ABSTRACT
Introduction: CD19+CD24hiCD38hi regulatory B cells (Bregs) and CD19+CD27+ memory B cells (Bmems) are B cell subsets with specific immunoregulatory properties. In this study, the balance of these subsets was investigated in pediatric immune thrombocytopenia (ITP) patients, and the frequencies of Bregs and Bmems before and after first-line therapy were measured.
Methods: Forty-nine pediatric ITP patients and 19 normal controls were enrolled in this study. The total CD19+ B cells, Bregs and Bmems in the peripheral blood (PB) of all cases were measured by flow cytometry.
Results: We found higher frequencies of total CD19+ B cells and Bmems in newly diagnosed ITP patients than those in normal controls (p < 0.01), whereas the frequencies of CD19+CD24hiCD38hi Bregs was significantly lower in ITP patients (p < 0.001). After therapy with MP + IVIG, the level of CD19+CD24hiCD38hi Bregs and Bmems were almost normalized.
Conclusion: Our results indicated that pediatric ITP patients were characterized by a decline in CD19+CD24hiCD38hi Bregs and increment of CD19+CD27+Bmems, and an increase of total CD19+ B cells in their peripheral blood.
Introduction
Immune thrombocytopenia (ITP) is an acquired autoimmune disease. It is the most common cause of hemorrhagic symptoms in children which is characterized by single thrombocytopenia accompanied with normal white blood cell count and hemoglobin. ITP can be classified clinically as acute/newly diagnosed (< 3 months), persistent (3–12 months) and chronic (> 12 months) [Citation1]. Although most ITP patients initially respond to first-line treatment, a large proportion of patients are unable to reach remission or would succumb to relapse. Furthermore, a proportion of patients with ITP are refractory to multiple treatments, which greatly increases the difficulty of treatment for patients with ITP. The pathogenesis of ITP remains enigmatic, although many researchers are highly considering the involvement of T cells and antibodies. However, in some cases, ITP is the result of primary immunodeficiency. Some scholars have proposed several mechanisms, including the failure of central and peripheral tolerance mechanisms, where the negative selection process is defective, which resulted in an increase of CD21 low B cells [Citation2], decreased number class-switch memory B cells and FOXP3 regulatory T cells [Citation3,Citation4]. A recent study suggests that ITP might be caused by endogenous B cell hyperfunction which results in a variety of specific antibodies including the anti-platelet antibody [Citation5]. CD19+CD24hiCD38hi regulatory B cells (Bregs), also known as immature/transitional B cells, play a conservatory role in autoimmune diseases through the production of interleukin-10 (IL-10) [Citation6]. The importance of Bregs is illustrated by the fact that Bregs deficiency can lead to the onset and deterioration of various autoimmune diseases, such as systemic sclerosis, Graves’ disease and systemic lupus erythematosus (SLE) [Citation7–9]. Furthermore, Li et al. [Citation10] indicated that a lower frequency and impaired function of Bregs in adult ITP, suggesting a possibility of Bregs dysregulation in ITP.
CD19+CD27+ cells are also known as human total memory B cells (Bmems). They are considered a source of inflammatory factors responsible for the pathogenesis of autoimmunity [Citation11]. Moreover, Kim et al. [Citation12] showed that CD27+ memory B cells in the blood were used to indicate the efficacy of rituximab treatment in patients with neuromyelitis optica (NMO). Since both Bmems and CD19+CD24hiCD38hi Bregs are important immunoregulatory B cell subsets, it is plausible to assume they reach an immune balance in vivo. To date, the immune balance of these B cells subsets in ITP patients, especially pediatric patients has not been well characterized.
The significance of the dysfunction of Bmems and Bregs in compromising immune tolerance in pediatric ITP is still unclear. We hypothesize that the evaluation of the Bmems/Bregs immune balance would lay the foundation for the assessment of disease progression and prognosis of pediatric ITP patients. In the current study, we examined the occurrence of blood Bmems and Bregs to reveal their immune balance status in newly diagnosed and recovered pediatric ITP patients as well as normal control.
