ABSTRACT
Background
Acute myeloid leukemia (AML) is a common hematological malignancy. Circular RNAs (circRNAs) are newly discovered endogenous non-coding RNAs that play important roles in regulating gene expression in cancer biology. The aim of this study was to identify novel prognostic and therapeutic targets associated with AML.
Methods
The expression levels of circRNA Plexin B2 (circPLXNB2), microRNA-654-3p (miR-654-3p) and cyclin D1 protein (CCND1) mRNA were detected by real-time quantitative polymerase chain reaction (qRT-PCR). The stability of circPLXNB2 was determined by RNase R and Actinomycin D assays. Cell counting kit-8 (CCK-8) and 5′-ethynyl-2′-deoxyuridine (EDU) assays were used to detect cell proliferation. Western blot was used to detect protein content. Cell apoptosis and cell cycle distribution were detected by flow cytometry. The relationship between miR-654-3p and circPLXNB2 or CCND1 was confirmed by dual-luciferase reporter assay.
Results
CircPLXNB2 was highly expressed in AML patients and cells, and circPLXNB2 was more stable than linear PLXNB2. Knockdown of circPLXNB2 affected the proliferation, apoptosis and cell cycle distribution of AML cells. CircPLXNB2 acted as a sponge for miR-654-3p and affected the progression of AML cells by targeting miR-654-3p. CCND1 was targeted by miR-654-3p, and miR-654-3p suppressed AML progression by targeting CCND1. CircPLXNB2 regulated CCND1 expression through sponging miR-654-3p.
Conclusion
In conclusion, circPLXNB2 was highly expressed in AML patients and cells and modulated tumor progression by regulating the circPLXNB2/miR-654-3p/CCND1 axis, suggested that circPLXNB2 might be a new therapeutic target for AML treatment.
KEYWORDS:
Introduction
Acute myeloid leukemia (AML) is a cancer characterized by a malignant clonal proliferation of myeloid hematopoietic stem cells [Citation1,Citation2]. Despite intensive research, AML is the most prevalent acute leukemia among adults with a high mortality rate [Citation3]. The five-year survival rate of AML patients is less than 24% because AML patients have poor response to treatment and unfavorable prognosis with numerous complications [Citation4,Citation5]. Recently, genetic and epigenetic alterations have been shown to participate in AML development [Citation6,Citation7]. Epigenetic regulators, including circular RNAs (circRNAs), are under intensive investigation at present [Citation8]. Uncovering the actions of these epigenetic regulators would provide the basis of targeted therapies.
CircRNAs are endogenous non-coding RNAs (ncRNAs) produced by back-splicing of pre-mRNAs [Citation9,Citation10]. CircRNAs play important roles in many physiological and biochemical processes through working as sponges of microRNAs (miRNAs) [Citation11,Citation12]. MiRNAs regulate gene expression by silencing or degrading their target mRNA molecules, thus further influencing various cellular processes [Citation13]. Dysregulation of circRNAs has been found in cancer biology, and dysregulated circRNAs operate as sponges of miRNAs to actively participate in the development of many cancers, including AML [Citation14,Citation15]. For example, circRNA-DLEU2 can accelerate the progression of AML by upregulating PRKACB by acting as a sponge of miR-496 [Citation16]. Circ_0040823 acts as a tumor suppressor in AML by mediating the miR-516b/PTEN axis [Citation17]. Our analysis showed that the expression change of circRNA Plexin B2 (circPLXNB2, ID: hsa_circ_0001257) was closely related to miR-654-3p.
Therefore, we studied the interaction between circPLXNB2 and miR-654-3p in AML, providing new ideas for the study of AML molecular mechanism and prevention.
Materials and methods
Sample collection
In this study, blood samples were collected from AML patients (n = 51) and healthy donors (n = 51) at The First Affiliated Hospital of Hainan Medical University. All patients passed pathological examination. After removal, clinical samples were quickly placed into liquid nitrogen and then transferred to the refrigerator at −80°C for storage. This experiment had been approved by The First Affiliated Hospital of Hainan Medical University Ethics Committee. All patients signed written informed consent.
