ABSTRACT
Background:
T cells’ function and activation and the immunosuppressive effect of regulatory T cells (Tregs) play a pivotal role in the occurrence and progression of acute myeloid leukemia (AML). In this study, we investigate the expression of T cell activation markers and quantity of Tregs in bone marrow (BM) and peripheral blood (PB) from AML patients and further characterized their correlation with BM leukemic blasts.
Methods:
Expression of CD25, CD38, CD69, and HLA-DR on the surfaces of CD4+ and CD8+ T cells and the quantity of Tregs in BM and PB from new diagnosed (ND), relapsed-refractory (RR), complete remission (CR) AML patients were measured via flow cytometry.
Results:
Compared to normal controls (NC), we found higher proportion of CD4+ CD69+ T cells, CD8+ CD69+ T cells and Tregs in PB. CD8+ CD38+ T cells and CD8+ HLA-DR+ T cells in RR were significantly higher than ND, CR and NC). Tregs were normalized when AML patients achieved CR. Moreover, there was a minor positive correlation between AML blasts and CD8+ CD25+ T cells or Tregs, while AML blasts had a minor negative correlation with CD4+ CD69+ T cells.
Conclusion:
Abnormal activation markers of T cells and Tregs may be involved in the pathological mechanism of ND and RR AML. Our results indicated that CD8+ CD38+ T cells and CD8+ HLA-DR+ T cells might be RR markers of AML patients. Furthermore, Tregs could be used as clinical indicators to evaluate prognosis for AML patients.
Introduction
Acute myeloid leukemia (AML) is a highly heterogeneous hematological disorder characterized by incremental bone marrow (BM) leukemic blasts which can infiltrate into peripheral blood (PB) or other tissues [Citation1,Citation2]. AML is initially derived from BM hematopoietic stem cells, which proliferate abundantly in BM before migrating to PB. Consequently, the occurrence and progression of AML are chiefly dependent on the bone marrow microenvironment [Citation3]. T cells are a crucial component of the immune microenvironment and also play a prominent role in anti-tumor immune response [Citation4]. Currently, relatively little is known about the functional status of T cells in BM of AML patients. Ismail et al. described an association between a high percentage of T cells in the BM and improved overall and leukemia-free survival in newly diagnosed AML patients [Citation5]. A recent study showed T cells’ infiltration in the BM microenvironment of newly diagnosed AML patients was significantly associated with increased overall survival, and AML established an immunosuppressive environment in BM through T cells’ checkpoint function [Citation6]. Although the pathogenesis of AML is not fully understood, activated T cells appear to play an important role in AML. However, little is known about the activation markers of CD4+ and CD8+ T cells in BM and BP of AML patients. Therefore, better assessment of immune cell status and T cells activation within the BM of AML patients may be important for uncovering the pathogenesis of leukemia and developing new therapeutic approaches.
Regulatory T cells (Tregs) are an important cell population with immunosuppressive effects in the leukemia bone marrow microenvironment [Citation7]. Foxp3 is a specific marker in CD4+ CD25+ Tregs and also the major driver of Treg differentiation [Citation8]. Foxp3+ Treg is characterized by low expression of CD127 and high expression of CD25 [Citation9]. Clinician and scholars consider CD4+ CD25+ CD127neg classification as Tregs. Several studies have demonstrated that the frequencies and function of Tregs increased in the BM and PB, which was associated with pool prognosis of AML patients [Citation10–12]. Zhou et al. suggested that the immunosuppressive effect of Tregs facilitated the survival of leukemic blasts, manifested by the ability of Tregs to hinder the expansion of cytotoxic T lymphocyte [Citation13]. A recent study showed that Tregs can activate the PI3 K/AKT signaling pathway and promote the stemness of AML cells through releasing high levels of the anti-inflammatory cytokine IL-10 [Citation14]. However, the relationship between Tregs in AML and BM leukemic blasts and the assessment of whether Tregs could be used as relapsed or prognostic indicators in AML are poorly understood.
CD38, CD25, CD69, and HLA-DR are the important markers for assessing the activation status of T cells. Among them, CD25 (also known as IL-2 receptor), is critical in maintaining Tregs mediated immune tolerance to self-antigens [Citation15,Citation16]. In this study, we comprehensively compared the expression of CD38, CD25, CD69, HLA-DR, and Tregs in both BM and PB T cells from AML patients at different stages. Moreover, we further characterized their correlation with BM leukemic blasts and evaluated their relationship in the progression of AML patients.
