ABSTRACT
Background
Deficiency in DNA damage response (DDR) pathway and accumulation of DNA damage increases mutation rates resulting in genomic instability and eventually increases the risk of cancer. The aim of our study was to investigate expressions of DNA repair genes as new prognostic biomarkers in acute myeloid leukemia (AML).
Methods
We utilized The Cancer Genome Atlas AML project (TCGA-LAML cohort, 15 acute promyelocytic leukemia (APL) and 155 non-APL AML) for the expression data of DNA repair genes. For validation, clinical samples (Ewha study group, 9 APL and 72 non-APL AML patients) were analyzed for the expression of 22 DNA repair genes using a custom RT2 Profiler PCR Array.
Results
APL patients presented significantly lower expression of DNA repair genes than non-APL AML patients in both study groups. Among non-APL AML patients, high expression levels of PARP1, XRCC1, and RAD51 were associated with poor overall survival (OS) probability in both study groups. Furthermore, Cox regression analysis showed that increased expression levels of PARP1, XRCC1, RAD51, BRCA1 and MRE11A could be independent risk factors for OS in the Ewha study group. Among non-APL patients of the Ewha study group, the OS probability of DDR-overexpressed group with at least one gene or more showing Z score greater than 1.5 was poorer than that of DDR non-overexpressed group.
Conclusion
In the current study, the DNA repair gene expression profile of APL patients was different from that of non-APL AML patients. Overexpression of DNA repair genes could be a poor prognostic biomarker in non-APL AML.
Introduction
The DNA damage response (DDR) pathway is a well-organized network that removes replication errors [Citation1–3]. Genomic instability caused by the malfunction of DDR is considered one of the most important carcinogenic mechanisms [Citation4–9]. One meta-analysis study reported that defective genetic alterations of any component within the DNA repair pathway could increase the risk for the development of leukemias and lymphomas up to 2000 fold [Citation9].
Dysregulation of DDR genes has been reported in various subtypes of acute myeloid leukemia (AML) patients [Citation10–13]. Acute promyelocytic leukemia (APL) has revealed decreased expression of various genes related to the DDR pathway [Citation11,Citation14,Citation15], indicating that genetic defect in DDR may affect the pathogenesis of APL. Other studies have shown that multiple base-excision repair (BER) genes are downregulated in patients with RUNX1-RUNX1T1 rearrangement, whereas DNA polymerase beta (POLB) gene is overexpressed [Citation10,Citation12]. FLT3-ITD mutated cell lines show low levels of Ku proteins verified by high levels of DNA ligase IIIα, which is related to highly error-prone DNA [Citation16]. The presence of DNMT3A and NPM1 mutations may have an influence on DDR, and their emergence can be regarded as key events in leukemogenesis [Citation16]. The silencing of DNA repair genes by gene polymorphisms is also considered a possible mechanism of AML development [Citation11]. RAD51 plays a central role in homologous recombination (HR) to recognize the DSB region by complexing with RAD51B, C, D, XRCC2, and XRCC3. Polymorphic variant RAD51-G135C is reported to correlate with an increased risk of therapy-related AML and an even higher risk when combined with polymorphic variant XRCC3-Thr241Met [Citation11,Citation17,Citation18]. Taken together, the dysregulation of DNA repair genes may be implicated in the pathogenesis of AML.
Comprehensive analysis of expression of DNA repair genes could clarify how genetic defect in DNA repair pathway are clinically involved in AML and confirm the possibility that the expression of DNA repair genes is a new prognostic biomarker for AML. We performed the comprehensive expression analysis of 21 DNA repair genes using data from The Cancer Genome Atlas AML project (TCGA-LAML cohort, https://portal.gdc.cancer.gov). As a validation cohort (Ewha study group), we analyzed gene expression of 22 DNA repair genes in clinical samples. Based on the expression profiles of DNA repair genes, we evaluated the clinical characteristics and prognoses of AML patients.
Methods
Patients
From the TCGA-LAML cohort, the mRNA expression data and patients’ clinical information was obtained using ‘TCGAbiolinks’ R package [Citation19]. Gene expression quantification data (Illumina HiSeq platform) was downloaded from the legacy database (data aligned against the genome of reference hg19) using the GDC application programming interface (API) method.
