ABSTRACT
Background
miR-454-3p is considered to have a crucial role in cancer progression, but the potential involvement in acute myeloid leukemia (AML) remains unclear.
Methods
Expression of miR-454-3p and ZEB2 mRNA and protein were quantified in AML cell lines. Cells were transfected with miR-454-3p inhibitor or mimic and cell growth was assessed by colony formation and CCK-8 assays and the cell cycle, apoptosis and autophagy were investigated by Western blotting, flow cytometry, immunofluorescence and 3-methyladenine (3-MA) treatment.
Results
miR-454-3p expression was attenuated in AML cells. miR-454-3p overexpression attenuated cell growth and stimulated cell cycle arrest, apoptosis and autophagy. Dual-luciferase reporter assays and bioinformatics analysis showed that AML progression was inhibited when miR-454-3p regulated ZEB2, an effect confirmed by rescue assays. 3-MA reduced the autophagy-inducing effect of ZEB2 knockdown and indicated that autophagy induced apoptosis. miR-454-3p downregulated p-mTOR/p-AKT levels in AML cells.
Conclusion
The novel role of miR-454-3p as a tumor inhibitor in AML via regulation of the ZEB2/AKT/mTOR axis was demonstrated, indicating miR-454-3p as a potential new molecular target for AML.
1. Introduction
Acute myeloid leukemia (AML) is a complex and heterogeneous hematological malignancy with characteristics of abnormal cell proliferation, inhibition of normal hematopoiesis and high mortality [Citation1]. Recent progress has resulted in therapeutic advances, including hematopoietic stem-cell transplantation and chemotherapy [Citation2,Citation3]. However, further elucidation of the molecular mechanisms underlying AML pathophysiology is required to enable the development of optimal treatments.
Many microRNAs (miRNAs) have been shown to be differentially expressed in normal and cancerous tissue and involved in human cancer progression [Citation4]. Differential expression of miRNAs may be related to the location of relevant genes in specific cancer-associated genomic regions with impacts on epigenetic mechanisms [Citation5]. MicroRNAs are known to exert anti-tumor effects on AML cells [Citation6,Citation7] and miR-454-3p has been implicated in various tumor types, such as breast cancer metastasis [Citation8], lung adenocarcinoma (LUAD) [Citation9] and meningioma [Citation10]. However, its roles and mechanisms of action in AML are unknown.
ZEB2 has been shown to be involved in AML pathogenesis [Citation11] and online bioinformatics databases indicate that miR-454-3p targets ZEB2. In this work, we confirmed that miR-454-3p is downregulated in AML cells and regulates their growth, apoptosis and autophagy. Mechanistically, miR-454-3p targeted ZEB2 and acted as a tumor suppresser in AML. Collectively, the current research suggests that miR-454-3p may be a novel molecular target for AML with therapeutic implications.
2. Materials and methods
2.1. Cell culture
NB4, THP-1, KG-1a, U937 and 293 T cell lines were maintained in our laboratory and peripheral blood mononuclear cells (PBMCs) were isolated from healthy controls. AML and U937 cells were cultivated in RPMI-1640 medium (Gibco, USA) containing 1% antibiotics (Beyotime, China) and 10% fetal bovine serum (FBS, USA). 293 T cells were cultivated in DMEM medium (Gibco, USA) with 10% fetal calf serum (FBS, USA). All cells were maintained at 37°C and 5% CO2.
2.2. PBMC isolation
Anticoagulated blood was taken from healthy donors using vacuum blood collection tubes, carefully added to the liquid surface of FICOLL solution (Biolab, Beijing, China), and centrifuged for 30 min at 600 × g. The mononuclear cell layer was aspirated and the cell pellet was rinsed 3 times with PBS and used for RNA extraction.
2.3. Real-time quantitative PCR
RNA extraction was performed using TRIZOL (Takara, Japan) and reverse transcription with PrimeScript RT Kit (Takara). RT-qPCR was carried out on a CFX Connect RT–PCR system (Bio-Rad, USA). The 2−ΔΔCt method was employed for measuring gene expression. All primer pairs () were designed and produced by Sangon Biotech (Shanghai, China).
Table 1. Primer sequence.