Materials and methods
Patients and controls
All samples were obtained with consent, and ethical approval was obtained from the Ethics Committee of Sun Yat-sen Memorial Hospital, Sun Yat-sen University. All AML patients were diagnosed and classified based on the World Health Organization classification of AML [Citation17]. CR was defined based on the International Working Group Criteria [Citation18]. In this study, a total of 26 BM samples and 24 PB samples were collected from ND AML patients [(range, median age), (BM: 14-71, 46.5; PB: 14-71, 46.5)], including 24 paired samples; 25 BM samples and 20 PB samples were collected from AML patients with RR (BM: 19-77, 46; PB: 22-77, 46), including 15 paired samples; 19 BM samples and 21 PB samples were collected from AML patients who achieved CR (BM: 18-65, 44; PB: 18-65, 44), including 8 paired samples. Moreover, 11 unpaired BM samples (21-61, 40) with slight iron deficiency anemia, having no immunological changes, and 25 PB samples from healthy volunteers (20-62, 38) were used as normal controls. The clinical characteristics of total AML patients and normal controls are shown in . The WHO classification, mutation and ELN risk status of AML are shown in Supplementary Table 1. All samples were freshly obtained in ethylenediamine tetra acetic acid (EDTA-2 K)- tubes and tested within 8 h of collection.
Table 1. Clinical characteristics of AML patients and normal controls.
Therapeutic regimen
ND AML patients with non-M3 AML subtypes were given standard induction chemotherapy with idarubicin (IDA) or mitoxantrone for 3 days and cytarabine (Ara-C) for 7 days. ND AML patients with M3 subtype received all-trans retinoic acid (ATRA) and arsenical with or without concurrent induction chemotherapy. RR AML patients received salvage chemotherapy or targeted therapy (e.g. AML with FLT3-ITD/TKD mutation were given gilteritinib for treatment). After the patients achieved CR, they were given consolidation chemotherapy with a high dose of Ara-C or with a standard dose of Ara-C and one anthracycline.
Multi-color flow cytometry analysis
Cell surface staining for multi-color flow cytometry was performed using the following fluorochrome conjugated monoclonal antibodies: CD45-KO (Clone: J.33), CD3-FITC (Clone: UCHT1), CD4-PC5.5 (Clone: J.33), CD25-PC7 (Clone: B1.49.9), CD69-PE (Clone: TP1.55.3), HLA-DR-APC (Clone: Immu-357), CD127-PE (Clone: R34.34), and erythrocyte lysis solution (all purchased from Beckman Coulter). CD8-APC-CY7 (Clone: SK1) and CD38-V450 (Clone: H87) were purchased from BD Biosciences. We constructed two panels to analyze cells surface markers, and fluorochrome labeled isotype-match antibodies were used as negative controls. Briefly, 100 μL BM or PB samples was mixed and incubated for 30 min in the dark at room temperature with antibodies or isotype controls (Fc blocking antibodies were added before the mix of fluorescently labeled antibodies). Followed by lysis with 500 µL of erythrocyte lysis solution before two washes with 1 mL of phosphate buffer saline (PBS). The supernatants were discarded after 5 mins/1500 rpm centrifugation, and cell pellets were resuspended in 500 µL of PBS for further multi-color flow cytometer analysis. Cells were analyzed with a Navios Flow Cytometer (Beckman Coulter), and data off-line analysis was performed with Kaluza software 2.1 (Beckman Coulter). The gating strategy for the T cells activation markers and Tregs is shown in . Debris, dead cells, and adhesions were removed via live/dead staining and SSC x FSC. Lymphocytes were gated among all events according to CD45 and SSC. CD3+ T cells were then gated in lymphocytes (Gate Lym). Activation markers on CD4+ and CD8+ T cells were shown in gated CD3+ T cells and CD4+ CD25+ CD127neg Tregs were gated in CD3+ CD4+ T cells.
Figure 1. The gating strategy for the T cells activation markers and Tregs. Representative flow cytometry plots showing T cell activation markers and Tregs in AML patients. Lymphocytes were first gated among all events according to CD45 and side-scatter. CD3+ T cells were then gated in lymphocytes (Gate Lym). Activation markers on CD4+ and CD8+ T cells were shown in gated CD3+ T cells and CD4+ CD25+ CD127neg Tregs were gated in CD3+ CD4+ T cells (the gating strategy of removing debris, dead cells and adhesions not shown above).
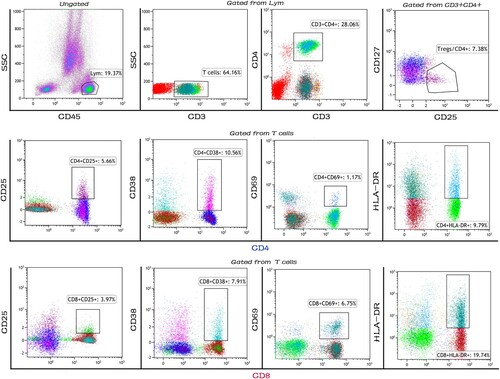
Statistical analysis
Statistical analyses were conducted using IBM SPSS Statistics 22.0 software. All data are presented as mean ± standard deviation. Expressions of T cell activation markers and Tregs for multiple groups were performed with Kruskal–Wallis tests, and One-way ANOVA was performed for data comparison among multiple groups if the data were normally distributed. Differences of frequencies in BM and PB from the same patients were performed using paired t-test. Spearman’s correlation was performed to assess the correlation between variables. Value of p < 0.05 was considered statistically significant.