Cell culture and transfection
The human AML cell lines HL-60 (promyelocytes, differentiated), Kasumi-1 (promyelocytes, differentiated) and 293T cells were purchased from Procell (Wuhan, China). Non-cancer human bone marrow stromal HS-5 cells (ATCC, Manassas, VA, USA) were cultured in 10% FBS DMEM (Gibco, Life Technologies, Carlsbad, CA, USA) enriched with 2% L-Glutamine (Gibco). AML cells were maintained in 10% FBS RPMI-1640 medium (Gibco) and 293T cells were propagated in 10% FBS DMEM (Gibco) in an incubator with 5% CO2 at 37°C.
siRNA targeting circPLXNB2 (si-circPLXNB2), miR-654-3p inhibitor, miR-654-3p mimic, cyclin D1 (CCND1) pcDNA vector (pcDNA-CCND1), circPLXNB2 expression plasmid (oe-circPLXNB2), and their negative controls (si-NC, inhibitor NC, mimic NC, pcDNA, vector) were acquired from Ribobio (Guangzhou, China). The lipofectamine 2000 (Invitrogen, Carlsbad, CA, USA) was applied for transfection. HL-60 and Kasumi-1 cells (1 × 106/well) were seeded in 24-well dishes 18 h before transfection with 100 nM siRNA or/and 200 ng plasmid or/and 50 nM miRNA mimic or/and 50 nM miRNA inhibitor. We harvested the transfected cells after 24 h for the subsequent assays.
Quantitative real-time PCR (qRT-PCR)
The isolation of total RNA was carried out via TRIzol reagent (Invitrogen). Then treatment with RNase R (Beyotime, Shanghai, China) was conducted at 37°C for 30 min. Whereafter, cDNAs were generated using the PrimeScript™ RT reagent Kit (TaKaRa, Dalian, China) with random hexamers (for circPLXNB2 and mRNAs) or microScript miRNA cDNA Synthesis Kit (Norgen Biotek, Thorold, ON, Canada) with stem-loop primers (for miR-654-3p). Then, the synthesized cDNA template was quantified on ABI7300 with SYBR Green Master Mix II (TaKaRa) with designed primers. The 2−ΔΔCT method was utilized to quantify the relative expression levels of circPLXNB2, CCND1 mRNA and miR-654-3p with GAPDH or U6 as an internal reference. AML cells were treated with 2 μg/mL Actinomycin D (Beyotime), followed by implement of qRT-PCR. The primer sequences were listed in .
Table 1. Primers sequences used for PCR.
Cell counting kit-8 (CCK-8) assay
CCK-8 cell viability assay was used to evaluate cell proliferation ability. Transfected AML cells were cultured in medium (96-well plates) in a CO2 incubator at 37°C for 24 h and then incubated with 10 μL CCK8 solution (GLPBIO, Shanghai, China) at indicated times for addition 2 h, and the absorbance was tested at 450 nm.
5-Ethynyl-2′-deoxyuridine (EDU) assay
The EDU assay was conducted to assess cell proliferation using EdU cell proliferation kit (Ribobio). Briefly, the transfected cells were treated with 50 μM EdU solution for 2 h in 96-well plates (4 × 103–1 × 105/well), and then immobilized with 4% paraformaldehyde for 30 min. After being stained with the nuclear dye DAPI for 30 min, the EdU-positive cells in five random fields per well were imaged and counted.
Flow cytometry
For apoptosis and cell cycle analyses, the transfected cells were collected and suspended in 300 μL binding buffer. For apoptosis analysis, the transfected cells were reacted with 5 μL Annexin V-FITC and 5 μL PI (Abcam, Cambridge, MA, USA) in the dark for 5–15 min at 37°C. For cycle distribution assay, the transfected cells were fixed with 70% ethanol overnight, followed by staining with PI (BD Biosciences). Finally, 1 × 105 events were analyzed by the FACS Aria III flow cytometer (BD Biosciences) with FlowJo software (TreeStar, Ashland, OR, USA). The cells were considered as early (Annexin V+/PI−) and late (Annexin V+/PI+) apoptotic cells.
Western blot
Proteins were isolated using the protein extraction kit with phosphatase and protease inhibitors (Beyotime). Then, total proteins were segregated by 10% SDS-PAGE gels, followed by the transference to PVDF membranes (Bio-Rad, CA, USA). Thereafter, primary antibodies against PCNA (ab29, 1/1000, Abcam), Bcl-2 (ab3212, 1/1000, Abcam), Bax (ab32503, 1/2000, Abcam), β-actin (ab6276, 1/1000, Abcam) and Cyclin D1 (ab40754, 1/1000, Abcam) were applied to incubate with the membranes overnight at 4°C, and the membranes were probed with secondary antibodies conjugated-HRP (Sangon, Shanghai, China) for 2 h at 37°C. Protein bands were assayed by ECL Substrate Kit (Bio-Rad). The Image Lab software (Bio-Rad) was used for densitometric analysis of western blots.