Materials and methods
Patients and controls
The study was approved by the Ethics Committee of the Sun Yat-Sen University. Written informed consent was obtained from each participant. All pediatric ITP patients were diagnosed based on the international working group guideline [Citation1]. We analyzed peripheral blood samples from 49 pediatric ITP patients and 19 healthy children at Sun Yat-sen University Hospital. Among the patients, 17 were newly diagnosed patients (8 males and 9 females at the range of 1–10 years old; median age = 3 years old) without treatment and 32 (17 males and 15 females at the range of 2–11 years old; median age = 5 years old) were in recovery after treatment with methylprednisolone (MP) plus intravenous immunoglobulin (IVIG). ITP patients showed symptoms of fever and skin mucosal bleeding. No visceral or intracranial hemorrhage was found in all cases. There were no obvious abnormalities in the physical examination, imaging examination and blood cell morphology in all cases, and the HIV antibody tests were all negative. Pediatric ITP patients with hypo-proliferative acute leukemia, myelodysplastic syndromes, aplastic anemia, hereditary thrombocytopenia, infection and drug factors, primary immunodeficiency and secondary immune deficiency disease were excluded. The clinical characteristics of 49 ITP patients and 19 normal children are shown in . Pediatric patients were considered to be in remission if: (1) PLT ≥ 100 × 109/L and the bleeding stopped after treatment was finished. (2) PLT ≥ 30 × 109/L and at least 2 times higher than the basal PLT value, with no bleeding detected. The PLT was detected twice with the time interval of more than 7 days from the day of therapeutic efficacy evaluation. Furthermore, 19 age- and gender-matched healthy children were recruited during the same period and the cohort of age and condition were no significant difference (p > 0.05).
Table 1. Clinical characteristics of pediatric ITP and normal control.
Samples and treatment
Fasting venous blood samples from newly diagnosed pediatric ITP patients were collected between 8:00 and 9:00 am on the second day of hospitalization. Blood samples of pediatric ITP patients in remission were collected 4 (3–6) weeks after the first-line therapy with MP plus IVIG. Fasting venous blood samples of healthy controls was collected between 8:00 and 9:00 am All samples were tested within 8 h of collection. The first-line therapy protocol is given as follows: MP (2 mg kg−1 d−1) was given orally for 4 weeks. The dosage was kept for 1–2 weeks when PLT ≥ 100 × 109/L and gradually decreased until drugs withdrawal. IVIG (400 mg kg−1 d−1 × 5d) was administered through intravenous infusion for five days. The average time needed to reach remission was 5 weeks.
Multiparameter flow cytometry analysis
The following fluorochrome-conjugated monoclonal antibodies were used: CD24-PE (Clone: ALB9), CD19-ECD (Clone: J3-119), CD38-PC5 (Clone: LS198.43), CD45-PerCp7 (Clone: J33), mouse IgG1-FITC (Clone: 679.1Mc7), mouse IgG1-PE (Clone: 679.1Mc7), mouseIgG1-ECD (Clone: 679.1Mc7), mouse IgG1-PC5 (Clone: 679.1Mc7) and erythrocyte lysis solution (were all purchased from Beckman Coulter). FITC-CD27 (Clone: L128) was purchased from BD Biosciences. Briefly, 100 µL of whole blood was immunostained for 30 min in the dark, followed by lysis with 500 µL of erythrocyte lysis solution before two washes with 500 µL of PBS. After centrifugation, the supernatants were discarded, and cell pellets were resuspended in 500 µL of PBS for analysis. Total CD19+ B cells, CD19+CD24hiCD38hi Bregs, and CD19+CD27+ memory B cells were distinguished with a Navios Flow Cytometer (Beckman Coulter). The Kaluza software 2.0 (Beckman Coulter) was used for data acquisition and off-line analysis.
Statistical analysis
Statistical analyses were conducted using SPSS 22.0 software. All data are presented as mean ± standard deviation. One-way ANOVA was performed for data comparison among multiple groups. Spearman’s correlation was performed to assess the correlation between variables. P < 0.05 was considered statistically significant.
Results
Flow cytometric analysis of total CD19+ B cells, Bregs and Bmems in pediatric ITP and normal controls
The gating strategy for Bregs and Bmems was shown in . Lymphocytes were first gated among all events according to side-scatter and CD45 (figure not shown). CD19+ cells were then gated in lymphocytes. Specific phenotypes of Bregs and Bmems are shown in gated CD19+ B cells, respectively.