Results
The distribution of T cells activation markers and Tregs in the BM and PB of AML patients at different stages
To evaluate the activated status of T cells in BM and PB from AML patients, we first measured the expression of T cell activation markers and compared them on CD4+ and CD8+ T cells from the BM and PB of AML patients in the ND, RR, CR stages and NC (Supplementary Table 2). In BM samples (See A), we observed a significant decrease of CD4+ CD25+ T cells (p = 0.002) and CD4+ CD38+ T cells (p = 0.007) in RR compared with the proportion in NC. In contrast, we found a significant increase of CD8+ CD38+ T cells (p < 0.0001), CD8+ CD69+ T cells (p = 0.001), and CD8+ HLA-DR+ T cells (p < 0.0001) in RR compared with the proportion in ND and NC. We also found the frequency of CD8+ CD38+ T cells (p = 0.048) was significantly decreased in CR compared to ND, and it was significantly elevated in RR compared to CR (p = 0.001). Meanwhile, in PB samples (B), RR had a lower proportion of CD4+ CD25+ T cells (p = 0.004) and CD4+ CD38+ T cells (p < 0.0001) compared to NC. In contrast, CD8+ CD38+ T cells (p < 0.0001) and CD8+ HLA-DR+ T cells (p < 0.0001) in RR were significantly increased than their proportion in ND and NC. Moreover, compared to CR, CD8+ CD38+ T cells and CD8+ HLA-DR+ T cells in RR were significantly increased. Interestingly, these results are consistent with those in BM samples, which indicate that there is a certain correlation between immune cells in the BM microenvironment of AML patients and PB. In addition, ND patients had a higher percentage of CD4+ CD69+ T cells (p < 0.0001) than that in RR and NC. Compared to NC, ND and RR patients had higher percentages of CD8+ CD69+ T cells.
Figure 2. The distribution of T cell activation markers and Tregs in the BM and PB of AML Patients at different stages. (A) The percentage of T cell activation markers from BM samples in each group. (B) The percentage of T cell activation markers from PB samples in each group. (C) The frequency and absolute number of Tregs from the BM in each group. (D) The frequency and absolute number of Tregs from the PB in each group. (BM: bone marrow; PB: peripheral blood; ND: newly diagnosed AML patients; RR: AML patients in relapsed-refractory conditions; CR: AML patients in complete remission; NC: normal controls; *p < 0.05; **p < 0.01; ***p < 0.001.)
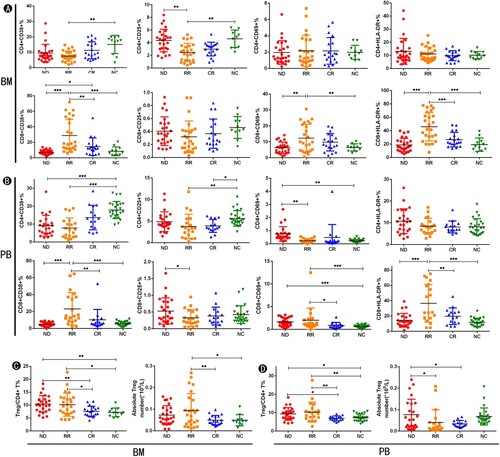
We then analyzed the frequency and absolute counts of Tregs from BM and PB of AML patients at different stages as well as NC (See C&D). We detected a higher proportion of Tregs from ND (p = 0.006; p = 0.008) and RR (p = 0.012; p = 0.014) when compared with CR and NC in BM/PB samples. Moreover, the absolute number of BM Tregs in RR was significantly increased compared to CR (p = 0.007) and NC (p = 0.020), while ND had a higher absolute number of PB Tregs than those in RR (p = 0.027) and CR (p = 0.017). Furthermore, the level of the Tregs was almost normal when the AML patients achieved CR (p > 0.05).
The results above indicated that AML patients at different stages have abnormal T cell activated status. Upregulation of CD8+ CD38+ T cells and CD8+ HLA-DR+ T cells in BM or PB are a characteristic of patients with RR AML patients. Besides, increased percentage of CD4+ CD69+ T cells and CD8+ CD69+ T cells in PB are a characteristic of patients with ND AML. Higher expression of CD8+ CD38+ T cells and CD8+ HLA-DR+ T cells might be a sign of relapsed-refractory disease. Moreover, Tregs in BM as well as PB expression recovery might be prognostic indicators of disease remission.