2.4. miR-454-3p overexpression and knockdown
Cells were grown in 6-well plates until 60% confluency. 5 µL Lipofectamine 3000 (Invitrogen, USA) was added to achieve transfection with 5 nM miR-454-3p mimic, negative control (NC) or 10 nM miR-454-3p inhibitor or inhibitor NC (RiboBio, Guangzhou, China). Further analysis was undertaken 48–72 h after transfection. The sequences of miR-454-3p mimic and inhibitor were listed in .
Table 2. mimic and inhibitor sequences.
2.5. ZEB2 knockdown
THP-1 cells were grown in 24-well plates. Lentiviral vectors containing ZEB2 or NC were synthesized by GENECHEM (Shanghai, China) and employed to create ZEB2 stably-silenced cell lines. Intense fluorescence was detected under a fluorescence microscope after 72 h incubation and 5 μg/mL puromycin (Abcam, UK) was added for 2 weeks to select stable knockdown cell lines.
2.6. Western blotting (WB)
Cells were harvested and washed at least 3 times with pre-cooled PBS, lysed with RIPA buffer (no. P0013; Beyotime) mixed with PMSF (100:1; Cell Signaling Technology, USA), vortexed 3 times for 10 min each and centrifuged at 13000 x g for 30 min at 4°C. Protein content was determined with BCA Protein Assay Kit (P0012; Beyotime) and supernatants boiled in 5 × loading buffer solution. Subsequently, equal volumes of protein were elecrophoresed on 8% and 10–15% SDS-polyacrylamide gels and transferred to the PVDF membrane. Membranes were blocked with TBST with 5% non-fat milk for 2–3 h, incubated for 8–16 h at 4°C with primary antibodies and for 1 h with HRP-labeled secondary antibodies (1:4000; Biosharp, China). Protein blots were detected using the ECL Substrate kit (WBULS0100; EMD Millipore, USA). Primary antibodies (1:1000) were as follows: β-actin (Boster, USA), ZEB2 (HUABIO, Hangzhou, China), p62 (Bimake, USA), ATG12 (Bimake), LC3 (CST, USA), cyclin B1 (CST, USA), p21 (CST, USA), cyclin D1 (WanLeibio, Shenyang, China), cleaved PARP (Abcam, Cambridge, UK), cleaved caspase 9 (CST), bcl-2 (Abcam), bax (Bimake), AKT (CST), m-TOR (CST), p-AKT (CST) and p-mTOR (CST). 3-MA was obtained from Selleck.
2.7. CCK-8 assay
Cells were plated at 3000–5000 cells/well in a volume of 100 μL. 10 μL CCK-8 reagent from the CCK-8 kit (MCE, USA) was added with incubation for 0, 24, 48, 72 and 96 h and absorbance read by microplate reader after 3 h incubation at 37°C, 5% CO2. Five replicates were examined for each experimental group.
2.8. Immunofluorescence (IF)
Cells were rinsed 3 times with PBS, pre-cooled 4% paraformaldehyde (Beyotime) added with incubation at room temperature for 15 min, permeabilized with 0.1% Triton X-100 (Beyotime) for 15 min at 37°C and incubated with 10% BSA for 1 h. Cells were smeared onto slides and exposed to rabbit anti-human LC3 primary antibody (1:100, Abcam, UK) at 4°C overnight and rabbit Alexa™ Fluor 488 (1:200; Abcam, UK) secondary antibody at 37°C for 1 h. DAPI (1:1000; Beyotime) was utilized to visualize nuclear staining and fluorescence detected by Laser Scanning Confocal Microscope.
2.9. Flow cytometry
Cell cycle stage and apoptosis were determined by flow cytometric analysis. One million cells were harvested and rinsed 3 times with PBS to evaluate cell cycle stage and cell apoptosis, which were measured by CytoFLEX flow cytometer (Beckman, USA). Annexin V-FITC/PI kit (Elabscience, Wuhan) was applied to evaluate apoptotic cells. To analyze the cell cycle, cells were resuspended in 900 μL pre-cooled 75% ethanol and mixed with 100 μL PBS, followed by overnight fixation at 4°C for assessment of cell cycle stage by cytometer. CytExpert software was used for data acquisition.
2.10. Colony formation assay
Cell lines transfected with sh-ZEB2 or sh-NC were suspended in 100 μL medium, inoculated into a 96-well plate at a density of 50–100 cells/well and grown for 7 days at 37°C, 5% CO2 before colonies were counted under a microscope.