The TCGA-LAML cohort of de novo 170 AML patients [Citation20] included 15 APL with PML-RARA rearrangement and 155 non-APL AML patients with the gene-expression data of the 21 DNA repair genes of interest (Supplemental Table 1). AML risk group was available on 155 non-APL AML patients in the TCGA-LAML cohort (, 17 in a favorable risk group, 101 in an intermediate risk group, and 37 in an adverse risk group). Survival analysis was performed on 155 non-APL patients (median follow-up period 10.2 months, range: 0.0–95.4 months). RNA-expression profiling on the Affymetrix U133 Plus 2 platform and clinical data were available through the GDC data portal (https://portal.gdc.cancer.gov).
Table 1. Baseline characteristics of AML patients in The Cancer Genome Atlas AML cohort (TCGA-LAML) and the Ewha study group.
The characteristics of 170 AML patients are described in . Gene expression data in the TCGA-LAML cohort was compared as the percentage of GAPDH.
For the validation of the TCGA-LAML cohort, Ewha study group enrolled 81 patients newly diagnosed with AML, including seven therapy-related AML, at our hospital between 2013 and 2018 (, Ewha study group). Nine APL and 72 non-APL AML patients (27 in favorable risk group, 31 in an intermediate risk group, and 14 in adverse risk group based on cytogenetics and molecular abnormalities [Citation21]) were included. The median follow-up period was 42.1 months (range: 0.1–74.1 months). The detailed treatment course in the Ewha study group is shown in Supplemental Figure 1.
In the Ewha study group, to categorize AML patients according to mRNA expression profiles, the Z score method was used. The expression results of each gene were converted to log2 values and then to Z scores. A Z score has a distribution with a mean of 0 and a standard deviation (SD) of 1; hence, 1 Z score means 1 SD [Citation13].
In the Ewha study group, to evaluate the impact of the upregulation of DNA repair genes in AML patients, we analyzed the clinical characteristics and OS probability according to two DDR expression groups. AML patients with at least one gene or more showing Z score greater than 1.5 out of 22 DNA repair genes were assigned to the DDR-overexpressed group, while the remaining patients were included in the DDR-non-overexpressed group.
Electronic medical records were retrospectively reviewed for the clinical and laboratory data in the Ewha Study group.
The present study was approved by the Institutional Review Board of Ewha Womans University, Mokdong Hospital (approval number: EUMC 2018-10-026).
DNA repair gene expression assay in the Ewha study group
We employed a custom RT2 Profiler PCR Array (CLAH29924, Qiagen, CA, USA) that could simultaneously assess the expression of 22 genes associated with DNA repair (Supplemental Table 1). Twenty-two genes comprised three BER pathway genes (APEX1, POLB, and UNG), four nucleotide excision repair (NER) pathway genes (RAD23A, RAD23B, CCNH, and XPC), four mismatch repair (MMR) pathway genes (MLH1, MLH3, MSH2, and PMS2), seven HR pathway genes (BRCA1, BRCA2, RAD50, RAD 51, ATM, POLD3, and MRE11A), and four non-homologous end joining (NHEJ) pathway genes (PARP1, LIG3, XRCC1, and FEN1). Five housekeeping genes (HPRT1, RPLP0, B2M, ACTB, and GAPDH) were included in each set as reference genes for the internal normalization. DNA repair genes in the Ewha Study group (22 genes) was the same as that of TCGA-LAML cohort (21 genes), except MRE11A.
From the bone marrow (BM) aspirates at AML diagnosis, RNA were extracted within 8–12 h of BM collection and stored at −70°C until analysis.
Peripheral bloods (PBs) from five healthy volunteer donors (two males and three females) were used as normal controls for the analysis of fold changes of mRNA expression.
RNA was isolated from the direct BM or PB samples using a QIAamp RNA Blood Mini Kit (Qiagen), and cDNA was synthesized using an RT2 First Strand Kit (Qiagen). The mixture of cDNA with RT2 SYBR Green ROX qPCR Mastermix (Qiagen) was loaded onto the custom RT2 Profiler PCR Array. Quantitative PCR with the RT2 Profiler PCR Array system was performed using the QuantStudio 5 Real-Time PCR Instrument (Thermo Fisher Scientific, MA, USA). All the procedures were carried out according to the manufacturer’s instructions.
The exported Ct values were analyzed through the data analysis web portal (http://www.qiagen.com/geneglobe). The Ct values were converted to fold changes compared to normal PB controls, and the 2−ΔΔCt method was applied for the analysis of fold changes of the AML group.