Figure 1. Representative flow cytometry plots showing CD19+ B cells, Bmems and Bregs in pediatric ITP patients and normal controls. (A) Representative dot plots showing blood CD19+ B cells in newly diagnosed patients, patients in remission and normal controls. (B) Blood memory B cells (CD19+CD27+) in ITP patients and normal controls. (C) Blood Bregs (CD19+CD24hiCD38hi) in ITP patients and normal controls. N-ITP: newly diagnosed patients; R-ITP: patients in remission; NC: normal controls.
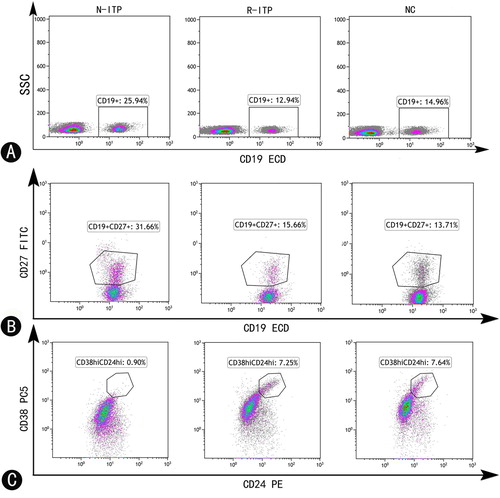
The frequency and absolute count (×109/L) of blood CD19+B cells in ITP patients
We measured the frequency and absolute count of blood CD19+B cells in ITP patients and normal controls. CD19+ B cells accounted for 24.72 ± 5.87% of lymphocytes in newly diagnosed patients, 16.44 ± 4.11% of lymphocytes in patients in remission, and 19.14 ± 3.86% in normal controls. Accordingly, newly diagnosed patients had the highest blood CD19+B cells (p = 0.000 vs. patients in remission; p = 0.002 vs. normal control). In addition, in comparison with normal controls, patients in remission had lower CD19+ B cells after treatment (p = 0.024) ((A)). The absolute CD19+ B cells count in N-ITP, R-ITP and normal controls were (0.8794 ± 0.5150) × 109/L, (0.5700 ± 0.2419) × 109/L and (0.7234 ± 0.2517) × 109/L, respectively ((B)). The absolute count of blood CD19+ B cells in N-ITP was substantially increased compared with R-ITP (p = 0.006), patients in remission had lower CD19+ B cells number compared with normal controls (p = 0.036).
Figure 2. The frequency and absolute count of total CD19+ B cells in lymphocytes. (A)The percentage of CD19+ B cells in each group. (B) The absolute count of CD19+ B cells in each group. N-ITP: newly diagnosed patients; R-ITP: patients in remission; NC: normal controls; *p < 0.05; **p < 0.01; ***p < 0.001.
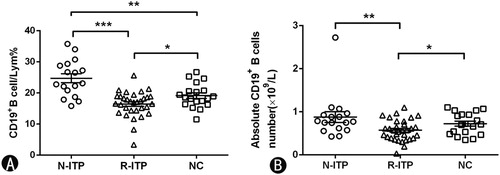
The frequency and absolute count (×109/L) of blood CD19+CD27+memory B cells in ITP patients
We then analyzed the frequency of blood CD19+CD27+ Bmems in ITP patients with/without remission and normal controls. As shown in (A), we observed a significant increase in the frequency of blood CD19+CD27+Bmems in newly diagnosed patients as compared to that in patients in remission (22.97 ± 7.20% vs. 15.92 ± 5.50%, p = 0.000) and in normal controls (22.97 ± 7.20% vs. 14.25 ± 3.46%, p = 0.000). Notably, after treatment with MP plus IVIG, the frequency of CD19+CD27+ Bmems almost returned to the normal level (p > 0.05). The absolute Bmems number in N-ITP, R-ITP and normal controls were (0.2097 ± 0.1642) × 109/L, (0.0918 ± 0.0534) × 109/L and (0.1016 ± 0.0389) × 109/L, as shown in (B). The absolute count of blood Bmems in N-ITP were increased compared with R-ITP (p = 0.001) and NC (p = 0.010). Moreover, the level of Bmems almost returned to normal (p > 0.05) after treatment.