2.11. Luciferase reporter assays
The potential binding site of miR-454-3p in human ZEB2 was selected from the overlapping predicted targets in STARBASE and TargetScan. Oligonucleotides, pMIR-ZEB2-3′UTR-wt and -mut, were synthesized by GeneCreate (Wuhan, China), and lipofectamine 2000 (Invitrogen, USA) was utilized to co-transfect luciferase reporter plasmid and miR-454-3p mimic/mimic-NC into 293 T cells. Cells were cultured at 37°C, 5% CO2 for 2 days, rinsed 3 times with PBS and Dual-Luciferase Reporter (DLR) System Kit used to determine Renilla and firefly luciferase activities (Promega, WI, USA).
2.12. Statistical analysis
All values are presented as mean ± SD. Prism v8.0 was used for all statistical analyses. Comparisons between 2 groups were made by Student’s unpaired t test. A value of p < 0.05 was considered to indicate statistical significance and levels of significance are marked as follows: ****p ≤ 0.0001, ***p ≤ 0.001, **p ≤ 0.01, and *p ≤ 0.05.
3. Results
3.1. miR-454-3p was downregulated in AML cell lines and overexpression led to growth inhibition and G2/M arrest
MiR-454-3p has previously been reported to be downregulated in diverse tumors [Citation12–14] but no functional role in AML has been recorded. Inspired by these findings, miR-454-3p expression was measured by RT-qPCR in 4 commonly used AML cell lines and compared with that in PBMCs. It was noted that miR-454-3p expression was much lower in the AML cells relative to PBMCs (a) and higher in U937 and THP-1 cells than in other cell lines. To ensure better visibility of the impact of knocking miR-454-3p down, the THP-1 cell line was selected for the following studies.
Figure 1. Effects of miR-454-3p overexpression and knockdown on THP-1 cell growth and cell cycle stage. miR-454-3p levels in KG1a, THP-1, NB4, U937 cells and PBMCs were assessed by RT-qPCR (a). Assessment of transfection efficiency of miR-454-3p mimic and inhibitor by RT-qPCR (b). Cell viability was examined by CCK-8 assay (c). Flow cytometric and WB analyses were conducted to assess cell cycle stage (d and e). *p < 0.05, **p < 0.01, ***p < 0.001, ****p < 0.0001.
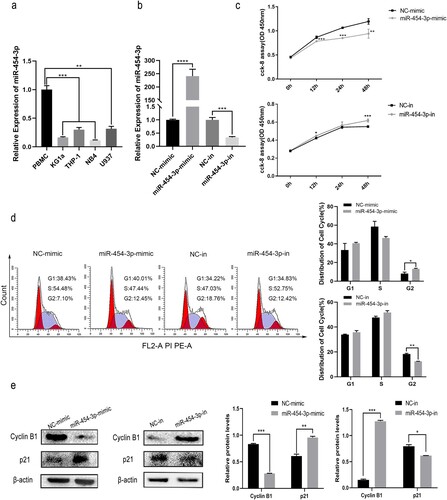
In the first experiments, RT-qPCR showed that miR-454-3p mimic/inhibitor-transfected THP-1 cells over- or under-express miR-454-3p (b). Next, cell viability was reduced by miR-454-3p over-expression and elevated by miR-454-3p inhibition, as shown by CCK-8 assays (c). Furthermore, cell cycle stages as determined by flow cytometry and WB revealed that miR-454-3p overexpression increased the cell fraction arrested in G2/M, the expression of cell cycle-related protein, cyclin B1 downregulated and p21 increased by miR-454-3p overexpression. MiR-454-3p knockdown produced the opposite effect (d and e). Altogether, these findings demonstrate that miR-454-3p attenuated AML cell proliferation, and a tumor inhibitor role was indicated.
3.2. miR-454-3p overexpression induced AML cell apoptosis and autophagy
To examine the functions of miR-454-3p in AML cells, apoptosis and autophagy were investigated using the THP-1 cell line. MiR-454-3p overexpression upregulated levels of the apoptotic proteins, bax/cleaved-PARP, and downregulated the anti-apoptotic protein, BCL2, in THP-1 cells, as shown by WB. By contrast, bax/cleaved-PARP expression showed a decrease and BCL2 an increase after miR-454-3p knockdown (a). Flow cytometry produced similar results (b).