Dual-luciferase reporter assay
The wild-type and mutant sequences of circPLXNB2 and CCND1 3′UTR with miR-654-3p binding sites or mutant target sites were individually generated and introduced into the psiCHECK2 luciferase reporter vector (Promega, Madison, WI, USA). Then, the above luciferase reporter vectors and mimic NC or miR-654-3p mimic were co-transfected into 293T cells, and luciferase activity was detected after 48 h.
Statistical analysis
All quantitative experiments were performed with at least three independent biological repeats in triplicate. All data were expressed as mean ± standard deviation (SD). Pearson’s correlation analysis was utilized for correlation expression. Statistical analysis was done using one-way ANOVA with post hoc Tukey’s multiple comparisons test (for multiple groups) or unpaired Student’s t-test (two-tailed, for two groups). P < 0.05 was considered as statistically significant.
Results
CircPLXNB2 was highly expressed in AML patients and cells
We first used qRT-PCR to determine the expression of circPLXNB2 in human AML. CircPLXNB2 expression was increased in AML patients compared with healthy controls ((A)). Consistently, circPLXNB2 was upregulated in AML cells (HL-60 and Kasumi-1) compared with normal HS-5 cells ((B)). CircPLXNB2 (hsa_circ_0001257) is located at chr22:50713540-50713723, with the length of 183 nt, which is generated by back-splicing of PLXNB2 pre-mRNA ((C)). To characterize circPLXNB2, we adopted RNase R and actinomycin D assays. RNase R assays showed that circPLXNB2, not linear PLXNB2 content, was tolerant to RNase R digestion ((D)), indicating that circPLXNB2 was stable in AML cells. Actinomycin D treatment decreased linear PLXNB2 content, but failed to affect circPLXNB2 in HL-60 and Kasumi-1 cells ((E)), indicating that circPLXNB2 was a true circular transcript. These results showed that circPLXNB2 was highly expressed in AML patients and cells.
Figure 1. CircPLXNB2 was highly expressed in AML patients and cells. (A) CircPLXNB2 expression in healthy donors (n = 51) and AML patients (n = 51) was detected by qRT-PCR. (B) qRT-PCR was used to detect the expression of circPLXNB2 in HS-5, HL-60 and Kasumi-1 cells. n = 3 independent biological replicates. (C) CircPLXNB2 was located in chr22: 50713540-50713723, with a length of 183 nt. (D) CircPLXNB2 and its matching linear mRNA expression in AML cells (HL-60 and Kasumi-1 cells) treated with or without RNase R were detected by qRT-PCR. n = 3 independent biological replicates. (E) CircPLXNB2 and its matching linear mRNA expression in AML cells (HL-60 and Kasumi-1 cells) treated with actinomycin D were detected. n = 3 independent biological replicates. *P < 0.05 vs. healthy controls, HS-5 cells, RNase R− or actinomycin D−.
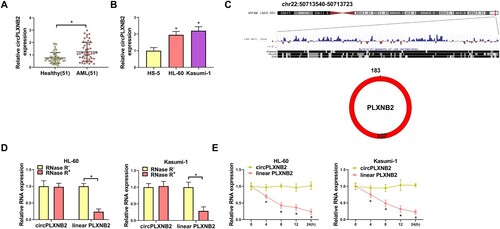
Knockdown of circPLXNB2 affected the proliferation, apoptosis and cell cycle distribution of AML cells
To study the influence of circPLXNB2 in AML, we conducted the phenotypic silencing with si-circPLXNB2. QRT-PCR analysis showed that si-circPLXNB2 significantly reduced the expression of circPLXNB2 in AML cells ((A)). CCK8 and EDU assays showed that circPLXNB2 knockdown suppressed the proliferation ability of AML cells ((B,C)). Flow cytometry showed that the apoptosis rate of AML cells was increased by circPLXNB2 depletion ((D)). Moreover, circPLXNB2 depletion promoted the percentage of the G0/G1 phase cells ((E)), indicating that circPLXNB2 depletion repressed cell cycle progression. Besides, circPLXNB2 depletion led to decrease in PCNA and Bcl-2 levels and increased BAX protein level in AML cells ((F)). All the data showed that circPLXNB2 knockdown hindered the proliferation and cell cycle progression and drove the apoptosis of AML cells.
Figure 2. Knockdown of circPLXNB2 affected the proliferation, apoptosis and cell cycle distribution of AML cells. (A) qRT-PCR was performed to detect the transfection efficiency of circPLXNB2 siRNA in AML cells. (B, C) CCK8 and EDU analyses for the proliferation of AML cells transfected with si-circPLXNB2. (D) Apoptosis of AML cells with circPLXNB2 knockdown was detected by flow cytometry. (E) Flow cytometry was used to detect cell cycle distribution of AML cells with circPLXNB2 knockdown. (F) Levels of PCNA, Bcl-2 and Bax were examined by Western blot in AML cells with circPLXNB2 knockdown. n = 3 independent biological replicates. *P < 0.05 vs. si-NC.
