ABSTRACT
Objective
The currently employed red blood cell reagents have a short shelf life. Some hospitals with a small number of specimens will be unable to utilize them within the validity period, resulting in a substantial increase in the purchase price. Therefore, the method of developing long-term red blood cell reagents is a problem worthy of further study.
Methods
In this experiment, the type and concentration of the red blood cell reagent treatment solution were evaluated based on the red blood cell antigen concentration 24 h after treatment. In addition, the qualified glutaraldehyde/paraformaldehyde reagent was stored for six months, and five red blood cell indices were measured every month. At the same time, the detection indices of treated red blood cell reagents and untreated red blood cell reagents were compared.
Results
It was discovered that treated red blood cells containing 0.005% GA and 0.05% PFA were more suitable for the preservation of red blood cells than other treated concentrations, and the preservation time could reach six months. The test tube method (n = 24) and microcolumn gel card (n = 35) were used to determine the accuracy of the treated blood cells containing 0.005% glutaraldehyde +0.05% paraformaldehyde, with an accuracy of 100%.
Conclusion
This experiment resulted in the development of a novel reagent for treating red blood cells with glutaraldehyde/paraformaldehyde fixed solution that can effectively prolong its storage time by two to three times that of red blood cell reagents currently on the market.
1. Introduction
When the blood types of blood donors and recipients are incompatible, such as when the red blood cells of type B blood donors are transfused into patients with type A blood, the anti -A antibodies of type B blood donors will also enter the patients and destroy the red blood cells, which can cause acute or delayed blood transfusion reactions and, in extreme cases, threaten the health and life of patients. Consequently, blood type identification prior to transfusion is crucial [Citation1–4]. The British Pharmacopoeia highlights the significance of results of ‘forward type’ and ‘reverse type’ prior to blood transfusion [Citation5]. The cross-matching and antibody screening experiment can only be conducted when the results of ‘forward type’ and ‘reverse type’ are consistent. The term ‘forward type’ refers to the detection of A/B antigens on the surface of RBCs(red blood cells), whereas ‘reverse type’ refers to the detection of anti-A/B antibodies in plasma using RBC reagent [Citation6,Citation7]. Red blood cell reagent is an anti-type detection reagent used to identify blood groups.
Red blood cell reagents for reverse typing detection are typically preserved using low temperature storage, freeze-drying, and storage at 4°C [Citation8]. However, each of the three methods listed above has flaws [Citation9–13]. Low-temperature freezing (typically −80°C) requires an ultra-low-temperature refrigerator or a liquid nitrogen tank, which consumes a significant amount of liquid nitrogen or electricity and is challenging to transport [Citation14–16]. The process of rewarming and washing to remove cryoprotectants (typically high-concentration glycerin) is complex, time-consuming, and laborious, and it is difficult to ensure that cryoprotectants can be added and removed without excessive red blood cell loss at a reasonable cost and in a reasonable amount of time [Citation17]. Freeze-drying requires the use of specialized freeze-drying equipment and a freeze-drying protective agent, as well as a labor-intensive and time-consuming operation process [Citation18,Citation19]. Preserving commercial red blood cell reagents at 4°C is a common method that is utilized in clinical practice. However, to date, the shelf life of commercial red blood cell reagents used in ABO reverse typing tests is only 2–3 months under 4°C. After more than three months, commercial red blood cell reagents become hemolyzed easily, and the antigen stability on the surface of commercial red blood cell reagents is poor, which is not conducive to ABO reverse typing test results.
The primary factors affecting RBC storage were repeated freezing and thawing cycles, pH, RBC storage solution, NO, and chemical modification [Citation8,Citation16]. Two fixatives, GA and PFA, were used in our laboratory to modify RBCs, extending their storage time from 2 to 3 months to 6–7 months. Fixatives are classified into two types: cross-linking and denaturation [Citation20,Citation21]. In the laboratory, we treated RBCs using cross-linking fixatives [Citation22,Citation23]. Combining GA and PFA to fix RBCs compensates for the shortcomings of a single application and achieves perfect fixation [Citation24]. GA is a linear 5-carbon dialdehyde that can react with the amino group of proteins in normal saline solution [Citation25] and maintain the protein's stable structure; however, it is slow and takes a long time to fix [Citation26]. Although PFA rapidly crosslinks with surrounding proteins via aldehyde groups to form a methylene bridge complex that preserves the structure of the protein, this process is insufficient to prevent protein redistribution and slight destruction of the cell membrane structure [Citation27]. Combining GA and PFA compensates for their shortcomings [Citation28,Citation29]. In addition, when GA and PFA interact with red blood cell reagents, the volume of red blood cells increases, and their deformability decreases [Citation30], making it difficult for GA/PFA treated red blood cell reagents to pass through the pores of the microcolumn gel cards. The purpose of this study is to develop a novel reverse typing red blood cell reagent that can be stored for 6 months and has high accuracy, and then apply it to the reverse typing test of clinical patients prior to blood transfusion to ensure the safety and efficacy of blood transfusion.