The proportions and absolute number (×109/L) of blood CD19+CD24hiCD38hi Bregs in ITP patients
We also measured the proportions and absolute number of Bregs in ITP patients and normal controls. The proportion of CD19+CD24hiCD38hi Bregs within the total CD19+B cells was significantly lower in newly diagnosed patients than their proportions in patients in remission (2.96 ± 1.34% vs. 5.97 ± 2.83%, p = 0.000) and normal controls (2.96 ± 1.34% vs. 7.55 ± 2.98%, p = 0.001) ((A)). The frequency of CD19+CD24hiCD38hi Bregs within lymphocytes was also significantly lower in newly diagnosed patients as compared to their frequencies in patients in remission (1.01 ± 0.49% vs. 1.51 ± 0.75%, p = 0.017) and normal controls (1.01 ± 0.49% vs. 1.54 ± 0.79%, p = 0.023) ((B)). Furthermore, the level of CD19+CD24hiCD38hi Bregs were almost normal (p > 0.05) after the treatment. The absolute Bregs number in N-ITP, R-ITP and normal controls were (0.0279 ± 0.268) × 109/L, (0.0328 ± 0.0197) × 109/L and (0.0544 ± 0.0313) × 109/L, respectively ((C)). The absolute number of blood Bregs in NC had a higher number compared with N-ITP and R-ITP (p = 0.011 vs. N-ITP; p = 0.004 vs. R-ITP).
Figure 4. The frequency and absolute count of Bregs subsets in CD19+ B cells and lymphocytes. (A) The frequency of Bregs in CD19+ B cells. (B) The frequency of Bregs in lymphocytes. (C) The absolute count of Bregs in each group. N-ITP: newly diagnosed patients; R-ITP: patients in remission; NC: normal controls; *p < 0.05; **p < 0.01; ***p < 0.001.
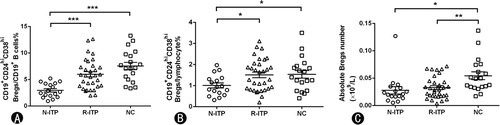
Discussion
The immune system is astonishingly complex, in which cellular and humoral immunity interact with each other to maintain a delicate immune balance. The frequencies and functions of regulatory immune cells largely determine the compromise of immune balance in autoimmune diseases like ITP. In the current study, we demonstrated the blood Bregs and Bmems in pediatric ITP patients and normal controls by flow cytometry. Our results confirmed that the frequencies of total CD19+ B cells and Bmems were significantly increased in newly diagnosed ITP patients. On the contrary, significantly declined CD19+CD24hiCD38hi Bregs were observed in newly diagnosed ITP patients. However, after first-line therapy, the frequencies of Bmems and CD19+CD24hiCD38hi Bregs cells returned towards their normal levels.
At present, most human memory B cells are CD27 positive, although some are CD27 negative [Citation13]. Memory B cells, which are produced in the germinal center, contribute to humoral immunity by rapidly differentiating into antibody-secreting plasma cells upon re-encountering antigens. Recent studies indicated that antibody-mediated autoimmune diseases are closely related to Bmems. Korganow et al. [Citation14] found that blood Bmems in SLE patients were significantly increased during the active period. Ameiro et al. [Citation15] showed that Bmems in PB and synovial fluid were significantly increased in patients with acute rheumatoid arthritis, and the level of Bmems returned to normal after treatment. In our study, we showed a significant increase in the frequency of Bmems in newly diagnosed patients, which is consistent with a previous study [Citation16].