Comparison of the proportion of T cells activation markers and Tregs in BM and PB from the same patients with AML at different stages
To better evaluate whether the immune status is different between BM and PB of AML patients, we assessed the expression of T cell activation markers and Tregs from both sites. We first assessed the T cells composition of paired BM and PB from the same patients in ND AML. We observed that the proportion of CD3+ T cells and CD8+ T cells among lymphocytes are significantly decreased in BM compared to PB (See Figure S1). This implied that the anti-leukemic capacity of CD8+ T cells in the BM of AML patients is suppressed. We also observed that CD4+ T cells in BM were elevated compared to PB. We then observed a significantly increased frequency of CD4+ CD69+ T cells (p = 0.0022), CD8+ CD38+ T cells (p < 0.0001), CD8+ CD69+ T cells (p < 0.0001), and CD8+ HLA-DR+ T cells (p = 0.0172) in BM compared with those of PB from ND AML patients (See A). In RR AML patients, the results showed that the proportion of CD4+ CD69+ T cells (p = 0.0036), CD4+ HLA-DR+ T cells (p = 0.0316), CD8+ CD69+ T cells (p = 0.0009), and CD8+ HLA-DR+ T cells (p = 0.0024) was significantly increased in BM compared with those of PB (See B). We also investigated a significantly increased proportion of CD8+ CD69+ T cells (p = 0.0176) and CD8+ HLA-DR+ T cells (p = 0.0198) in BM compared with those of PB from CR AML patients (See C). We further examined the proportion of Tregs and found that the frequency of Tregs was significantly increased in BM compared with that of PB from ND AML patients. Interestingly, the percentage of Tregs was significantly higher in BM compared with that of PB from CR. There were no significant differences in the proportion of Tregs in RR AML patients (See D). These results suggest that the BM microenvironment of AML patients possesses hyper expressions of T cell activation markers and Tregs compared with PB. The stronger immunosuppression in the BM microenvironment of AML patients might promote progression and relapsed-refractory AML, and restoring the immunosuppression in the BM microenvironment might be a key factor for preventing relapsed-refractory AML and treating AML patients.
Figure 3. Comparison of the expression of T cell activation markers from the same patient in BM and PB of AML patients. (A) The expression of T cell activation markers between BM and matched PB of ND AML patients (n = 24). (B) The expression of T cell activation markers between BM and matched PB of RR AML patients (n = 15). (C) The expression of T cell activation markers between BM and matched PB of ND AML patients (n = 8). (D) The proportion of Tregs between BM and matched PB of ND (n = 24), RR (n = 15) and CR (n = 8) AML patients. (BM: bone marrow; PB: peripheral blood; ND: newly diagnosed AML patients; RR: relapsed-refractory AML patients; CR: AML patients in complete remission; NC: normal controls.)
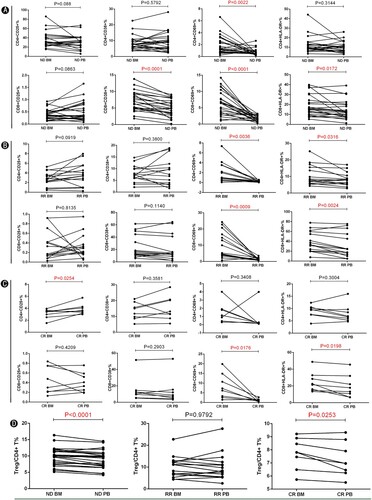
Correlation between the proportion of T cell activation markers or Tregs and AML blasts
Complex components of the BM microenvironment are critical for maintaining the survival of leukemic blasts, but little is understood about the correlation between AML blasts and activated T cells or Tregs. Therefore, we investigated the correlation between the proportion of T cell activation markers or Tregs and AML blasts. We found that AML blasts had a minor positive correlation with CD8+ CD25+ T cells (n = 70, r = 0.2418, p = 0.0437) and Tregs (n = 70, r = 0.2781, p = 0.0197). In contrast, AML blasts had a minor negative correlation with CD4+ CD69+ T cells (n = 70, r = −0.2352, p = 0.049). In addition, there was no correlation with AML blasts between the CD8+ HLA-DR+ T cells, CD8+ CD38+ T cells, and activation markers of CD4+ T cells (See ). These results suggest that the higher proportion of AML blasts in the BM microenvironment of AML patients might enhance a higher expression of CD8+ CD25+ T cells or lower expression of CD4+ CD69+ T cells and immunosuppressive effect of Tregs.