Cytogenetics and molecular analysis in the Ewha study group
The unstimulated 24–48 short-term cultures using BM aspirates of all patients were analyzed by G-banding. The results were determined according to the International System for Human Cytogenetic Nomenclature (ISCN) 2016 [Citation22]. A complex karyotype was defined as a karyotype with three or more chromosomal abnormalities. To confirm KMT2A rearrangement, an additional FISH analysis was performed using commercially available KMT2A break-apart probes (Abbott/Vysis, IL, USA).
Statistical analysis
In the TCGA-LAML cohort and the Ewha study group, the differences in the expression of DNA repair genes between the AML patients were evaluated using the Wilcoxon–Mann–Whitney test for two groups and the Kruskal–Wallis test with post-hoc Mann–Whitney test for three or more groups.
In the TCGA-LAML cohort and the Ewha study group, the survival was analyzed using the Kaplan–Meier log-rank test for univariate analysis in non-APL AML patients. The cutoff point for each DNA repair gene on the TCGA-LAML cohort was determined by categorizing 146 patients into two groups, such as high expressed and low expressed groups, by changing the proportion of patients with high and low expression. In the Ewha study group, the cutoff point for each DNA repair gene was simulated while changing by 0.1 Z score and determined as the point with the most significant split [Citation23]. In the Ewha study group, using the cutoffs, we performed Cox proportional hazard regression for multivariate analysis in non-APL AML patients.
In the Ewha study group, Pearson’s Chi-square test was used for the analysis of early death rate, complete remission (CR) rate, relapse rate after CR, and the distribution of DDR expression group by age, risk group, and previous chemotherapy history. Early death was defined as death before the initiation of therapy or within 28 days after initiation of therapy.
Analyse-it, 5.01 (Analyse-it Software, Ltd., Leeds, UK), MedCalc Statistical Software version 19.0.3 (MedCalc Software bvba, Ostend, Belgium) and R software ver. 3.3.3 (R Foundation for Statistical Computing, Vienna, Austria. URL http://www.R-project.org/) were used for statistical analysis. P<0.05 was considered statistically significant.
Results
Comparisons of mRNA expression levels of DNA repair genes according to AML subgroup in the TCGA-LAML and the Ewha study group
APL and non-APL
The APL group revealed significantly lower expression of 20 DNA repair genes (except for LIG3 gene), compared to the non-APL AML group in the TCGA-LAML cohort ((A)). By the Ewha study group, lower expressions of BRCA1, RAD51, POLD3, PARP1, RAD23A, MLH1, and MLH3 in APL patients could be validated ((B)). The Ewha study group demonstrated that the APL group tended to show lower expression of BRCA2, RAD50, ATM, and POLB genes, compared to non-APL AML, although not statistically significant.
Figure 1. Relative gene expression of DNA repair genes between APL and non-APL AML, (A) percentage of GAPDH as a comparator using RNA-expression profiling on the Affymetrix U133 Plus 2 platform in The Cancer Genome Atlas AML cohort (TCGA-LAML), and (B) fold changes compared to normal controls using quantitative PCR with an RT2 Profiler PCR array system in the Ewha study group. The horizontal bars represent medians, boxes represent inter-quartile ranges, and dotted lines represent 5th and 95th percentiles. Abbreviations: APL, acute promyelocytic leukemia.
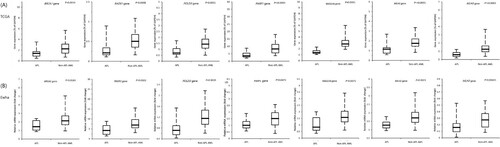
Three risk groups in non-APL AML
Among the three risk groups in non-APL AML patients of the TCGA-LAML cohort, FEN1, RAD23A, XRCC1, and RAD23B in the adverse risk group showed higher expressions than favorable risk group or intermediate risk group ((A)). However, none of the 22 DNA repair genes showed a difference between them in the Ewha study group.
Figure 2. Relative gene expression of RAD23A, RAD23B, FEN1, and XRCC1 DNA repair gene in non-APL AML risk subgroups (A) using the TCGA-LAML cohort and (B) the Ewha study group. Post-hoc comparison was performed among risk groups. The horizontal bars represent medians, boxes represent inter-quartile ranges, and dotted lines represent 5th and 95th percentiles.