Different IL-10-producing B cell subpopulations have been reported to express similar surface markers but exert different functions. They are universally referred to as Bregs [Citation17]. However, CD19+CD24hiCD38hi Bregs were reported to be the main source of IL-10, and they can inhibit T cell proliferation and Th1 polarization, and induce the expression of regulatory T cells [Citation18]. Although no relevant human studies have been reported, various mouse disease models showed that IL-10 produced by Bregs played a key role in controlling and maintaining the immune function of regulatory T cells and promotes differentiation [Citation19,Citation20]. Recent studies have shown that the absence of Bregs exacerbates autoimmune and chronic inflammatory diseases and promotes tumor progression [Citation21,Citation22]. In this study, Bmems were significantly elevated in newly diagnosed ITP cases while CD19+CD24hiCD38hi Bregs were significantly decreased. Indeed, in ITP patients, Asmaa M et al. showed the data of a reduction in Bmems with long duration of illness [Citation23]. However, we found a significant increase in the frequency of blood CD19+CD27+Bmems in newly diagnosed ITP patients. This difference in outcome may be due to differences in research methods or individual difference in disease cohort. A similar phenomenon of an increase in the frequencies of peripheral CD19+CD27+ Bmems have also been reported in patients with active ITP as compared with normal controls [Citation16]. Although the fundamental explanation for increased Bmems remains unknown, we hypothesized that CD19+CD24hiCD38hi Bregs might inhibit Bmems proliferation. Decreased Bregs in patients with ITP weaken inhibition of Bmems and thereby increased Bmems. However, this hypothesis needs further verification. Moreover, Bmems and CD19+CD24hiCD38hi Bregs returned to their normal levels after treatment, suggesting the restoration of Bregs/Bmems balance. Notably, CD19+CD24hiCD27+ Bregs, a CD27+ memory B cell subset, have been reported that possess regulatory function via producing IL-10 [Citation24], although the number is low. In addition, CD19+CD24hiCD27+ B cells could be a unique subset of activated memory B cells, so they are not identical to other memory B cells [Citation25]. CD27+ memory B cells can rapidly differentiate into antigen-dependent and antigen-independent plasmablasts, and could present antigens and secrete cytokines [Citation26,Citation27]. Memory B cells-derived plasmablasts can produce abundant and different autoantibodies to cause tissue damages. Therefore, the increase of Bmems might be a crucial contributing factor to ITP pathogenesis.
In our study, we observed a significant increase in the frequencies of Bregs while decreased of Bmems and total CD19+ B cells in pediatric ITP after first-line treatment. Although the detailed mechanism of this change is beyond the extent of our present study, In spite of its clinical efficacy, the precise mechanism by which IVIG inhibits pro-inflammatory immune responses remains unclear. Imbach et al. first reported that IVIG may reverse pediatric ITP patients [Citation28]. Some researchers revealed that IVIG can block Fc-receptors on macrophages of the reticuloendothelial system and/or regulate their expression or affinity, and IVIG may also affect cellular immunity by restoring the number and function of regulatory T cells [Citation29–31]. Hori et al. [Citation32]proposed a new immunosuppressive action of IVIG, which acts on steady-state B cells to inhibit their homeostatic expansion directly. On the other side, Gruver-Yates et al. revealed that dexamethasone can prompt B cells apoptosis in each stage of B cells maturation when they are isolated from the bone marrow and spleen via binding with the glucocorticoid receptor [Citation33]. On the other hand, glucocorticoid therapy promoted the viability of human B cells in vitro and supported the survival and development of human peripheral blood B cells [Citation34]. With these evidence, we hypothesized that Bmems might be more sensitive to the apoptotic effect of methylprednisolone compared to Bregs. Moreover, we conjectured that high dose methylprednisolone might enhance Bregs inhibited immunoregulatory function of CD19+CD27+ Bmems in pediatric ITP.
In this study, our data show a significant increase in the frequency of blood Bmems and decrease in the frequency of blood CD19+CD24hiCD38hi Bregs in pediatric ITP patients strongly indicated the imbalance of the CD19+CD24hiCD38hi Bregs and Bmems in pediatric ITP. Notably, after first-line therapy, the frequencies of both cell types returned to normal, suggesting that these cells could be used as clinical markers for evaluation of therapy efficacy and prognosis. However, the detailed mechanism of Bmems–Bregs interaction and the mechanisms of immunoregulation in pediatric ITP need to be further verified.
Acknowledgments
The authors are thankful to the staff of the department of clinical laboratory, Guangdong Provincial Key Laboratory of Malignant Tumor Epigenetics and Gene Regulation, and dermatology department at Sun Yat-Sen Memorial Hospital for assistance with result interpretation and collection of samples. We thank Xianghua Lin and Pheier Saw for providing the analysis software and for their guidance with the statistical analysis, and Songyin Huang, Xiaohong Luo for assistance with the data collection. Fang J and Lin L designed the research study, performed the research, analyzed the data and wrote the paper; Lin D and Duan C designed the research study, assisted with the research and analyzed the data; Zhang R and Liu X assisted with performing the research. Wang D assisted in obtaining data. All co-authors contributed to manuscript revision and have approved the final version.