Figure 4. Correlation between the proportion of T cell activation markers or Tregs and AML blasts in 70 cases of AML patients. The figure above shows AML blasts were positively correlated with CD8+ CD25+ T cells (n = 70, r = 0.2418, p = 0.0437) and Tregs (n = 70, r = 0.2781, p = 0.0197). Simultaneously, AML blasts were negatively correlated with CD4+ CD69+ T cells (n = 70, r = −0.2352, p = 0.049).
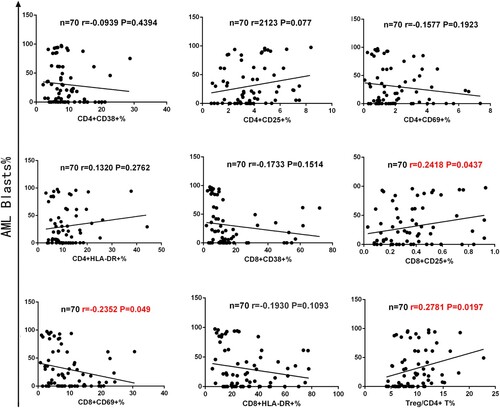
Discussion
The AML BM microenvironment (‘home’ of the AML blasts) is extremely complex, and soluble factors secreted by AML blasts can hinder T cells proliferation and activation [Citation19]. The immunosuppressive effect of Tregs, T cell functions, and anti-tumor effects play a key role in the occurrence and development of AML. Previous studies on T cell activation markers in hematological diseases mostly focused on PB rather than BM [Citation20–23]. However, relevant study of T cells activation status and Tregs of BM or PB from AML patients at different stages was lacking. In this present study, we synthetically compared the distribution of T cell activation markers and Tregs in BM and PB from AML patients at different disease stages. We found that T cell activation markers were aberrantly expressed and Tregs were upregulated in BM and PB of AML patients. Additionally, the level of Tregs in BM and PB were normalized when the AML patients achieved CR.
Lymphocyte activation is accompanied by altered expression of their surface markers, and the expression of distinct activation antigens is crucial for T cells’ function. According to the duration of the activation response after stimulation, lymphocyte activation markers can be separated into early activation markers (CD69 and CD71) and late activation (CD25, CD26, HLA-DR) antigens [Citation24]. CD38 is a transmembrane glycoprotein that has extracellular enzymatic activity and functions as a receptor adhesion molecule. Activated T cells, B cells, and natural killer cells can express high levels of CD38 [Citation25,Citation26]. Chaturvedi et al. indicated CD38 as a crucial marker for identifying T cells activation in patients with hemophagocytic lymphohistiocytosis [Citation27]. An earlier study describes elevated T cell activation markers (CD69, HLA-DR, CD57, and CD71) in PB at diagnosis when compared with normal controls, and characterized systemic T cells activation as a common sign of hematological tumors [Citation28]. CD69, the earliest activation antigen, was over-expressed on infiltrated lymphocytes in different human inflammatory situations [Citation29,Citation30]. A recent study showed that untreated AML patients exhibited signs of increased lymphocyte activation and depletion compared to healthy donors [Citation31]. In our study, we found ND AML patients had higher proportion of CD4+ CD69+ T cells and CD8+ CD69+ T cells in PB compared to the NC. Interestingly, we did not find significant differences in CD4+ CD69+ T cells and CD8+ CD69+ T cells between ND and NC in BM samples, which may be due to the more complex tumor environment in BM from AML patients compared to the circulating environment of PB. In addition, we also found CD8+ CD38+ T cells and CD8+ HLA-DR+ T cells in RR (both in BM and PB) were significantly higher than the ND, CR, and NC groups after a comprehensive analysis of T cell activation markers in BM and PB at different disease stages. The result above suggests that CD8+ T cells in BM with RR are in an overactive state, but whether overactivated T cells are affected in function has not been well elucidated. These findings make it possible for CD8+ CD38+ T cells and CD8+ HLA-DR+ T cells to be indicators of RR AML patients. A variety of immune cells, including CD8+ T cells and their subsets, can be detected in the tumor microenvironment, and the anti-tumor efficacy is mainly attributed to CD8+ T cells [Citation32]. Meanwhile, CD8+ PD-1 and CD8+ TIGIT, two crucial immune checkpoints in AML treatment, were found to be more highly expressed in the BM of ND and RR AML patients compared to PB [Citation33]. Brauneck et al. found an increased proportion of CD8 + CD73negTIGIT+ T cells in ND and RR AML patients [Citation34]. Le Dieu et al. showed that gene expression profiling of CD8+ T cells in PB from ND AML patients characterized an abnormal activation profile and modifications in genes vital for immunologic synapse formation [Citation35]. These all indicate that depletion of T cells’ functions and phenotypic changes play an important role in the progression of AML. However, the dysfunctional state of CD8+ T cells has not been well elucidated in AML. In this study, CD8+ CD38+ T cell and CD8+ HLA-DR+ T cell activation were abnormally over-expressed in the RR AML group, and we speculated that the over-expression of CD8+ T cells might weaken the anti-tumor efficacy and benefits to relapsed-refractory AML. However, the specific mechanism still needs further research.