2. Materials and methods
2.1. Blood samples, materials, and instruments
The blood transfusion department of the China–Japan Union Hospital of Jilin University provided whole blood and plasma samples. Blood samples were collected from drug-free, 16-to-55-year-old adults of both sexes who are in good health. Plasma samples were collected at random from patients requiring blood transfusions around the globe, the majority of whom were Han. There are no age, sex, or drug restrictions. The Ethics Committee of the China–Japan Union Hospital of Jilin University approved this ethical review (approval number:20220425010). Guoyao Company provided 25% pure GA and 4% pure PFA. GA and PFA were sterile filtered before use. Normal saline was a self-made 0.9% sodium chloride solution that had a pH between 7.2 and 7.4 and was sterilized by high-pressure steam. The ultraviolet spectrophotometer (Hitachi) signal is U-3900H. TD-A medical centrifuge was purchased from Changchun Boyan Scientific Instrument Co., Ltd.
2.2. Study design
2.2.1. Preparation of a solution treated with GA and PFA
GA was diluted to 0.01% by mass with normal saline and diluted with normal saline until the mass fraction was 0.1%. GA and PFA were mixed in a volume ratio of 1:1 to obtain GA with a mass fraction of 0.005% in normal saline and PFA with a mass fraction of 0.05% in normal saline (stored at 4°C).
2.2.2. Determination of GA/PFA treated solution concentration and type
In this experiment, the types and concentrations of the GA/PFA treated solutions were altered to produce solutions with different concentrations and types. Different concentrations of GA (0.2%, 0.18%, 0.16%, 0.14%, 0.12%, 0.1%, 0.04%, 0.03%, 0.02%, 0.01%) and solution containing varying concentrations of GA and PFA (GA+PFA: 0.05%+0.2%, 0.04%+0.2%, 0.03%+0.2%, 0.02%+0.2%, 0.01%+0.2%, 0.01%+0.05%, 0.02%+0.1%, 0.02%+0.05%, 0.005%+0.1%, 0.005+0.05%) were tested. The antigen intensity of 0.1% GA+0.005% PFA and 0.005% GA+0.05% PFA was detected using the tube method within 24 h, and the result was 4+, which met the clinical standard. However, the antigen intensity of red blood cells treated by other GA/PFA treated solutions did not reach 2+ within 24 h using the test tube method. Finally, the red blood cells were treated with 0.1% GA+0.005% PFA and 0.005% GA+0.05% PFA stationary solutions, and their storage time was observed. Simultaneously, the experiment of treating red blood cells with 0.02%GA+0.05%PFA was added to determine the antigen intensity, dispersibility, appearance, free hemoglobin concentration in supernatant, and accuracy of red blood cells treated with various GA/PFA concentrations for 6 months.
2.2.3. Determination of the time required for a GA/PFA liquid to treat red blood cell reagent
Red blood cells were treated with a GA/PFA treated solution for varying amounts of time (5, 10, and 20 min), and the resulting effects were observed. When the GA/PFA treated solution reacted with red blood cells for 5 min, the supernatant was poured after the next centrifugation, and it was observed that the red blood cells flowed into the test tube mouth with the supernatant. This effect was identical to that of fresh red blood cells that were not treated with the GA/PFA treated solution. However, when the GA/PFA treated solution reacts with red blood cells for 10 or 20 min, the red blood cells will not flow with the supernatant but will instead deposit at the bottom of the tube, indicating that the GA/PFA treated solution has an obvious effect on red blood cells. As 10 min is shorter than 20 min, saving time during product preparation, the action time of the GA/PFA treated solution on red blood cells was set to 10 min.
2.2.4. Specific steps of red blood cell treatment with glutaraldehyde/ paraformaldehyde
Fresh, healthy adult red blood cells anticoagulated with sodium citrate were subjected to microcolumn gel card testing for ‘forward type’ and ‘reverse type’ to confirm the accuracy of blood type. Red blood cells of types A and B were washed three times with physiological saline, centrifuged at 1200 rpm for 5 min each time, and red cell pellet was collected after the final centrifugation. To obtain erythrocytes with a mass fraction of 10%, the red cell pellet was diluted with physiological saline. Afterwards, a 1:1 mixture of GA and PFA was added to a 10% red blood cell solution, which was blended for 10 min at room temperature (25°C). Currently, GA and PFA concentrations are 0.005% and 0.05%, respectively. Then washed with normal saline four 4 times (1200 rpm for 5 min), and the hematocrit was retained. The hematocrit was diluted evenly to a concentration of 5% and a volume of 3 mL by adding red blood cell preservation solution to sterilized 10 mL plastic test tubes. For sealing, parafilm was utilized. Using red blood cell preservation reagent, dilute the fresh hematocrit to 5% concentration (volume is 3 mL) after three washings. Finally, the sealed 5% GA/PFA-treated red blood cell reagent and the sealed 5% chemically untreated red blood cell reagent were stored at 4°C and red blood cell indexes were determined. The storage and utilization conditions for both are identical.