Disclosure statement
No potential conflict of interest was reported by the authors.
References
- Rodeghiero F, Stasi R, Gernsheimer T, et al. Standardization of terminology, definitions and outcome criteria in immune thrombocytopenic purpura of adults and children: report from an international working group. Blood. 2009;113(11):2386–2393. doi: 10.1182/blood-2008-07-162503
- Azizi G, Abolhassani H, Asgardoon MH, et al. Autoimmunity in common variable immunodeficiency: epidemiology, pathophysiology and management. Expert Rev Clin Immunol. 2017;13:101–115. doi: 10.1080/1744666X.2016.1224664
- Sanchez-Ramon S, Radigan L, Yu JE, et al. Memory B cells in common variable immunodeficiency: clinical associations and sex differences. Clin Immunol. 2008;128:314–321. doi: 10.1016/j.clim.2008.02.013
- Arumugakani G, Wood PM, Carter CR. Frequency of Treg cells is reduced in CVID patients with autoimmunity and splenomegaly and is associated with expanded CD21lo B lymphocytes. J Clin Immunol. 2010;30:292–300. doi: 10.1007/s10875-009-9351-3
- Giordano P, Cascioli S, Lassandro G, et al. B-cell hyperfunction in children with immune thrombocytopenic purpura persists after splenectomy. Pediatr Res. 2016;79(2):262–270. doi: 10.1038/pr.2015.211
- Rosser EC, Mauri C. Regulatory B cells: origin, phenotype, and function. Immunity. 2015;42(4):607–612. doi: 10.1016/j.immuni.2015.04.005
- Athanasios M, Theodora S, Areti V, et al. Breg cells are numerically decreased and functionally impaired in patients with systemic sclerosis. Arthritis Rheumatol. 2016;68(2):494–504. doi: 10.1002/art.39437
- Kristensen B, Hegedus L, Lundy SK, et al. Characterization of regulatory B cells in Graves’ disease and Hashimoto’s thyroiditis. PLoS One. 2015;10(5):e0127949. doi: 10.1371/journal.pone.0127949
- Blair PA, Norena LY, Flores-Borja F, et al. CD19 + CD24hiCD38hi b cells exhibit regulatory capacity in healthy individuals but are functionally impaired in systemic lupus erythematosus patients. Immunity. 2010;32(1):129–140. doi: 10.1016/j.immuni.2009.11.009
- Li X, Zhong H, Bao W, et al. Defective regulatory B-cell compartment in patient with immune thrombocytopenia. Blood. 2012;120:3318–3325. doi: 10.1182/blood-2012-05-432575
- Duddy M, Niino M, Adatia F, et al. Distinct effector cytokine profiles of memory and naïve human B cell subsets and implication in multiple sclerosis. J Immunol. 2007;178(10):6092–6099. doi: 10.4049/jimmunol.178.10.6092
- Kim SH, Kim W, Li XF, et al. Repeated treatment with rituximab based on the assessment of peripheral circulating memory B cells in patients with relapsing neuromyelitis optica over 2 years. Arch. Neurol. 2011;68(11):1412–1420. doi: 10.1001/archneurol.2011.154
- Klein U, Rajewsky K, Kuppers R. Human immunoglobulin IgM + IgD+ peripheral blood B cells expressing the CD27 cell surface antigen carry somatically mutated variable region genes: CD27 as ageneral marker for somatically mutated (memory) B cells. J Exp Med. 1998;188:1679–1689. doi: 10.1084/jem.188.9.1679
- Korganow AS, Knapp AM, Nehme-Schuster H, et al. Peripheral B cell abnormalities in patients with systemic lupus erythematous in quiescent phase: decreased memory B cells and membrane CDl9 expression. J Autoimmun. 2010;34(4):426–434. doi: 10.1016/j.jaut.2009.11.002
- Souto-Carneiro MM, Mahadevan V, Takada K, et al. Alterations in peripheral blood memory B cells in patients with active rheumatoid arthritis are dependent on the action of tumour necrosis factor. Arthritis Res Ther. 2009;11(3):R84. doi: 10.1186/ar2718
- Lyu M, Hao Y, Li Y, et al. Upregulation of CD72 expression on CD19 + CD27+ memory B cells by CD40L in primary immune thrombocytopenia. Br J Haematol. 2017;178(2):308–318. doi: 10.1111/bjh.14671
- Mauri C, Menon M. The expanding family of regulatory B cells. Int Immunol. 2015;27(10):479–486. doi: 10.1093/intimm/dxv038
- Sylvain A, Matthieu M, Maxime S, et al. Pathogenesis of immune thrombocytopenia. Autoimmun Rev. 2017;16:620–632. doi: 10.1016/j.autrev.2017.04.012
- Mauri C, Bosma A. Immune regulatory function of B cells. Ann Rev Immunol. 2012;30:221–241. doi: 10.1146/annurev-immunol-020711-074934
- Carter NA, Vasconcellos R, Rosser EC, et al. Mice lacking endogenous IL-10-producing regulatory B cells develop exacerbated disease and present with an increased frequency of Th1/Th17 but a decrease in regulatory T cells. J Immunol. 2011;186(10):5569–5579. doi: 10.4049/jimmunol.1100284
- Qianzi Z, Jung LK. Frequency of CD19 + CD24hiCD38hiregulatory B cell is decreased in peripheral blood and synovial fluid of patients with juvenile idiopathic arthritis: a preliminary study. Pediatr Rheumatol. 2018;16(44):1–9.