Tregs are a class of cells with strong immunosuppressive effects in the tumor microenvironment, and their high infiltration is associated with poor survival of many types of cancers. Simultaneously, to deplete Tregs and control their function is one of the important strategies for future cancer immunotherapy [Citation36]. Tregs not only play an immunosuppressive role in the BM of AML patients, but also control peripheral immune tolerance. In our study, we detected elevated proportions of Tregs (both in BM and PB) in ND compared to NC, which was consistent with previous studies [Citation11,Citation12,Citation37]. Moreover, we also detected a high proportion of Tregs (both in BM and PB) in RR compared to NC. These results indicate that Tregs are involved in the pathogenesis of both ND and RR AML patients. In contrast, no statistical significance was found when we compared the absolute counts of Tregs between ND and NC, which might be due to the wide range of WBC counts in ND AML and the usage of dual platforms to calculate absolute counts of Tregs. Notably, the proportion of Tregs were normalized when AML achieved CR, suggesting that Tregs could be used as clinical markers for evaluation of prognosis.
To facilitate clinical monitoring of immune status and understand the dysfunctional immune status of BM and PB in each AML patient, we assessed whether T cells activation markers and Tregs in BM from the same patient were similar to those in PB. In matched BM and PB samples from ND AML patients, the ratio of CD3+ T cells and CD8+ T cells in BM was significantly lower than that in PB, which was consistent with previous studies [Citation38]. We then observed the accumulation of CD4+ CD69+ T cells, CD8+ CD69+ T cells, and CD8+ HLA-DR+ T cells in the BM of ND and RR. Moreover, we found a higher proportion of CD8+ CD38 + T cells in the BM of DN AML patients. With regards to the expression of Tregs, we confirmed previous results that showed the proportion of Tregs in BM was higher than those from PB in the same ND AML patient [Citation12]. These results suggest that the immunosuppressive effect in the BM microenvironment of AML patients might be stronger than that of PB. This may provide a new solution for improving the therapy of AML patients in the future and allow us to focus more on the restoration of the BM immune microenvironment in AML patients. Interestingly, when we evaluated Tregs in matched BM and PB in the CR group, we found that the proportion of Tregs in BM was still higher than that in PB even if AML patients achieved CR, which might be one of the potential reasons for AML relapse after long-term CR in some AML patients. However, more data and research are needed to verify this speculation.
Finally, we analyzed the correlation between the proportion of T cell activation markers or Tregs and AML blasts. We found that AML blasts had a minor positive correlation with CD8+ CD25+ T cells and Tregs. Additionally, AML blasts had a minor negative correlation with CD4+ CD69+ T cells. Xu et al. showed that Tregs can assist AML blasts to evade immune surveillance [Citation14]. Although rare relational human studies have been reported, a recent study showed that co-culture with AML blasts and incubations with AML plasma hinder the proliferation of T cells in vitro [Citation39]. Knaus HA et al. also indicated that AML blasts immediately altered CD8+ T cells expansion, co-signaling, viability, and expression of senescence marker [Citation40]. Our results suggest that AML blasts can inhibit the early activation of CD4+ T cells and promote the expression of Tregs and late activation of CD8+ T cells. Whether the AML blasts in BM of AML patients are correlated with T cell activation and Tregs is worth further study with a large cohort.
Although our data were statistically significant, our study still has some limitations. First, our study was based on a single-center prospective study with a small sample size. Therefore, more data and research and a multi-center prospective study are needed to confirm our conclusion. Second, this study is primarily descriptive in nature, and we could not uncover the potential mechanism by which CD38, CD69, and HLA-DR contributed to ND or RR AML. This study needs to be followed up with additional T cells function (including T cells exhaustion) experiments or analysis of single cell sequencing results. Third, most of the RR AML patients were not diagnosed for the first time in our hospital, and we could not do follow-up studies of their T cell activation markers and Tregs.