2.2.5. Preparation of red blood cell preserving solution
In our laboratory, red blood cell preservation reagents are prepared using water that has been sterilized by steam under high pressure. The primary components of red blood cell preservation reagent include trisodium citrate, sodium chloride, glucose, sucrose, adenine, creatinine, kanamycin, chloramphenicol, sodium azide, and others. Glucose is an energy source, adenine can prolong the storage time of red blood cells at 4°C, and sodium azide inhibits the reproduction of bacteria and other microorganisms.
2.2.6. Detection indicators for RBCs
In this experiment, the preservation ability of GA/PFA treated solutions was evaluated based on the related indices of red blood cell reagents, such as antigen potency, antibody specificity, free hemoglobin in the supernatant, preservation appearance, dispersibility, and precision.
2.2.6.1. Antigen intensity of red blood cell reagent
In this experiment, the antigen intensity of the erythrocyte reagent was confirmed using the test tube and microcolumn gel methods, respectively. The agglutination strength of red blood cell reagent and antibody reagent verified the antigen intensity of red blood cell reagent. The microcolumn gel method primarily verified the viability of 0.005% glutaraldehyde /0.05% paraformaldehyde to treat red blood cell reagents via the clarity of the microcolumn gel card's background. After red blood cells were treated with glutaraldehyde and paraformaldehyde, their volume increases, and after centrifugation, it was difficult for red blood cells to deposit on the bottom of a microcolumn gel card through the gel's pores. This experiment compared the background clarity of microcolumn gel cards after detecting glutaraldehyde-treated and untreated erythrocyte reagents using the microcolumn gel method. Tables S1 and S2 of the Supplementary Materials provide agglutination strength interpretation standards for the test tube and microcolumn gel techniques.
2.2.6.2. Hemolysis of red blood cell
2.2.6.2.1. Appearance detection of red blood cell reagent
At the seventh month of the preliminary experiment, the appearance of red blood cell reagent was observed, and it was discovered that the red blood cell reagent treated with glutaraldehyde/paraformaldehyde was hemolyzed and the color of the supernatant turned red. This experiment evaluated the hemolysis of red blood cell reagent by observing and recording the color of the red blood cell reagent supernatant after 24 h and 6 months, as well as the stratification between the supernatant and packed red blood cells.
2.2.6.2.2. Detection of free hemoglobin in supernatant
The 5% glutaraldehyde/paraformaldehyde treated red blood cell reagent and the 5% untreated red blood cell reagent were sealed and stored at 4°C. The supernatant was removed from a plastic tube every other month to determine the content of free hemoglobin in the supernatant by colorimetry. The remaining hematocrit red blood cells were evaluated for additional markers. The supernatant was treated in strict accordance with the Nanjing jiancheng hemoglobin detection kit (colorimetric method). Lastly, Graphpad Prism9.4.1 was utilized to statistically analyze the three experimental data and generate a line graph.
2.2.6.3. Red blood cell reagent for detection of antibody titration
To detect the alteration in erythrocyte antigen activity, the antibody titer of erythrocyte reagent was measured. Anti-A and anti-B antibody reagents were diluted with normal saline, resulting in anti-A and anti-B antibodies with various dilutions of 1:2, 1:4, 1:8, 1:16, 1:32, 1:64, 1:128, 1:256, and 1:512. React 100 L of antibody with each dilution of 100 L of 4% red blood cell reagent, centrifuge for 1 min at 1000 rpm, and record the reaction intensity.
2.2.6.4. Detection of erythrocyte specificity
Using anti -A reagent (purchased from Shanghai Hematology), anti -B reagent (purchased from Shanghai Hematology), anti -AB reagent (purchased from Shanghai Hematology), human A red blood cell plasma, human B red blood cell plasma, human O red blood cell plasma, human AB red blood cell plasma (All plasma is supplied by the China–Japan Union Hospital of Jilin University), the specificity of red blood cell reagent was determined using the test tube method.
2.2.6.5. Red blood cell dispersion detection
In this experiment, the dispersion of red blood cell reagents treated with glutaraldehyde/paraformaldehyde, untreated red blood cell reagents, and fresh red blood cells stored for 24 h was observed under a low-power microscope to determine whether there was agglutination or clustering of 2–3 cells.
2.2.7. Red blood cell precision examination
To evaluate the accuracy of glutaraldehyde/paraformaldehyde treated red blood cell reagents and untreated red blood cell reagents stored for 6 months, plasma samples were detected by storing red blood cell reagents for 6 months, untreated red blood cell reagents, and fresh red blood cells within 24 h. The majority of the experiment was conducted using the microcolumn gel method and the test tube method. Because the test tube method is the gold standard for clinical blood group identification, fresh red cells are used with the test tube method to determine the blood type of plasma samples. Tables S1 and S2 of the Supplementary Materials provide agglutination strength interpretation standards for the test tube and microcolumn gel techniques.