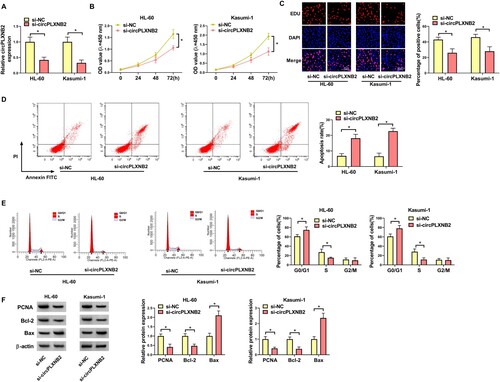
CircPLXNB2 acted as a sponge for miR-654-3p
We then explored the mechanism of circPLXNB2’s function in affecting AML cell phenotypes. Using the Starbase3.0 online database, miR-654-3p was predicted to bind to circPLXNB2 ((A)). Dual-luciferase assays showed that miR-654-3p mimic resulted in suppressed luciferase activity of circPLXNB2 wt in 293T cells, while circPLXNB2 mut was tolerant to miR-654-3p ((B)). In AML patients, miR-654-3p expression was decreased ((C)) and negatively correlated with circPLXNB2 expression ((D)). QRT-PCR also showed that miR-654-3p expression was decreased in AML cells compared with normal HS-5 cells ((E)). To evaluate the regulation of circPLXNB2 on miR-654-3p expression, we manipulated circPLXNB2 expression in AML cells with oe-circPLXNB2 or si-circPLXNB2. Overexpression of circPLXNB2 upon oe-circPLXNB2 introduction, validated by qRT-PCR ((F)), repressed miR-654-3p expression, while circPLXNB2 knockdown led to augmentation in miR-654-3p expression ((G)). These results together showed that circPLXNB2 acted as a sponge for miR-654-3p.
Figure 3. CircPLXNB2 acted as a sponge for miR-654-3p. (A) miR-654-3p was predicted to be the target of circPLXNB2 by using the Starbase3.0 online database. (B) Dual-luciferase reporter assay was used to confirm whether circPLXNB2 combined with miR-654-3p. n = 3 independent biological replicates. (C) qRT-PCR detection of miR-654-3p in healthy donors (n = 51) and AML patients (n = 51). (D) Pearson’s correlation analysis was performed to assess the relationship between circPLXNB2 and miR-654-3p expression in AML patients. n = 51. (E) qRT-PCR was used to detect the expression of miR-654-3p in HS-5, HL-60 and Kasumi-1 cells. n = 3 independent biological replicates. (F) qRT-PCR detection of circPLXNB2 in HL-60 and Kasumi-1 cells transfected with vector or oe-circPLXNB2. n = 3 independent biological replicates. (G) qRT-PCR detection of miR-654-3p in HL-60 and Kasumi-1 cells transfected with vector, oe-circPLXNB2, si-NC, or si-circPLXNB2. n = 3 independent biological replicates. *P < 0.05 vs. healthy controls, HS-5 cells, mimic NC, vector or si-NC.
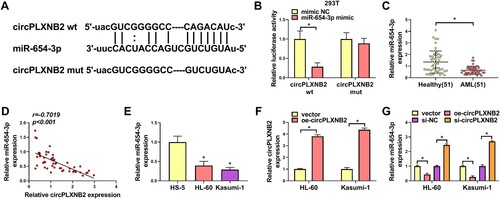
CircPLXNB2 knockdown affected the proliferation, apoptosis and cell cycle distribution of AML cells by targeting miR-654-3p
QRT-PCR showed that transfection of miR-654-3p inhibitor decreased the expression of miR-654-3p in AML cells ((A)). To directly study whether the function of circPLXNB2 knockdown in affecting AML cell phenotypes is due to miR-654-3p upregulation, we introduced miR-654-3p inhibitor into AML cells after transfection by si-circPLXNB2. MiR-654-3p inhibitor could reduce miR-654-3p expression induced by circPLXNB2 knockdown in AML cells ((B)). CCK8 and EDU assays showed that miR-654-3p inhibitor could restore the proliferation ability of AML cells repressed by circPLXNB2 knockdown ((C,D)). Flow cytometry showed that miR-654-3p inhibitor could abate circPLXNB2 knockdown-driven apoptosis increase of AML cells ((E)). Moreover, miR-654-3p inhibitor could abolish circPLXNB2 knockdown-mediated cell cycle arrest ((F)). Western blot showed that miR-654-3p inhibitor could abolish circPLXNB2 knockdown-mediated the decrease in PCNA and Bcl-2 levels and the increase of BAX protein level in AML cells ((G)). These results together showed that circPLXNB2 knockdown affected the proliferation, apoptosis and cell cycle distribution of AML cells by targeting miR-654-3p.