In summary, we have phenotypically characterized the BM-resident and PB T cells’ activation markers and Tregs in patients with AML at different disease stages. We found ND AML patients had higher proportions of CD4+ CD69+ T cells, CD8+ CD69+ T cells and Tregs in PB. CD8+ CD38+ T cells and CD8+ HLA-DR+ T cells in RR were significantly higher than ND, CR, and NC groups, which indicated CD8+ CD38+ T cells and CD8+ HLA-DR+ T cells might be a sign of relapsed-refractory AML. Notably, Tregs in BM and PB were restored to normal when the AML patients achieved CR, suggesting that Tregs could be used as clinical markers for evaluation of prognosis. There is a minor positive correlation between the proportion of AML blasts and CD8+ CD25+ T cells or Tregs, while AML blasts have a minor negative correlation with CD4+ CD69+ T cells. In addition, the BM microenvironment of AML patients possess a hyper-expression of both T cell activation markers and Tregs compared with PB in the paired match patients. However, the mechanism of interaction among activated T cell markers, Tregs, and AML blasts still require further study.
Supplemental Material
Download MS Word (20.9 KB)Supplemental Material
Download MS Word (17.1 KB)Disclosure statement
No potential conflict of interest was reported by the author(s).
Additional information
Funding
References
- Estey E, Döhner H. Acute myeloid leukaemia. Lancet. 2006;368(9550):1894–1907.
- Cai SF, Levine RL. Genetic and epigenetic determinants of AML pathogenesis. Semin Hematol. 2019;56(2):84–89.
- Shafat MS, Gnaneswaran B, Bowles KM. Rushworth SA: the bone marrow microenvironment - home of the leukemic blasts. Blood Rev. 2017;31(5):277–286.
- Lamble AJ, Lind EF. Targeting the immune microenvironment in acute myeloid leukemia: a focus on T cell immunity. Front Oncol. 2018;8:213.
- Ismail MM, Abdulateef NAB. Bone marrow T-cell percentage: a novel prognostic indicator in acute myeloid leukemia. Int J Hematol. 2017;105(4):453–464.
- Lamble AJ, Kosaka Y, Laderas T, et al. Reversible suppression of T cell function in the bone marrow microenvironment of acute myeloid leukemia. Proc Natl Acad Sci U S A. 2020;117(25):14331–14341.
- Ustun C, Miller JS, Munn DH, et al. Regulatory T cells in acute myelogenous leukemia: is it time for immunomodulation? Blood. 2011;118(19):5084–5095.
- Kim JM, Rasmussen JP, Rudensky AY. Regulatory T cells prevent catastrophic autoimmunity throughout the lifespan of mice. Nat Immunol. 2007;8(2):191–197.
- Hartigan-O'Connor DJ, Poon C, Sinclair E, et al. Human CD4+ regulatory T cells express lower levels of the IL-7 receptor alpha chain (CD127), allowing consistent identification and sorting of live cells. J Immunol Methods. 2007;319(1–2):41–52.
- Kanakry CG, Hess AD, Gocke CD, et al. Early lymphocyte recovery after intensive timed sequential chemotherapy for acute myelogenous leukemia: peripheral oligoclonal expansion of regulatory T cells. Blood. 2011;117(2):608–617.
- Szczepanski MJ, Szajnik M, Czystowska M, et al. Increased frequency and suppression by regulatory T cells in patients with acute myelogenous leukemia. Clin Cancer Res: An Official Journal Am Assoc Cancer Res. 2009;15(10):3325–3332.
- Shenghui Z, Yixiang H, Jianbo W, et al. Elevated frequencies of CD4+ CD25+ CD127lo regulatory T cells is associated to poor prognosis in patients with acute myeloid leukemia. Int J Cancer. 2011;129(6):1373–1381.
- Zhou Q, Bucher C, Munger ME, et al. Depletion of endogenous tumor-associated regulatory T cells improves the efficacy of adoptive cytotoxic T-cell immunotherapy in murine acute myeloid leukemia. Blood. 2009;114(18):3793–3802.
- Xu Y, Mou J, Wang Y, et al. Regulatory T cells promote the stemness of leukemia stem cells through IL10 cytokine-related signaling pathway. Leukemia. 2022;36(2):403–415.
- Mizui M, Koga T, Lieberman LA, et al. Tsokos GC: IL-2 protects lupus-prone mice from multiple end-organ damage by limiting CD4-CD8- IL-17-producing T cells. J Immunol. 2014;193(5):2168–2177.
- Laurence A, Tato CM, Davidson TS, et al. Interleukin-2 signaling via STAT5 constrains T helper 17 cell generation. Immunity. 2007;26(3):371–381.
- Khoury JD, Solary E, Abla O, et al. The 5th edition of the world health organization classification of haematolymphoid tumours: myeloid and histiocytic/dendritic neoplasms. Leukemia. 2022;36(7):1703–1719.
- Cheson BD, Bennett JM, Kopecky KJ, et al. Revised recommendations of the international working group for diagnosis, standardization of response criteria, treatment outcomes, and reporting standards for therapeutic trials in acute myeloid leukemia. J Clin Oncol: Off J Am Soc Clin Oncol. 2003;21(24):4642–4649.