3. Results
3.1. Red blood cell reagent antigen intensity
3.1.1. The test tube method for determining the antigen intensity of red blood cell reagent
The chart depicts the antigen intensity changes of type-A RBC detected in the test tubes over time and under various modification conditions. The antigen intensity of 0.2% GA+0.05% PFA treated RBC was 2+; in the third month, the antigen intensity of 0.1% GA+0.05% PFA treated RBC was 2+, which did not meet the clinical application standards. Within the range of permissible error, the antigen intensity of type-B RBC detected at various time points and under various modification conditions was essentially identical to that of type-A RBC (). Within 6 months, the antigen intensity of red blood cells treated with 0.005% glutaraldehyde /0.05% paraformaldehyde is equivalent to the antigen intensity of untreated red blood cells; both are 4+.
Figure 1. Test tube method for detecting the antigen intensity of GA/PFA treated red blood cells with different concentrations. At six months, the antigen intensity of red blood cells treated with 0.005% GA and 0.05% PFA was 4+; the antigen intensity of 0.2% GA+0.05% PFA treated RBCs was 2+ within 24 h; in the third month, the antigen intensity of 0.1% GA+0.05% PFA treated RBCs was 2+, which did not meet clinical application standards.
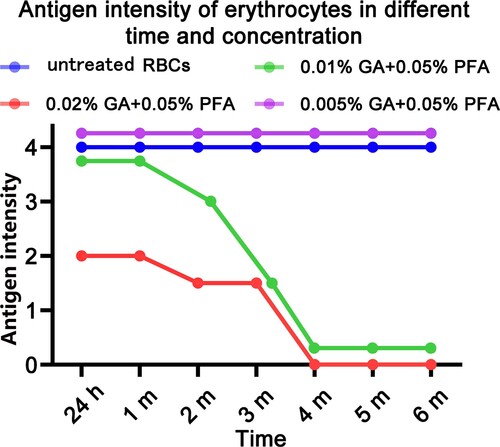
3.1.2. Detecting the antigen intensity of red blood cell reagent with antibody reagent and plasma using the microcolumn gel method
3.1.2.1. Antibody reagent
demonstrated that both the positive result (red blood cells collected at the top of the microcolumn gel card) and the negative result (red blood cells collected at the bottom of the microcolumn gel card) were acceptable, and there was no cause for concern. Red blood cells of type A had an agglutinate in the presence of anti-A antibody reagent, but not anti-B antibody reagent. Red blood cells of type B had an agglutinate with anti-B antibody reagent, but not with anti-A antibody reagent. Compared to the results of the first month, red blood cells were treated with 0.02% GA /0.05% PFA and 0.01% GA /0.05% PFA in the sixth month. After centrifugation, red particles were observed in the column, and the negative background of the microcolumn gel card was manifestly obscured. Red blood cells were treated with 0.005% glutaraldehyde /0.05% paraformaldehyde; the negative background in the microcolumn gel card was clear, and there were no red particles in the column, which was consistent with the untreated red blood cells reagent. The negative context was obscure. After centrifugation, the presence of red particles in the column was primarily attributable to the increase in red blood cell volume and decrease in deformability caused by glutaraldehyde and paraformaldehyde fixation of red blood cells, and the inability of the treated red blood cells to pass through the microcolumn's gel pores. The above results showed that the new reagent developed by treating red blood cells with 0.005% GA/0.05% PFA can replace the commercial red blood cell reagent for clinical reverse typing detection.
Figure 2. The results of testing a GA/PFA treated red blood cell reagent against an antibody reagent using a microcolumn gel card (Anti-A antibody reagent is contained in the blue gel tubes, whereas anti-B antibody reagent is contained in the yellow gel tubes). In the card test results for the 0.005% GA+0.05% PFA treated red blood cell reagent at the first and sixth months, the negative background was evident and the antigen intensity was 4+. In the first month, the negative background was clearly visible in the card results of red blood cell reagent treated by other concentrations of GA/PFA treated solution, whereas in the sixth month, the negative background was no longer visible.
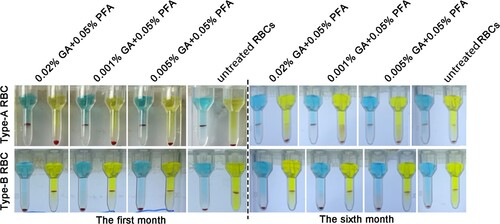
3.1.2.2. Plasma
demonstrated that both the positive result (red blood cells collected at the top of the microcolumn gel card) and the negative result (red blood cells collected at the bottom of the microcolumn gel card) were acceptable, and there was no cause for concern. Red blood cells of type A had agglutinate with plasma of types B and O, but not with plasma of types A and AB. Red blood cells of type B had agglutinate with plasma of types A and O, but not with plasma of types B and AB. In the first month, red blood cells were treated with 0.02% GA /0.05% PFA and 0.01% GA /0.05% PFA, and the negative background of the microcolumn gel card was obviously unclear. After centrifugation, the column contained red particles. Moreover, the image demonstrated that the red particles in the background of type A red blood cell reagent are more prominent than those of type B red blood cell reagent. At the sixth month, red blood cells were treated with 0.02% GA /0.05% PFA and 0.01% GA /0.05% PFA, and the negative background of the microcolumn gel card was obviously unclear. After centrifugation, the column contained red particles. Red blood cells were treated with 0.005% GA /0.05% PFA; the negative background in the microcolumn gel card was clear, and there were no red particles in the column, which was consistent with the untreated red blood cells reagent. The negative context was obscure. After centrifugation, the presence of red particles in the column was primarily attributable to the increase in red blood cell volume and decrease in deformability caused by glutaraldehyde and paraformaldehyde fixation of red blood cells, and the inability of the treated red blood cells to pass through the microcolumn's gel pores. In clinical reverse typing detection, the new reagent developed by treating red blood cells with 0.005% GA/0.05% PFA can replace the commercial red blood cell reagent. In addition, it should be noted that the number of tubes in each group increased in comparison to 3.1.2.1 because 3.1.2.1 reacted with anti-A and anti-B antibody reagents, whereas this section reacted with plasma from A, B, O, and AB individuals.