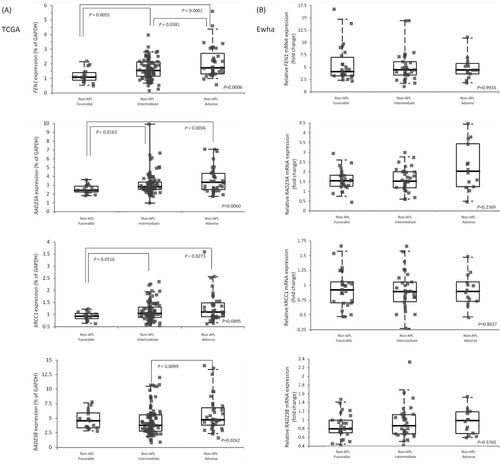
Cytogenetic subgroup in non-APL AML
In the TCGA-LAML cohort, AML with complex karyotype revealed significantly higher expressions of the APEX1, PARP1, RAD23A, RAD23B, MSH2, POLD3, and FEN1 genes than AML with normal karyotype (Supplemental Figure 2A).
Similarly, the Ewha study group showed that RAD23A was significantly highly expressed in AML with complex karyotype than any other subgroups (Supplemental Figure 2B).
In CBF AML of TCGA cohort, FEN1 revealed significantly lower expression than the other groups (Supplemental Figure 2A).
Prognostic impact according to individual mRNA expression levels of DNA repair genes of non-APL AML patients in the TCGA-LAML cohort and the Ewha study group
Through the univariate analysis of the log-rank survival for each DNA repair gene from the TCGA-LAML cohort, we found that patients with higher expression of PARP1, XRCC1, RAD23A, and RAD51 showed poor survival for non-APL AML patients ((A), P=0.0339, 0.0255, 0.0431, and 0.0229, respectively). In the Ewha Study group, high expression of most of the DNA repair genes was validated to be associated with poor survival with different Z score cutoffs ((B) and Supplemental Figure 4). In particular, PARP1, XRCC1, RAD51, BRCA1, and MRE11A showed significantly inferior OS in patients with increased mRNA expression ((B), P=0.0143, 0.0047, 0.0008, 0.0487, and 0.0030, respectively).
Figure 3. Overall survival of non-APL AML patients (A) according to PARP1, XRCC1, RAD51, and RAD23A in the TCGA cohort and (B) PARP1, XRCC1, RAD51, BRCA1 and MRE11A expression in the Ewha study group by Kaplan Meier log rank test.
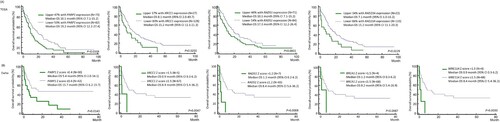
Of particular, in the Ewha Study group, follow up data of 58 non-APL AML patients were available for multivariate analysis to evaluate independent prognostic factors. Age, and risk group were observed to be adverse prognostic factors, while allogeneic hematopoietic stem cell transplantation (allo-HSCT) was to be a favorable prognostic factor (). The genes of PARP1, BRCA1, XRCC1, RAD51, and MRE11A were confirmed as adverse prognostic factors for OS in the Ewha study group ().
Table 2. Multivariate analysis on overall survival of DNA repair genes in non-APL AML patients in the Ewha study group.
Clinical characteristics and outcomes between DDR-overexpressed and DDR-non-overexpressed groups in non-APL AML patients in the Ewha study group
Based on the Z scores of 22 DNA repair genes, there were 35 DDR-overexpressed patients and 46 DDR-non-overexpressed patients. The proportion of patients with DDR overexpression varies significantly with age group, indicating that the incidence of DDR-overexpressed patients is increasing with age (Supplemental Figure 3A, P=0.0147). However, the proportions of the DDR-overexpressed patients were not different according to risk group, and previous chemotherapy (Supplemental Figure 3B and 3C, P=0.5155 and 0.1331, respectively). There were no differences in WBC count, hemoglobin, platelet count or BM blast percentage between the two groups (P>0.05, Supplemental Table 2).
On univariate analysis of OS in AML patients based on risk groups, the APL group showed the best survival followed by the risk groups sequentially ((A), P=0.0288). Since we found that APL patients showed lower expression values in various genes of the DDR pathway and superior survival among the risk groups, we analyzed the OS of AML patients excluding APL patients.
Figure 4. Kaplan Meier survival curves of (A) according to the risk groups among all AML patients, and (B) based on the expression levels of DNA repair genes among non-APL AML patients in the Ewha study group. * Patients with at least one gene or more showing Z-scores greater than 1.5. † The remaining patients. Abbreviations: APL, acute promyelocytic leukemia.