Figure 4. CircPLXNB2 knockdown affected the progression of AML cells by targeting miR-654-3p. (A) Transfection efficiency of miR-654-3p inhibitor in AML cells was detected by qRT-PCR. (A-G) HL-60 and Kasumi-1 cells were co-transfected with si-circPLXNB2 and/or miR-654-3p inhibitor. (B) The expression level of miR-654-3p was detected by qRT-PCR. (C, D) Proliferation of transfected cells was detected by CCK8 and EDU assays. (E) Apoptosis of transfected cells was detected by flow cytometry. (F) The cell cycle distribution of transfected cells was determined by flow cytometry. (G) Levels of PCNA, Bcl-2 and Bax were examined by Western blot in AML cells. n = 3 independent biological replicates. *P < 0.05 vs. inhibitor NC, si-NC or si-circPLXNB2 + inhibitor NC.
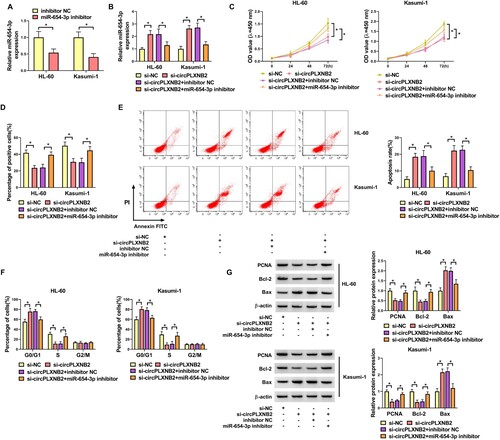
MiR-654-3p directly targeted CCND1
In order to identify the downstream effectors of miR-654-3p, we used the Starbase3.0 online database and found that miR-654-3p contained target binding sites to CCND1 3′UTR ((A)). Dual-luciferase assays showed that miR-654-3p mimic only repressed the luciferase activity of CCND1 3'UTR wt in 293 T cells ((B)). CCND1 mRNA was highly expressed in AML patients ((C)). Expression correlation analysis showed that CCND1 mRNA level was negatively correlated with miR-654-3p expression in AML patients ((D)). Western blot showed that CCND1 protein expression was increased in AML cells compared with normal HS-5 cells ((E)). Furthermore, circPLXNB2 knockdown led to downregulation in CCND1 protein content, whereas miR-654-3p inhibitor could reverse the decline ((F)). All these results showed miR-654-3p directly targeted CCND1, and circPLXNB2 could regulate CCND1 expression through miR-654-3p.
Figure 5. MiR-654-3p directly targeted CCND1. (A) miR-654-3p was predicted using the Starbase3.0 online database to bind with CCND1 3′UTR wt but not with CCND1 3′UTR mut. (B) Dual-luciferase reporter assay was used to confirm whether CCND1 could combine with miR-654-3p. n = 3 independent biological replicates. (C) qRT-PCR was used to detect CCND1 mRNA expression in healthy controls (n = 51) and AML patients (n = 51). (D) Pearson’s correlation analysis was performed to assess the relationship between CCND1 mRNA and miR-654-3p expression in AML patients. n = 51. (E) CCND1 protein was detected by Western blot in HS-5, HL-60 and Kasumi-1 cells. n = 3 independent biological replicates. (F) HL-60 and Kasumi-1 cells were co-transfected with si-circPLXNB2 and/or miR-654-3p inhibitor, and the content of CCND1 protein in transfected cells was detected. n = 3 independent biological replicates. *P < 0.05 vs. healthy controls, HS-5 cells, mimic NC, si-NC or si-circPLXNB2 + inhibitor NC.