Figure 3. The results of a microcolumn gel card examination of a GA/PFA treated red blood cell reagent in plasma (In the sets of four tubes, the plasma of blood types A, B, O, and AB are arranged from left to right). In the card test results of 0.005% GA+0.05% PFA treated red blood cell reagent at the first and sixth months, the negative background was unmistakable and the antigen intensity was 4+. In the card results of red blood cell reagent treated by other concentrations of GA/PFA treated solution, the negative background was visible in the first month, but it was not visible in the sixth month.
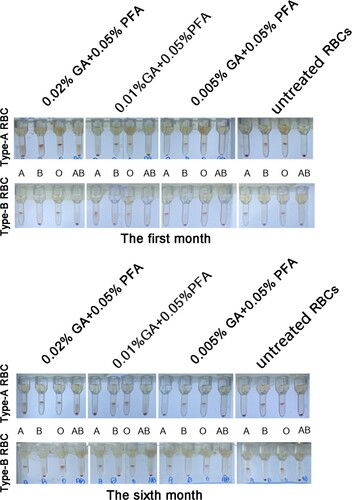
3.2. Hemolysis of red blood cell
3.2.1. The appearance of RBC
The color of the supernatant of A/B GA/PFA-treated red blood cell reagent and untreated red blood cell reagent had not changed in the first month, and the supernatant and red blood cells were clearly layered (). At six months, the color of the supernatant of A/B red blood cell reagent treated with glutaraldehyde did not visibly change, whereas the color of the supernatant of untreated red blood cell reagent visibly changed to red. Fortunately, no matter whether GA/PFA was used to treat red blood cells or untreated red blood cells, the layered red blood cells were obviously separated from the supernatant. The red color of supernatant was caused by the rupture and hemolysis of red blood cells, as well as the exudation of hemoglobin.
Figure 4. The appearance of RBCs in the first and sixth months after treatment with various concentrations of treated solution. The color of treated red blood cell reagents containing 0.01% GA+0.05% PFA and 0.005% GA+0.05% PFA did not change significantly in the first and sixth months, whereas the color of untreated red blood cell reagents became notably red in the sixth month.
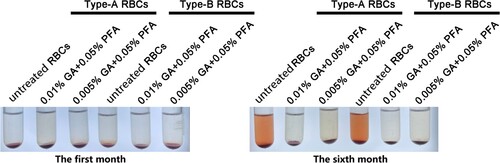
3.2.2. Free hemoglobin content in supernatant
demonstrated that the concentration of free hemoglobin in the supernatant of type A /B red blood cell reagents was low during the first three months, whereas the concentration of free hemoglobin in the supernatant of untreated red blood cell reagents had increased significantly since the third month, while the concentration of red blood cell hemoglobin treated with 0.005% GA /0.05% PFA had not increased significantly. It demonstrated that untreated red blood cell reagents exhibit severe hemolysis after the third month. In the third month, the difference in hemoglobin content between untreated A-type red blood cell reagent and untreated B-type red blood cell reagent is primarily attributable to the fact that in three parallel experiments of untreated B-type red blood cells, the hemoglobin content in one experiment was lower, while the results in the other two experiments were higher, which was not significantly different from that of untreated A-type red blood cells (shown by the error bar in ). The low hemoglobin concentration in the supernatant of untreated type B red blood cells may be due to human operation error or variations in erythrocyte fragility between individuals. Specific values of free hemoglobin in supernatant can be found in Table S3 of the supplementary materials.
3.3. Results of the erythrocyte antibody reagent titration
demonstrated that the antibody titer of red blood cell reagents was lower in the sixth month than in the first. However, the reduction in the 0.005% GA/0.05% PFA group was not evident, and the antibody titer in the first and sixth months of this group was comparable, so the error margin could be considered unchanged. Red blood cell reagents treated with 0.02% GA /0.05% PFA and 0.01% GA/0.05% PFA were incapable of detecting antibodies by the sixth month. Similarly, the antibody titer of untreated red blood cell reagent decreased significantly, which was primarily attributable to the hemolysis of untreated red blood cell reagent.
