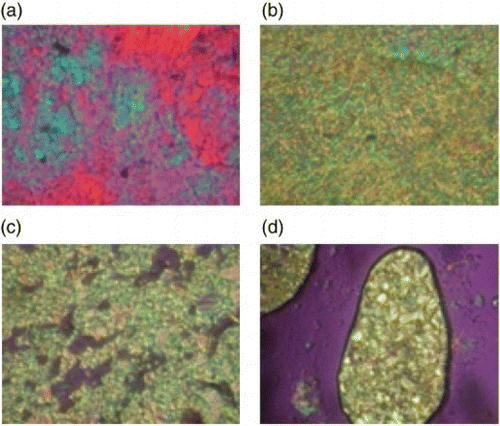
show the XRD patterns of the polymers at different temperatures. As can be seen from , polymer 3a showed some strong peaks at room temperature, indicating a crystalline state. Even when the sample was heated to a higher temperature, this pattern was maintained until thermal degradation. As can be seen from , polymer 3b showed several peaks at room temperature, indicating a crystalline state. This XRD pattern remained when the polymer was heated to 160°C and 215°C (higher than the 195°C Tk−k defined by DSC). When further heated to 280°C (higher than the 229°C Tm defined by DSC), only a strong peak appeared at q=2.06 nm−1 (d=3.05 nm) in the small-angle region, and no peaks appeared in the wide-angle region. This is indicative of the smectic phase. Sequentially, the sample was cooled to 210°C, 110°C, and room temperature, and no change in the XRD pattern was observed due to the thermal degradation. Even when the sample was heated to a temperature higher than 300°C, however, the peak of the small-angle region did not disappear, but degradation occurred.
Figure 7. XRD patterns of polymer 3c at different temperatures: (a) heating to a moderate temperature so that thermal degradation would not occur and (b) heating to a high temperature so that the isotropic phase could be obtained.
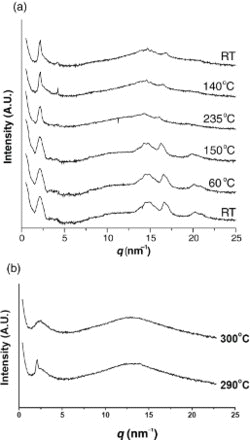
As can be seen from , at room temperature, polymer 3c showed XRD patterns indicating a crystalline state. When heated to 60°C and 150°C (lower than the 195°C first Tk−k defined by DSC), the crystalline XRD patterns were maintained. When heated to 235°C, in the wide-angle region, the peak intensity decreased, but the peak did not disappear, whereas in the small-angle region, a strong peak persistently appeared at q=2.08 nm−1. Sequentially, when this sample was cooled to 140°C and room temperature, the XRD pattern for the crystalline state did not disappear. This means that the crystalline state remained. When another specimen was heated to 290°C and 300°C, polymer 3c showed the XRD pattern for the smectic and isotropic phases, respectively, but after heating to 300°C, the reversible patterns could not be obtained due to the severe thermal degradation.
As given in , by assuming the molecular chain to be fully extended in the all-trans-conformation, the lengths of the repeating units of polymers 3b and 3c can be calculated to be 36.5 and 35.5 nm, respectively. Thus, the d-values of polymers 3b and 3c can be estimated to be 3.02 and 3.05 nm, respectively, and the tilt angles (θ) of the mesogens normal to the layer can be estimated to be 34 and 32°, respectively. Therefore, it can be concluded that these two polymers form the smectic C phase, as shown in .
Table 2 XRD data for the smectogenic polymers.
Conclusions
In summary, in this study, three main-chain azomethine polymers containing bent-core mesogens with acute/obtuse-angled central cores (Ar=2.7-naphthylene, 2,3-naphthylene, and 1,2-phenylene) were synthesized, and the influence of the structure of the central units was evaluated. In spite of the introduction of the bent-shaped mesogen into the main chain, all the polymers were semicrystalline, and their solubility was very poor. Furthermore, all the polymers were smectogenic liquid crystalline. Interestingly, it was seen that in spite of the acute-subtended angle and the extruded second benzene ring, polymers 3b and 3c could form the smectic C phase. This implies that molecular alignment is possible along the bent direction in the melt state.
Funding
This research was supported by Basic Science Research Program through a National Research Foundation of Korea (NRF) grant funded by the Ministry of Education, Science, and Technology (No. NRF-2013R1A1A2012471).
References
- T. Niori, T. Sekine, J. Watanabe, T. Furukawa, and H. Takezoe, J. Mater. Chem. 6, 1231 (1996).
- D.R. Link, G. Natale, R. Shao, J.E. Maclennan, M.A. Clark, E. Korblova, and D.M. Walba, Science 278, 1924 (1997).
- G. Pelzl, S. Diele, and W. Weissflog, Adv. Mater. 11, 707 (1999).
- D. Shen, A. Pegenau, S. Diele, I. Wirth, and C. Tschierske, J. Am. Chem. Soc. 122, 1593 (2000).
- H.N. Shreenivasa Murthy and B.K. Sadashiva, Liq. Cryst. 29, 1223 (2002).
- W. Weissflog, H.N. Shreenivasa Murthy, S. Diele, and G. Pelzl, Phil. Trans. R. Soc. A 364, 2657 (2006).
- H. Takezoe and Y. Takanishi,. Jpn. J. Appl. Phys. 45, 597 (2006).
- X. Chen, K.K. Tenneti, C.Y. Li, Y. Bai, X. Wan, X. Fan, Q.-F. Zhou, L. Rong, B.S. Hsiao, Macromolecules 40, 840 (2007).
- S. Demel, C.F.S. Slugovc, K. Fodor-Csorba, and G. Galli, Macromol. Rapid Commun. 24, 636 (2003).
- K. Fodor-Csorba, A. Jakli, and G. Galli, Macromol. Symp. 218, 81 (2004).
- E-J. Choi, J.C. Ahn, L.C. Chien, C.K. Lee, W.C. Zin, D.C. Kim, and S.T. Shin, Macromolecules, 37, 71 (2004).
- E-J. Choi, E.-C. Kim, C.-W. Ohk, W.-C. Zin, J.-H. Lee, and T.-K. Lim, Macromolecules 43, 2865 (2010).
- N. Gimeno, A. Sanchez-Ferrer, N. Sebastian, R. Mezzenga, and M. Blanca Ros, Macromolecules 44, 9586 (2011).
- M. Tokita, K. Kato, R. Ishige, S. Okuda, S. Kawauchi, K. Okoshi, S. Kang, and J. Watanbe, Polymer 52, 5830 (2011).