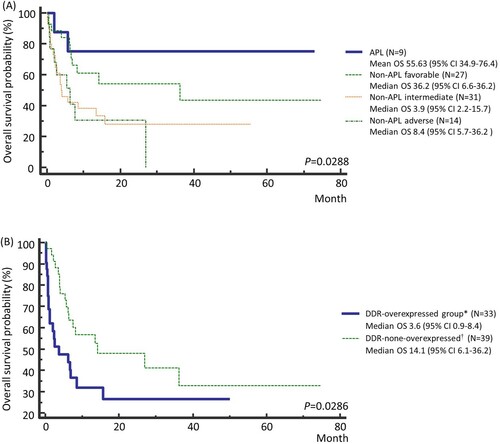
The DDR-overexpressed group showed poorer survival than the DDR non-overexpressed group in non-APL AML patients ((B), P=0.0286). Early death rate in the DDR-overexpressed group was significantly higher than in the DDR-non-overexpressed group (, P=0.0012), while the CR rate was significantly lower in the DDR-overexpressed group than in the DDR-non-overexpressed group (, P=0.0378). Analysis on outcomes based on risk groups showed statistically significant difference only in CR rate (, P=0.0222).
Table 3. Outcomes of non-APL AML according to the AML risk group and DNA damage response Expression group in the Ewha study group.
Among 63 non-APL AML patients who underwent chemotherapy, the patients treated with the standard intensive chemotherapy showed the most favorable OS, while patients treated with the low-intensity chemotherapy had the worst OS (Supplemental Figure 5A, P=0.0152). Among the patients treated with the abbreviated-scheduled chemotherapy, the DDR-overexpressed group tended to have inferior OS than the DDR-non-overexpressed group (Supplemental Figure 5C, P=0.0503). The DDR-overexpressed patients who received allo-HSCT showed the best OS than other subgroups (Supplemental Figure 6).
Discussion
In both the TCGA-LAML cohort and Ewha study group, the expression of DNA repair genes was significantly lower in APL patients than in non-APL AML patients. As for prognostic impact in non-APL AML patients, the TCGA-LAML cohort demonstrated that overexpression of PARP1, XRCC1, RAD23A, and RAD51 was associated with poor survival. Among non-APL patients of the Ewha study group, the increased expression levels of PARP1, BRCA1, XRCC1, RAD51, and MRE11A with each different Z score cutoff were independent factors of poor OS prognosis. The OS probability of the DDR-overexpressed group with at least one gene or more showing Z score greater than 1.5 was poorer than that of the DDR-non-overexpressed group.
In APL, the PML-RARA protein has been reported to disrupt PML nuclear bodies, leading to impaired DDR through repressing the BER, HR, and NHEJ pathways [Citation11,Citation14,Citation15,Citation24–28]. Regarding non-APL AML patients, a few studies have shown that RUNX1/RUNX1T1 fusion oncoprotein could suppress the HR pathway (BRCA1, BRCA2, and/or KU70 protein) (13, 14, 29). In contrast, AML driven by KMT2A fusions could be proficient in DDR, and it may be caused by the fact that HOXA9 (a key target of KMT2A fusions) can promote the expression of various HR-associated genes [Citation14,Citation29]. Consistent with previous studies, the TCGA-LAML cohort and the Ewha study group validated that APL patients showed significantly lower expression of DNA repair genes in multiple pathways of DDR compared to non-APL AML patients.
Concerning karyotypic subgroups in this study, higher expression of the APEX1, PARP1, RAD23A, RAD23B, MSH2, POLD3, and FEN1 genes was observed in patients with complex karyotype, while AML with normal karyotype showed more downregulation of RAD23B than the other karyotypic subgroups (Supplemental Figure 2). Although the exact roles of RAD23A and RAD23B have not yet been elucidated in AML, RAD23 complexed with XPC recognizes UV-induced DNA distortion and leads to successive DNA repair through the NER pathway [Citation30,Citation31]. According to one study, upregulation of DNA repair genes associated with DSB repair and cell cycle checkpoint signaling genes was observed in AML with a complex karyotype [Citation17]. Another study showed that PARP1 and LIG3 were upregulated in patients with chromosomal translocation [Citation32]. Taken together, increased DNA repair gene expression could be a distinct characteristic in AML with a complex karyotype [Citation17,Citation33,Citation34].