Table 1. Reaction outcomes of red blood cell reagents with antibodies at various dilutions.
3.4. RBC specificity
The results indicated that GA/PFA treated RBC had the same specificity as untreated RBC. The results of treating red blood cells with GA and PFA were detailed in Table S4 of the supplementary materials.
3.5. Dispersion of red blood cells
To confirm that the agglutination caused by the combination of erythrocyte surface antigen and specific antibody is not nonspecific agglutination caused by time or other factors, we observed the dispersibility of erythrocytes six months after treatment. We found that GA/PFA treated red blood cells, untreated red blood cells after 6 months of storage, and fresh red blood cells were well dispersed under the microscope, and there was no significant difference between the three types (). This phenomenon eliminated nonspecific agglutination from the antigen–antibody reactions.
3.6. Verification of the precision of GA/PFA treated red blood cell test results
3.6.1. Application of treated RBC in storage for the sixth month by the tube test
In this experiment, both untreated and GA+PFA-treated red blood cells stored for six months were used to react with plasma, and the results were observed and recorded. Within 24 h, the fresh red blood cells react with the same batch of plasma to determine the plasma's blood type. The accuracy of GA+PFA-treated red blood cells and untreated red blood cells stored for six months was then evaluated. The results demonstrated that the GA/PFA treated red blood cell reagent which had been stored for six months (100%, n = 24) was more accurate than the untreated red blood cell reagent which had been stored for six months (96%, n = 24). Untreated red blood cell reagents may be ineffective for detecting type A plasma ().
Table 2. Verification of the precision of GA/PFA treated red blood cell reagents using tube tests.
3.6.2. Application of treated RBC in storage for the sixth month by the gel card
To determine the accuracy of GA/PFA treated red blood cells, we compared the results of the reverse typing test of GA/PFA treated red blood cells stored for 6 months, untreated red blood cells stored for 6 months, and fresh red blood cells in 24 h in gel cards. We also compared the results of fresh red blood cells in the test tube method (gold standard). The results demonstrated that the accuracy of GA/PFA treated blood cells was 100% (n = 35), which was superior to that of untreated blood cells (77%, n = 35) ().
Table 3. Verification of the precision of GA/PFA treated red blood cell reagents using the column gel method.
4. Discussion
Red blood cells are preserved by red blood cell preservation solution, which contains trisodium citrate, sodium chloride, glucose, Sucrose, adenine, creatine, kanamycin, chloramphenicol, and sodium azide, among others (the components of the commercialized red blood cell reagents are the same as those in 2.2.3). The novel reagent developed in this experiment enhances the treatment effect of GA and PFA, thereby extending the storage time of commercial red blood cell reagents from 2∼3 months to 6 months. In other words, the preservation time of the novel red blood cell preservation reagent developed in this experiment is two-to-three times longer than commercial red blood cell preservation reagent. In this experiment, the inventive concept is that the untreated red blood cell reagent is fresh red blood cells preserved by red blood cell preservation solution, which is consistent with the preparation method and preservation method of commercial red blood cell reagent, i.e. the untreated red blood cell reagent is equivalent to commercial red blood cell reagent. In this experiment, GA and PFA are used to treat red blood cells, which is equivalent to comparing GA and PFA to treat red blood cells with commercial red blood cells.
In this experiment, red blood cells were treated with GA and PFA to develop a new red blood cell reagent that effectively prolonged the storage time of red blood cell reagent. Currently, Yu Y has published a report on the treatment of red blood cells with aldehyde reagents. This experiment conforms to the experimental design of Yu Y [Citation31]. Red blood cells are fixed by aldehyde reagent, but the type and concentration of aldehyde reagent vary. In Yu Y's study, the storage time of red blood cell reagents treated with 0.025% GA is 3 months, whereas in the experiment, the storage time of red blood cell reagents treated with 0.005% GA and 0.05% PFA is 6 months, doubling Yu Y's storage time. Yu Y investigates the effects of GA and PFA on the antigen intensity of antigens other than A and B, including D, C, E, C, E, M, N, S, S, K, P1, FY (a), JK (a), and LE (a). However, other than A and B antigens, no other antigens were investigated in this experiment, which was primarily for research purposes. In this experiment, only red blood cell reagents were prepared to identify anti-A/ anti-B antibodies used for reverse typing detection; no other antigens were involved.
In this experiment, GA and PFA were found to be effective in treating red blood cells, and a novel red blood cell reagent was developed without affecting antigen performance. In this experiment, 25% GA mother liquor and 4% PFA mother liquor with determined concentrations were diluted in series to treat red blood cell reagents. Among them, observing the flow of red blood cells after centrifugation can judge the fixation effect of GA and PFA on red blood cells. After centrifugation, red blood cells will settle to the bottom of the test tube when treated with GA and PFA. When the supernatant is poured, the red blood cells at the bottom of the test tube will not flow out of the test tube with the supernatant. However, when the untreated red blood cell reagent is centrifuged and the supernatant is poured, the red blood cells will flow with the supernatant as it flows out of the test tube, or even flow out of the test tube. This phenomenon provides evidence that GA and PFA can fix red blood cells.