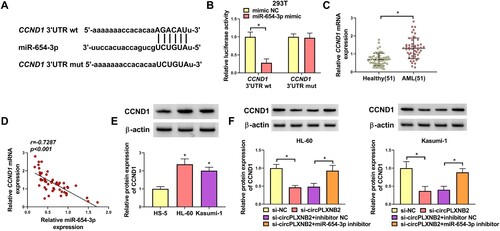
MiR-654-3p suppressed AML progression by targeting CCND1
Western blot showed that CCND1 expression plasmid (pcDNA-CCND1) led to an increase in CCND1 protein content in AML cells ((A)). QRT-PCR showed that miR-654-3p mimic increased miR-654-3p level in AML cells ((B)). To further validate the implication of the miR-654-3p/CCND1 axis in AML cell phenotypes, we transfected miR-654-3p mimic alone or together with pcDNA-CCND1 into AML cells. Western blot showed that co-transfection of pcDNA-CCND1 reversed CCND1 protein decrease caused by miR-654-3p mimic ((C)). CCK8 and EDU assays showed that re-expression of CCND1 protein could reverse miR-654-3p-mediated decrease in proliferation of AML cells ((D,E)). Flow cytometry showed that re-expression of CCND1 protein could inhibit apoptosis increase induced by miR-654-3p mimic ((F)). Also, re-expression of CCND1 protein could inhibit cell cycle arrest induced by miR-654-3p mimic ((G)). Western blot showed that elevated miR-654-3p expression decreased PCNA and Bcl-2 protein levels and promoted Bax protein expression in AML cells, while re-expression of CCND1 protein could abolish these effects ((H)). These results showed that miR-654-3p suppressed AML cell phenotypes by targeting CCND1.
Figure 6. MiR-654-3p suppressed AML progression by targeting CCND1. (A) Western blot was used to detect the expression efficiency of CCND1 protein overexpression vector in AML cells. (B) qRT-PCR was used to detect the expression efficiency of miR-654-3p overexpression vector in AML cells. (C–H) Mimic NC, miR-654-3p mimic, miR-654-3p mimic + pcDNA and miR-654-3p mimic + pcDNA-CCND1 were transfected into AML cells. (C) The expression of CCND1 protein in transfected cells was detected. (D, E) Proliferation of transfected cells was detected by CCK8 and EDU assays. (F) Apoptosis of transfected cells was detected by flow cytometry. (G) The cell cycle distribution of transfected cells was determined by flow cytometry. (F) Levels of PCNA, Bcl-2 and Bax were examined by Western blot in AML cells. n = 3 independent biological replicates. *P < 0.05 vs. pcDNA, mimic NC, miR-654-3p mimic + pcDNA.
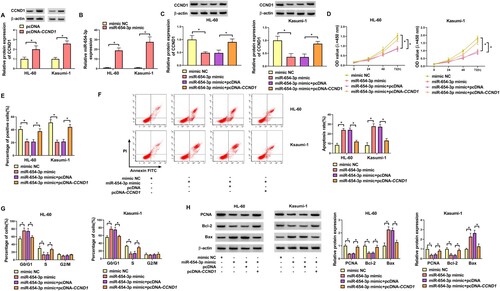
Discussion
AML is a malignant clonal disease with multiple prognostic problems [Citation1,Citation18]. As modern medicine advances, about half of patients with AML meet with relapses or complications, despite advances in treatment [Citation19,Citation20]. Therefore, we need to identify new therapeutic targets and understand their pathogenesis. Some studies have suggested that circRNAs are implicated in AML progression [Citation21,Citation22]. Elevated circPLXNB2 levels have been revealed to be related to the prognosis of AML patients [Citation23]. Our study further investigated the precise role of circPLXNB2 in AML. In our work, circPLXNB2 expression was discovered to be up-regulated in AML, and circPLXNB2 silencing reduced the proliferation ability and promoted apoptosis in AML cells. Our findings also showed that circPLXNB2 regulated the expression of CCND1 protein through miR-654-3p, providing further evidence for the role of circPLXNB2 in AML.
MiR-654-3p has established a tumor inhibitory role in various human cancers, such as colorectal cancer, sinonasal squamous cell carcinoma and lung cancer [Citation24–26]. MiR-654-3p is demonstrated to actively participate in AML progression [Citation27]. Here we uncovered, for the first time, that circPLXNB2 functioned as a miR-654-3p sponge. Moreover, circPLXNB2 targeted miR-654-3p to regulate the progression of AML. Similarly, circ_0079480 contributes to AML development by sponging miR-654-3p [Citation27].