Upregulated DNA repair genes in AML patients may allow error-prone DNA to repair in cancer cells, leading to its continuous survival, and may exert resistance to chemotherapy [Citation11,Citation17]. The present study showed that the overexpression of DNA repair genes in non-APL AML patients was associated with poor prognosis, whereas decreased DNA repair gene expression was observed in APL patients with a good prognosis. The poor prognosis in the DDR-overexpressed group can be partially explained by higher early death rate and lower CR rate than that in the non-overexpressed group (). Similar to our study, increased PARP1 mRNA levels have previously shown negative correlations with prognosis in AML [Citation32,Citation34–37]. The RUNX1/RUNX1T1 group subgrouped by higher expression of BRCA1, RAD51, or CHK2 genes showed the worst prognosis and poor OS [Citation11,Citation38]. Another study showed that the upregulation of RAD51 was associated with relapse and drug-resistance in AML with FLT3-ITD/TKD mutation [Citation11]. Taken together, whereas compromised DDR may induce leukemogenesis by accumulating DNA damage, upregulated DDR can make AML cells to escape DNA repair mechanisms and lead to resistance to chemotherapy as a result of the increased ability of AML cells to repair damaged DNA lesions [Citation11]. In that prognosis is improved when receiving allo-HSCT in the overexpressed group of patients (Supplemental Figure 6), the Allo-HSCT might be one option to overcome DDR overexpression.
Concerning the kinds of upregulated DNA repair genes, the present study demonstrated that overexpression of PARP1, BRCA1, XRCC1, RAD51, and MRE11A presented a negative correlation with prognosis in non-APL AML patients. Related genes were not confined to one specific mechanism in the DNA repair pathway but were involved in various mechanisms (HR, NER, MMR, etc.), indicating that DDR may be heterogeneously impaired in AML. One study showed that patients with adverse cytogenetic risk had higher PARP1 expression than other cytogenetic risk groups in non-APL AML [Citation37]. Another study showed that a PARP1-high-expression group showed more frequent FLT3-ITD mutation [Citation36]. High PARP1 expression predicted poor survival in AML patients with normal karyotype [Citation36]. Similar to AML, PARP1 was an adverse prognostic marker for OS in patients with low to intermediate-1 risk MDS according to the international prognostic scoring system [Citation35].
Chemotherapy agents generate more DNA damage in AML cells and probably upregulate the PARP1 gene. PARP1 stimulates BER for SSB and facilitates the MRE-11-mediated recruitment of RAD51, a DNA damage marker, to recognize DSB, hence it prevents the accumulation of potentially lethal DSBs [Citation13]. The dysregulated DNA repairing activity resulting from PARP1 overexpression in AML cells may induce anti-apoptosis of AML blasts, which results in a poor response to chemotherapy. Therefore, the inhibition of PARP1 might be an effective option to overcome chemoresistance in AML.
PARP inhibitor (PARPi) can induce synthetic lethality, which kills cells with defects in the repair of DSBs via the HR mechanism [Citation1,Citation3,Citation39,Citation40,Citation41]. In previous studies, AML with lower expression of BRCA1 mRNA level was sensitive to PARPi, whereas AML with higher expression of PARP1 consistently showed resistance to PARPi [Citation33,Citation34]. AML cells with low expression of DNA repair genes, including RAD51, ATM, BRCA1, and BRCA2, displays the extreme response to PARPi [Citation36]. A few reports have demonstrated that RUNX1/RUNX1T1 or PML/RARA fusion oncoproteins are extremely sensitive to PARP inhibition, partly caused by their suppression of HR gene expression and their altered DDR [Citation14,Citation29]. These findings indicate that the DDR pathways could be an ideal target for AML treatment.
TCGA-LAML cohort and Ewha study group showed almost the same result in the mRNA expression and OS probability. There were some differences in the results. Those different results between two study groups may be caused by the difference in the characteristics of the patient groups. The TCGA-LAML cohort contains 170 de novo AML, whereas the Ewha study group comprises 91% of de novo AML and 9% of therapy-related AML (). Unlike the TCGA-LAML cohort, the Ewha study group included a larger proportion of non-APL favorable risk group and less non-APL adverse risk group (). Relatively smaller sample size in the Ewha study group may not be enough to prove statistical significance. It should also be noted that the two experiments were conducted using different test methods; RNA-expression profiling using next generation sequencing in the TCGA-LAML cohort and quantitative mRNA PCR in the Ewha study group.