- Palomares O, Akdis M, Martín-Fontecha M, et al. Mechanisms of immune regulation in allergic diseases: the role of regulatory T and B cells. Immunol Rev. 2017;278:219–236. doi: 10.1111/imr.12555
- Asmaa M, Sanaa S, Ahmed E, et al. Regulatory and memory B lymphocytes in children with newly diagnosed immune thrombocytopenia. J Hematol. 2017;6(4):81–86. doi: 10.14740/jh336w
- Masson A, Bouaziz JD, Le Buanec H, et al. CD24hiCD27+ and plasma blast-like regulatory B cells in human chronic graft-versus-host disease. Blood. 2015;125(11):1830–1839. doi: 10.1182/blood-2014-09-599159
- Jin L, Weiqian C, Lihuan Y. Peripheral CD24hi CD27+ CD19+ B cells subset as a potential biomarker in naive systemic lupus erythematosus. Int J Rheum Dis. 2013;16:698–708. doi: 10.1111/1756-185X.12229
- Dorner T. Crossroads of B cell activation in autoimmunity: rationale of targeting B cells. J Rheumatol Suppl. 2006;77:3–11.
- Roll P, Palanichamy A, Kneitz, C, et al. Regeneration of B cell subsets after transient B cell depletion using anti-CD20 antibodies in rheumatoid arthritis. Arthritis Rheum. 2006;54(8):2377–2386. doi: 10.1002/art.22019
- Imbach P, Barandun S, d'Apuzzo V, et al. High-dose intravenous gamma globulin for idiopathic thrombocytopenic purpura in childhood. Lancet. 1981;1:1228–1231. doi: 10.1016/S0140-6736(81)92400-4
- Ballow M. Mechanisms of action of intravenous immune serum globulin therapy. Pediatr Infect Dis J. 1994;13:806–811. doi: 10.1097/00006454-199409000-00012
- Labarque V, Van Geet C. Clinical practice: immune thrombocytopenia in paediatrics. Eur J Pediatr. 2014;173:163–172. doi: 10.1007/s00431-013-2254-6
- Kessel A, Ammuri H, Peri R, et al. Intravenous immunoglobulin therapy affects T regulatory cells by increasing their suppressive function. J Immunol. 2007;179:5571–5575. doi: 10.4049/jimmunol.179.8.5571
- Hori A, Fujimura T, Kawamoto S. Anti-inflammatory intravenous immunoglobulin (IVIg) suppresses homeostatic proliferation of B cells. Cytotechnology. 2018;70(3):921–927. doi: 10.1007/s10616-017-0176-2
- Gruver-Yates AL, Quinn MA, Cidlowski JA. Analysis of glucocorticoid receptors and their apoptotic response to dexamethasone in male murine B cells during development. Endocrinology. 2014;155(2):463–474. doi: 10.1210/en.2013-1473
- Kovacs WJ. To B or not to B? Glucocorticoid impact on B lymphocyte fate and function. Endocrinology. 2014;155(2):339–342. doi: 10.1210/en.2013-2085