In this experiment, the novel reagent of 0.005% GA /0.05% PFA for treating red blood cells was compared to the untreated red blood cell reagent (commercial red blood cell reagent), and the antigen intensity detected by the test tube method remained the same for six months. When detecting the antibody titration, however, the new reagent of 0.005% GA /0.05% PFA for treating red blood cells was compared to the first and sixth months. Antibody titrations decreased in untreated red blood cell reagent and red blood cell reagent treated with other concentrations. It demonstrated that hemolysis had an effect on the antibody titer of red blood cell reagents (). After six months of storage, the negative background detected by microcolumn gel card was distinct ( and ), and the dispersion was consistent (). The absence of hemolysis is an advantage of the novel reagent for treating red blood cells with 0.005% GA /0.05% PFA over the untreated red blood cell reagent (commercial red blood cell reagent). Upon observing the appearance and free hemoglobin in the supernatant of the untreated red blood cell reagent within six months, it was determined that the reagent exhibited strong hemolysis ( and ). Similarly, the antigen intensity of other red blood cell reagents (0.02% GA /0.05% PFA, 0.01% GA /0.05% PFA) did not meet the clinical standard (), so they cannot be used for clinical detection. The experiment demonstrated that the accuracy of the 0.005% GA /0.05% PFA reagent for treating red blood cells is superior to that of the reagent for untreated red blood cells ( and ). Red blood cell reagents were best treated with 0.005% GA/0.05% PFA. A novel red blood cell reagent developed by treating red blood cells with 0.005% GA /0.05% PFA is suitable for clinical reverse typing detection.
The novel red blood cell reagent for reverse typing that has been treated with GA and PFA can be stored at 4°C for 6 months, effectively reducing the cost of purchasing reagents for the blood transfusion department. Similarly, GA and PFA are cheap and simple to acquire, and they are anticipated to be utilized in antibody screening cells and antibody identification cells.
Nonetheless, this experiment has some restrictions. In this experiment, the hemolysis rate was determined by the appearance of the reverse-typed red blood cell reagent and the concentration of free hemoglobin in the supernatant. However, the proportion of red blood cells was not determined. In subsequent experiments, it is therefore necessary to determine check for percentage of intact cells using an abalone technical plate or flow cytometry.
5. Conclusion
The novel red blood cell reagent treated with 0.005% GA /0.05% PFA established in this study can extend the storage time of red blood cell reagent to 6 months, which is 2–3 times longer than the current commercial red blood cell reagent. Moreover, the reagent is compatible with microcolumn gel technology, which is anticipated to replace the commercial red blood cell reagent for reverse typing detection.
Supplemental Material
Download MS Word (20.5 KB)Acknowledgements
We thank all study participants for their time and support. The authors also thank the CAS Key Lab of Bio-Medical Diagnostics, Suzhou Institute of Biomedical Engineering and Technology, and Chinese Academy of Sciences for their support.
Data availability statement
Data available on request from the authors.
Disclosure statement
No potential conflict of interest was reported by the author(s).
Additional information
Funding
References
- Ebrahimi Fana S, Paknejad M, Aminian M. Paper based analytical devices for blood grouping: a comprehensive review. Biomed Microdevices. 2021;23(3):34. doi:10.1007/s10544-021-00569-w
- Zhang H, Liu R, Li Q, et al. Flipped quick-response code enables reliable blood grouping. ACS Nano. 2021;15(4):7649–7658. doi:10.1021/acsnano.1c01215
- Akinyemi TO, Fasola FA, Olateru-Olagbegi OA. The evolving application of DNA-based genotyping of red blood cells in blood grouping: a narrative review. West Afr J Med. 2021;38(3):269–273.
- Ekman S, Flower R, Barnard RT, et al. Computational modeling - an approach to the development of blood grouping reagents. Expert Rev Hematol. 2021;14(4):329–334. doi:10.1080/17474086.2021.1908119
- Miller FP, Vandome AF, Mcbrewster J, et al. British Pharmacopoeia Commission. Third Addendum to the British Pharmacopoeia, 1932. Majesty’S Stationery Office, 1977.
- Andıç N. Practical solutions for problems in blood grouping and crossmatching. Turk J Haematol. 2022;39(1):55–60. doi:10.4274/tjh.galenos.2021.2021.0544
- Chenna D, Shastry S, Kanukula VR, et al. Validation of lateral flow assay for blood grouping on hemolyzed sample. Asian J Transfus Sci. 2020;14(1):98. doi:10.4103/ajts.AJTS_28_17
- Stoll C, Stadnick H, Kollas O, et al. Liposomes alter thermal phase behavior and composition of red blood cell membranes. Biochim Biophys Acta. 2011;1808(1):474–481. doi:10.1016/j.bbamem.2010.09.012
- Eades B. Freezing and recovering rare red blood cells using glycerol. Immunohematology. 2020;36(3):85–88.