Conclusions
This study demonstrated that the DNA repair gene expression profile of APL patients was different from that of non-APL AML patients, showing lower expression of DNA repair genes. Overexpression of DNA repair genes such as PARP1, BRCA1, XRCC1, RAD51, and MRE11A could be one of the markers of poor prognosis in non-APL AML patients. These findings should be evaluated further in larger cohorts to be implemented in clinical practice.
Supplemental Material
Download MS Word (3.4 MB)Supplemental Material
Download MS Word (32.1 KB)Disclosure statement
No potential conflict of interest was reported by the author(s).
Data availability statement
The TCGA-LAML datasets analyzed for this study can be found in the GDC data portal (https://portal.gdc.cancer.gov). The datasets generated and analyzed of Ewha study group are not publicly available.
Additional information
Funding
References
- Cerrato A, Morra F, Celetti A. Use of poly ADP-ribose polymerase [PARP] inhibitors in cancer cells bearing DDR defects: the rationale for their inclusion in the clinic. J Exp Clin Cancer Res. 2016;35:179.
- Lim D, Ngeow J. Evaluation of the methods to identify patients who may benefit from PARP inhibitor use. Endocr Relat Cancer. 2016;23:R267–R285.
- Shaheen M, Allen C, Nickoloff JA, et al. Synthetic lethality: exploiting the addiction of cancer to DNA repair. Blood. 2011;117:6074–6082.
- Brose MS, Rebbeck TR, Calzone KA, et al. Cancer risk estimates for BRCA1 mutation carriers identified in a risk evaluation program. J Natl Cancer Inst. 2002;94:1365–1372.
- Hanahan D, Weinberg RA. The hallmarks of cancer. Cell. 2000;100:57–70.
- Hanahan D, Weinberg RA. Hallmarks of cancer: the next generation. Cell. 2011;144:646–674.
- King MC, Marks JH, Mandell JB. New York breast cancer study G. breast and ovarian cancer risks due to inherited mutations in BRCA1 and BRCA2. Science. 2003;302:643–646.
- Shiovitz S, Korde LA. Genetics of breast cancer: a topic in evolution. Ann Oncol. 2015;26:1291–1299.
- Friedenson B. The BRCA1/2 pathway prevents hematologic cancers in addition to breast and ovarian cancers. BMC Cancer. 2007;7:152.
- Alcalay M, Meani N, Gelmetti V, et al. Acute myeloid leukemia fusion proteins deregulate genes involved in stem cell maintenance and DNA repair. J Clin Invest. 2003;112:1751–1761.
- Esposito MT, So CW. DNA damage accumulation and repair defects in acute myeloid leukemia: implications for pathogenesis, disease progression, and chemotherapy resistance. Chromosoma. 2014;123:545–561.
- Krejci O, Wunderlich M, Geiger H, et al. P53 signaling in response to increased DNA damage sensitizes AML1-ETO cells to stress-induced death. Blood. 2008;111:2190–2199.
- Nieborowska-Skorska M, Sullivan K, Dasgupta Y, et al. Gene expression and mutation-guided synthetic lethality eradicates proliferating and quiescent leukemia cells. J Clin Invest. 2017;127:2392–2406.
- Esposito MT, Zhao L, Fung TK, et al. Synthetic lethal targeting of oncogenic transcription factors in acute leukemia by PARP inhibitors. Nat Med. 2015;21:1481–1490.
- Zhao L, So CW. PARP-inhibitor-induced synthetic lethality for acute myeloid leukemia treatment. Exp Hematol. 2016;44:902–907.
- Gafencu GA, Tomuleasa CI, Ghiaur G. PARP inhibitors in acute myeloid leukaemia therapy: how a synthetic lethality approach can be a valid therapeutic alternative. Med Hypotheses. 2017;104:30–34.
- Schoch C, Kern W, Kohlmann A, et al. Acute myeloid leukemia with a complex aberrant karyotype is a distinct biological entity characterized by genomic imbalances and a specific gene expression profile. Genes Chromosomes Cancer. 2005;43:227–238.
- Seedhouse C, Faulkner R, Ashraf N, et al. Polymorphisms in genes involved in homologous recombination repair interact to increase the risk of developing acute myeloid leukemia. Clin Cancer Res. 2004;10:2675–2680.
- Colaprico A, Silva TC, Olsen C, et al. TCGAbiolinks: an R/bioconductor package for integrative analysis of TCGA data. Nucleic Acids Res. 2016;44:e71.