- Isidori A, Salvestrini V, Ciciarello M, et al. The role of the immunosuppressive microenvironment in acute myeloid leukemia development and treatment. Expert Rev Hematol. 2014;7(6):807–818.
- Chen Y, Zhou Y, Chen P, et al. Excessive expressions of T cell activation markers in pediatric immune thrombocytopenia. Thromb Res. 2019;180:1–9.
- Klion AD, Law MA, Riemenschneider W, et al. Familial eosinophilia: a benign disorder? Blood. 2004;103(11):4050–4055.
- Papadaki HA, Stamatopoulos K, Damianaki A, et al. Activated T-lymphocytes with myelosuppressive properties in patients with chronic idiopathic neutropenia. Br J Haematol. 2005;128(6):863–876.
- Yang C, Zhu X, Zhang T, et al. EBV-HLH children with reductions in CD4+ T cells and excessive activation of CD8+ T cells. Pediatr Res. 2017;82(6):952–957.
- Reddy M, Eirikis E, Davis C, et al. Comparative analysis of lymphocyte activation marker expression and cytokine secretion profile in stimulated human peripheral blood mononuclear cell cultures: an in vitro model to monitor cellular immune function. J Immunol Methods. 2004;293(1–2):127–142.
- van de Donk N, Richardson PG, Malavasi F. CD38 antibodies in multiple myeloma: back to the future. Blood. 2018;131(1):13–29.
- Krejcik J, Casneuf T, Nijhof IS, et al. Daratumumab depletes CD38+ immune regulatory cells, promotes T-cell expansion, and skews T-cell repertoire in multiple myeloma. Blood. 2016;128(3):384–394.
- Chaturvedi V, Marsh RA, Zoref-Lorenz A, et al. T-cell activation profiles distinguish hemophagocytic lymphohistiocytosis and early sepsis. Blood. 2021;137(17):2337–2346.
- Van den Hove LE, Vandenberghe P, Van Gool SW, et al. Peripheral blood lymphocyte subset shifts in patients with untreated hematological tumors: evidence for systemic activation of the T cell compartment. Leuk Res. 1998;22(2):175–184.
- Zhou Y, Zhang Y, Johnson A, et al. Combined CD25, CD64, and CD69 biomarker panel for flow cytometry diagnosis of sepsis. Talanta. 2019;191:216–221.
- Miki-Hosokawa T, Hasegawa A, Iwamura C, et al. CD69 controls the pathogenesis of allergic airway inflammation. J Immunol. 2009;183(12):8203–8215.
- Zhigarev D, Varshavsky A, MacFarlane A, et al. Lymphocyte exhaustion in AML patients and impacts of HMA/venetoclax or intensive chemotherapy on their biology. Cancers. 2022;14(14):3352.
- St Paul M, Ohashi PS. The roles of CD8(+) T cell subsets in antitumor immunity. Trends Cell Biol. 2020;30(9):695–704.
- Xu L, Liu L, Yao D, et al. PD-1 and TIGIT Are highly Co-expressed on CD8(+) T cells in AML patient bone marrow. Front Oncol. 2021;11:686156.
- Brauneck F, Haag F, Woost R, et al. Increased frequency of TIGIT(+)CD73-CD8(+) T cells with a TOX(+) TCF-1low profile in patients with newly diagnosed and relapsed AML. Oncoimmunology. 2021;10(1):1930391.
- Le Dieu R, Taussig DC, Ramsay AG, et al. Gribben JG: peripheral blood T cells in acute myeloid leukemia (AML) patients at diagnosis have abnormal phenotype and genotype and form defective immune synapses with AML blasts. Blood. 2009;114(18):3909–3916.
- Ohue Y, Nishikawa H. Regulatory T (Treg) cells in cancer: Can Treg cells be a new therapeutic target?. Cancer Sci. 2019;110(7):2080–2089.
- Williams P, Basu S, Garcia-Manero G, et al. The distribution of T-cell subsets and the expression of immune checkpoint receptors and ligands in patients with newly diagnosed and relapsed acute myeloid leukemia. 2019;125(9):1470–1481.
- Jia B, Wang L, Claxton DF, et al. Bone marrow CD8 T cells express high frequency of PD-1 and exhibit reduced anti-leukemia response in newly diagnosed AML patients. Blood Cancer J. 2018;8(3):34.
- Pyzer AR, Stroopinsky D, Rajabi H, et al. MUC1-mediated induction of myeloid-derived suppressor cells in patients with acute myeloid leukemia. Blood. 2017;129(13):1791–1801.
- Knaus HA, Berglund S, Hackl H, et al. Signatures of CD8+ T cell dysfunction in AML patients and their reversibility with response to chemotherapy. JCI Insight. 2018;3(21):e120974.