- Emrich T, Stracke JO, Guo X, et al. Increasing robustness, reliability and storage stability of critical reagents by freeze-drying. Bioanalysis. 2021;13(10):829–840. doi:10.4155/bio-2020-0299
- Alves D, Sparrow R, Garnier G. Rapidly freeze-dried human red blood cells for pre-transfusion alloantibody testing reagents. J Biomed Mater Res B Appl Biomater. 2021;109(11):1689–1697. doi:10.1002/jbm.b.34825
- Yoshida T, Prudent M, D’alessandro A. Red blood cell storage lesion: causes and potential clinical consequences. Blood Transfus. 2019;17(1):27–52. doi:10.2450/2019.0217-18
- Turner TR, Lautner L, Hill A, et al. Evaluating the quality of red blood cell concentrates irradiated before or after cryopreservation. Transfusion. 2020;60(1):26–29. doi:10.1111/trf.15589
- Downing RG, Strong PL, Hovanec BM, et al. Considerations in the determination of boron at low concentrations. Biol Trace Elem Res. 1998;66(1-3):3–21. doi:10.1007/BF02783122
- Wolkers WF, Oldenhof H. Principles underlying cryopreservation and freeze-drying of cells and tissues. Methods Mol Biol. 2021;2180:3–25. doi:10.1007/978-1-0716-0783-1_1
- Sengupta P, Mahalakshmi V, Stebin JJ, et al. Nitric oxide donors offer protection to RBC from storage lesion. Transfus Clin Biol. 2020;27(4):229–236. doi:10.1016/j.tracli.2020.07.002
- Feng Y, Ping Tan C, Zhou C, et al. Effect of freeze-thaw cycles pretreatment on the vacuum freeze-drying process and physicochemical properties of the dried garlic slices. Food Chem. 2020;324:126883. doi:10.1016/j.foodchem.2020.126883
- Schmid P, Huvard MJ, Lee-Stroka AH, et al. Red blood cell preservation by droplet freezing with polyvinylpyrrolidone or sucrose-dextrose and by bulk freezing with glycerol. Transfusion. 2011;51(12):2703–2708. doi:10.1111/j.1537-2995.2011.03258.x
- García-Roa M, Del Carmen Vicente-Ayuso M, Bobes AM, et al. Red blood cell storage time and transfusion: current practice, concerns and future perspectives. Blood Transfus. 2017;15(3):222–231. doi:10.2450/2017.0345-16
- Adamiak K, Sionkowska A. Current methods of collagen cross-linking: review. Int J Biol Macromol. 2020;161:550–560. doi:10.1016/j.ijbiomac.2020.06.075
- Neufang A, Duenschede F, Espinola-Klein C, et al. Contemporary results with the biosynthetic glutaraldehyde denatured ovine collagen graft (Omniflow II) in femoropopliteal position. J Vasc Surg. 2020;71(5):1630–1643. doi:10.1016/j.jvs.2019.08.234
- Khapre MA, Pandey S, Jugade RM. Glutaraldehyde-cross-linked chitosan–alginate composite for organic dyes removal from aqueous solutions. Int J Biol Macromol. 2021;190:862–875. doi:10.1016/j.ijbiomac.2021.09.026
- Anastassacos FM, Zhao Z, Zeng Y, et al. Glutaraldehyde cross-linking of oligolysines coating DNA origami greatly reduces susceptibility to nuclease degradation. J Am Chem Soc. 2020;142(7):3311–3315. doi:10.1021/jacs.9b11698
- Rodig SJ. Fixing attached cells for staining. Cold Spring Harb Protoc. 2020;2020(8):099689. doi:10.1101/pdb.prot099689
- Qin Y, Jiang W, Li A, et al. The combination of paraformaldehyde and glutaraldehyde Is a potential fixative for mitochondria. Biomolecules. 2021;11(5):711. doi:10.3390/biom11050711
- Tanaka KAK, Suzuki KGN, Shirai YM, et al. Membrane molecules mobile even after chemical fixation. Nat Methods. 2010;7:865–866.
- Thavarajah R, Mudimbaimannar V, Elizabeth J, et al. Chemical and physical basics of routine formaldehyde fixation. J Oral Maxillofac Pathol. 2012;16:400–405.
- Kiernan JA. Formaldehyde, formalin, paraformaldehyde and glutaraldehyde: what they are and what they do. Microsc Today. 2000;8:8–13.
- Mena SE, de Beer MP, McCormick J, et al. Variable-height channels for microparticle characterization and display. Lab Chip. 2020;20(14):2510–2519. doi:10.1039/d0lc00320d
- Immerman KL, Melaragno AJ, Ouellet RP, et al. Morphology of glutaraldehyde-fixed preserved red blood cells and 24-hr post-transfusion survival. Cryobiology. 1983;20(1):30–35. doi:10.1016/0011-2240(83)90056-1
- Yu Y, Sun X, Guan X, et al. Effects of hydroformylation treatment on the storage time and blood group antigen expressions of reagent red blood cells. Transfus Apher Sci. 2014;50(3):462–466. doi:10.1016/j.transci.2014.02.019