- Cancer Genome Atlas Research Network, Ley TJ, Miller C, Ding L, et al. Genomic and epigenomic landscapes of adult de novo acute myeloid leukemia. N Engl J Med. 2013;368:2059–2074.
- Dohner H, Estey E, Grimwade D, et al. Diagnosis and management of AML in adults: 2017 ELN recommendations from an international expert panel. Blood. 2017;129:424–447.
- McGowan-Jordan JSA, Schmid M. ISCN 2016: An international system for human cytogenetic nomenclature (2016). Basel (Switzland): Karger; 2016.
- Budczies J, Klauschen F, Sinn BV, et al. Cutoff finder: a comprehensive and straightforward Web application enabling rapid biomarker cutoff optimization. PLoS One. 2012;7:e51862.
- Chang HR, Munkhjargal A, Kim MJ, et al. The functional roles of PML nuclear bodies in genome maintenance. Mutat Res. 2018;809:99–107.
- di Masi A, Cilli D, Berardinelli F, et al. PML nuclear body disruption impairs DNA double-strand break sensing and repair in APL. Cell Death Dis. 2016;7:e2308.
- Tallman MS, Nabhan C, Feusner JH, et al. Acute promyelocytic leukemia: evolving therapeutic strategies. Blood. 2002;99:759–767.
- Virag L, Szabo C. The therapeutic potential of poly(ADP-ribose) polymerase inhibitors. Pharmacol Rev. 2002;54:375–429.
- Voisset E, Moravcsik E, Stratford EW, et al. Pml nuclear body disruption cooperates in APL pathogenesis and impairs DNA damage repair pathways in mice. Blood. 2018;131:636–648.
- Zhao L, So CWE. PARPi potentiates with current conventional therapy in MLL leukemia. Cell Cycle. 2017;16:1861–1869.
- Chen L, Madura K. Evidence for distinct functions for human DNA repair factors hHR23A and hHR23B. FEBS Lett. 2006;580:3401–3408.
- Dantuma NP, Heinen C, Hoogstraten D. The ubiquitin receptor Rad23: at the crossroads of nucleotide excision repair and proteasomal degradation. DNA Repair (Amst. 2009;8:449–460.
- Pashaiefar H, Yaghmaie M, Tavakkoly-Bazzaz J, et al. The association between PARP1 and LIG3 expression levels and chromosomal translocations in acute myeloid leukemia patients. Cell J. 2018;20:204–210.
- Faraoni I, Compagnone M, Lavorgna S, et al. BRCA1, PARP1 and gammaH2AX in acute myeloid leukemia: role as biomarkers of response to the PARP inhibitor olaparib. Biochim Biophys Acta. 2015;1852:462–472.
- Wang L, Cai W, Zhang W, et al. Inhibition of poly(ADP-ribose) polymerase 1 protects against acute myeloid leukemia by suppressing the myeloproliferative leukemia virus oncogene. Oncotarget. 2015;6:27490–27504.
- Diamantopoulos P, Zervakis K, Zervakis P, et al. Poly (ADP-ribose) polymerase 1 mRNA levels strongly correlate with the prognosis of myelodysplastic syndromes. Blood Cancer J. 2017;7:e533.
- Li X, Li C, Jin J, et al. High PARP-1 expression predicts poor survival in acute myeloid leukemia and PARP-1 inhibitor and SAHA-bendamustine hybrid inhibitor combination treatment synergistically enhances anti-tumor effects. EBioMedicine. 2018;38:47–56.
- Pashaiefar H, Yaghmaie M, Tavakkoly-Bazzaz J, et al. PARP-1 Overexpression as an independent prognostic factor in adult Non-M3 acute myeloid leukemia. Genet Test Mol Biomarkers. 2018;22:343–349.
- Bullinger L, Rucker FG, Kurz S, et al. Gene-expression profiling identifies distinct subclasses of core binding factor acute myeloid leukemia. Blood. 2007;110:1291–1300.
- Curtin NJ. DNA repair dysregulation from cancer driver to therapeutic target. Nat Rev Cancer. 2012;12:801–817.
- Farmer H, McCabe N, Lord CJ, et al. Targeting the DNA repair defect in BRCA mutant cells as a therapeutic strategy. Nature. 2005;434:917–921.
- Gaymes TJ, Shall S, Farzaneh F, et al. Chromosomal instability syndromes are sensitive to poly ADP-ribose polymerase inhibitors. Haematologica. 2008;93:1886–1889.