CCND1 is located in chromosome 11q13 and encodes cyclin D1 protein, which belongs to the highly conserved cyclin family and regulates CDK kinase [Citation28]. Studies have shown that CCND1 protein enhanced cytarabine sensitivity in AML cells [Citation29]. CCND1 protein can promote cell cycle progression by Kit-Ras-Raf-MEK-ERK pathway [Citation30]. In this study, we first found that miR-654-3p targeted CCND1, and the re-expression of CCDN1 protein reversed the function of miR-654-3p. Previous work showed that in AML, miR-655-3p regulated cancer cell sensitivity to cytarabine by targeting CCND1 [Citation29]. Furthermore, circPLXNB2 regulated CCND1 protein expression through sponging miR-654-3p.
Such analysis is limited at present by the lack of in vivo research using AML animal models. In this study, the precise function of circPLXNB2 was only studied in two strains (HL-60 and Kasumi-1) of AML cells, which could not fully and accurately reflect its role in AML pathogenesis. In further research, more cell lines will be used. Due to the small clinical sample size, whether circPLXNB2 can be used as a biomarker for the diagnosis of AML remains to be resolved. In this study, siRNA targeting circPLXNB2 appears to represent a promising anti-AML agent that blocks cancer cell proliferation and promotes cell apoptosis. We envision that the siRNA may to be starting point for the development of circRNA-based therapies against AML.
In short, our study confirmed that circPLXNB2 acted as a competing endogenous RNA (ceRNA) for miR-654-3p to up-regulate CCND1 protein expression, thereby promoting AML process (). Our study provides new evidence for developing circPLXNB2 as a promising target for AML treatment.
Figure 7. Graphical representation of the circPLXNB2/miR-654-3p/CCND1 axis in AML progression. CircPLXNB2 is derived from the back-splicing of PLXNB2 pre-mRNA and acts as the ceRNA of miR-654-3p to up-regulate CCND1 protein expression, thereby promoting the proliferation and inhibiting the apoptosis in AML cells.
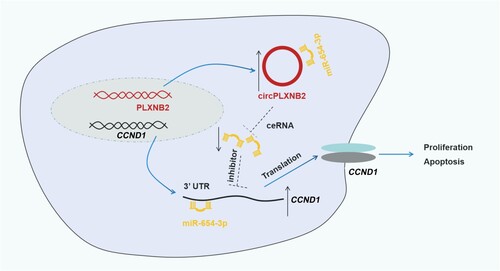
Ethics approval and consent to participate
Written informed consent was obtained from patients with approval by the Institutional Review Board in The First Affiliated Hospital of Hainan Medical University.
Disclosure statement
No potential conflict of interest was reported by the author(s).
Data availability statement
Please contact the correspondence author for the data request.
References
- Gregory TK, Wald D, Chen Y, et al. Molecular prognostic markers for adult acute myeloid leukemia with normal cytogenetics. J Hematol Oncol. 2009;2:23. DOI:10.1186/1756-8722-2-23
- Shih AH, Abdel-Wahab O, Patel JP, et al. The role of mutations in epigenetic regulators in myeloid malignancies. Nat Rev Cancer. 2012;12(9):599–612. DOI:10.1038/nrc3343
- De Kouchkovsky I, Abdul-Hay M. Acute myeloid leukemia: a comprehensive review and 2016 update. Blood Cancer J. 2016;6(7):e441. DOI:10.1038/bcj.2016.50
- Crossnohere NL, Richardson DR, Reinhart C, et al. Side effects from acute myeloid leukemia treatment: results from a national survey. Curr Med Res Opin. 2019;35(11):1965–1970. DOI:10.1080/03007995.2019.1631149
- Short NJ, Konopleva M, Kadia TM, et al. Advances in the treatment of acute myeloid leukemia: new drugs and new challenges. Cancer Discov. 2020;10(4):506–525. DOI:10.1158/2159-8290.CD-19-1011
- Owattanapanich W, Herzig J, Jahn N, et al. Genetic alterations in Thai adult patients with acute myeloid leukemia and myelodysplastic syndrome-excess blasts detected by next-generation sequencing technique. Ann Hematol 2021;100(8):1983–1993.
- Mehdipour P, Santoro F, Minucci S. Epigenetic alterations in acute myeloid leukemias. FEBS J 2015;282(9):1786–1800.
- Lux S, Blätte TJ, Gillissen B, et al. Deregulated expression of circular RNAs in acute myeloid leukemia. Blood Adv. 2021;5(5):1490–1503.
- Vo JN, Cieslik M, Zhang Y, et al. The landscape of circular RNA in cancer. Cell. 2019;176(4):869–881.e13. DOI:10.1016/j.cell.2018.12.021
- Shang Q, Yang Z, Jia R, et al. The novel roles of circRNAs in human cancer. Mol Cancer. 2019;18(1):6. DOI:10.1186/s12943-018-0934-6
- Yan B, Zhang Y, Liang C, et al. Stem cell-derived exosomes prevent pyroptosis and repair ischemic muscle injury through a novel exosome/circHIPK3/ FOXO3a pathway. Theranostics. 2020;10(15):6728–6742.
- Awan FM, Yang BB, Naz A, et al. The emerging role and significance of circular RNAs in viral infections and antiviral immune responses: possible implication as theranostic agents. RNA Biol. 2021;18(1):1–15.
- Shah MY, Ferrajoli A, Sood AK, et al. Microrna therapeutics in cancer – an emerging concept. EBioMedicine. 2016;12:34–42. DOI:10.1016/j.ebiom.2016.09.017
- Wang J, Li H, Liang Z. circ-MYBL2 Serves As A Sponge For miR-361-3p Promoting Cervical Cancer Cells Proliferation And Invasion. Onco Targets Ther. 2019;12:9957–9964. DOI:10.2147/OTT.S218976
- Li X, Yang B, Ren H, et al. Hsa_circ_0002483 inhibited the progression and enhanced the Taxol sensitivity of non-small cell lung cancer by targeting miR-182-5p. Cell Death Dis. 2019;10(12):953. DOI:10.1038/s41419-019-2180-2
- Wu DM, Wen X, Han XR, et al. Role of circular RNA DLEU2 in human acute myeloid leukemia. Mol Cell Biol 2018;38(20). e0037522.
- Wang N, Yang B, Jin J, et al. Circular RNA circ_0040823 inhibits the proliferation of acute myeloid leukemia cells and induces apoptosis by regulating miR-516b/PTEN. J Gene Med. 2022;24(3):e3404.
- Riva L, Luzi L, Pelicci PG. Genomics of acute myeloid leukemia: the next generation. Front Oncol. 2012;2:40.
- Tabe Y, Konopleva M. Role of microenvironment in resistance to therapy in AML. Curr Hematol Malig Rep. 2015;10(2):96–103. DOI:10.1007/s11899-015-0253-6
- Ishikawa F, Yoshida S, Saito Y, et al. Chemotherapy-resistant human AML stem cells home to and engraft within the bone-marrow endosteal region. Nat Biotechnol. 2007;25(11):1315–1321. DOI:10.1038/nbt1350
- Sun YM, Wang WT, Zeng ZC, et al. circMYBL2, a circRNA from MYBL2, regulates FLT3 translation by recruiting PTBP1 to promote FLT3-ITD AML progression. Blood. 2019;134(18):1533–1546.
- Wang X, Jin P, Zhang Y, et al. CircSPI1 acts as an oncogene in acute myeloid leukemia through antagonizing SPI1 and interacting with microRNAs. Cell Death Dis. 2021;12(4):297. DOI:10.1038/s41419-021-03566-2
- Lin L, Wang Y, Bian S, et al. A circular RNA derived from PLXNB2 as a valuable predictor of the prognosis of patients with acute myeloid leukaemia. J Transl Med. 2021;19(1):123. DOI:10.1186/1479-5876-12-123
- Zhang H, Shen Z, Zhou Y, et al. Downregulation of miR-654-3p in colorectal cancer indicates poor prognosis and promotes cell proliferation and invasion by targeting SRC. Front Genet. 2020;11:577948.
- Xiong J, Xing S, Dong Z, et al. Yang P: miR-654-3p suppresses cell viability and promotes apoptosis by targeting RASAL2 in non-small-cell lung cancer. Mol Med Rep. 2021;23(2):124.
- Cui X, Yang Y, Yan A. MiR-654-3p constrains proliferation, invasion, and migration of sinonasal squamous cell carcinoma via CREB1/PSEN1 regulatory axis. Front Genet. 2021;12:799933.
- Hu Q, Gu Y, Chen S, et al. Hsa_circ_0079480 promotes tumor progression in acute myeloid leukemia via miR-654-3p/HDGF axis. Aging (Albany NY). 2020;13(1):1120–1131.
- UniProt C. UniProt: a worldwide hub of protein knowledge. Nucl Acid Res. 2019;47(D1):D506–D515.
- Zhang B, Sun YF, Zhang XM, et al. TUG1 weakens the sensitivity of acute myeloid leukemia cells to cytarabine by regulating miR-655-3p/CCND1 axis. Eur Rev Med Pharmacol Sci. 2020;24(9):4940–4953.
- Amos-Landgraf J, Franklin C, Godfrey V, et al. The mutant mouse resource and research center (MMRRC): the NIH-supported national public repository and distribution archive of mutant mouse models in the USA. Mamm Genome. 2021;33(1):203–212.