Figure 2. Effects of miR-454-3p overexpression and knockdown on THP-1 cell apoptosis and autophagy. WB and flow cytometry were conducted to determine the apoptosis of THP-1 cells (a and b). Expression of autophagy-associated proteins, p62 and LC3, in THP-1 was detected by WB (c). GFP-LC3 expression in THP-1 cells was measured by immunofluorescence (d). *p < 0.05, **p < 0.01, ***p < 0.001.
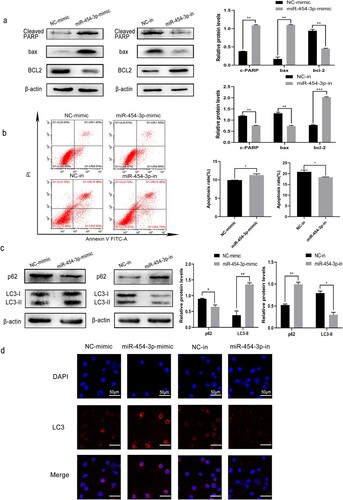
Autophagy is a form of programd cell death which slows cancer progression during the early stages [Citation15] and is achieved via numerous autophagy-related (ATG) proteins. SQSTM1/p62 is a prototype autophagy receptor which accumulates when autophagy pathways are disturbed and degradation of ATGs is impaired [Citation16]. The transformation of LC3-I to LC3-II is generally employed to appraise autophagy [Citation17]. Therefore, to explore whether miR-454-3p affects autophagy in AML cells, we quantified LC3, ATG12 and P62 in THP-1. Distinct accumulation of LC3-Ⅱ and ATG12 was detected in THP-1 cells when miR-454-3p was overexpressed, accompanied by decreased p62 (c). Furthermore, under miR-454-3p overexpression, THP-1 cells expressing GFP-LC3 were observed by laser scanning confocal microscopy in order to detect autophagosomes. GFP-LC3 became more difficult to detect after knocking down miR-454-3p (d). Hence, it can be inferred that miR-454-3p promotes AML cell apoptosis and autophagy, and that its knockdown facilitates their apoptosis and autophagy.
3.3. miR-454-3p targets on ZEB2
ZEB2 has been described as a tumor promoter, contributing to poor prognosis in several human cancers [Citation18,Citation19] and was identified as a suitable target from the bioinformatics databases, TargetScan 7.2, miRDB and starBase v2.0. ZEB2 and miR-454-3p were found to possess analogous binding sites (a), implying that ZEB2 may be a target of miR-454-3p. DLR assays showed decreased luciferase activities of ZEB2-3’UTR-WT and miR-454-3p-mimic co-transfected 293 T cells (b). Moreover, RT-qPCR data indicated suppression of ZEB2 expression with miR-454-3p overexpression and elevation of ZEB2 expression with miR-454-3p knockdown (c). WB experiments produced similar results (d). In conclusion, ZEB2 appears to be a downstream target of miR-454-3p.
Figure 3. ZEB2 was targeted by miR-454-3p. Interaction between ZEB2 and miR-454-3p was predicted using the databases, starBase v2.0, miRDB and TargetScan 7.2 (a). Dual-luciferase reporter assays of miR-454-3p-mimic and NC-mimic co-transfected with ZEB2-3’UTR-mut or ZEB2-3’UTR-wt in 293 T cells (b). Protein and mRNA levels of ZEB2 after transfection with miR-454-3p mimic or inhibitor were detected by WB and RT-qPCR in THP-1 cells (c and d). *p < 0.05, **p < 0.01, ***p < 0.001. n.s., not significant.
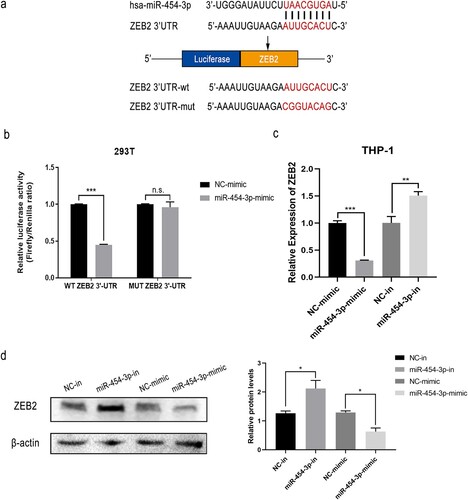
3.4. ZEB2 was upregulated in AML cell lines and ZEB2 knockdown inhibited growth and caused G0/G1 arrest
Based on the above findings, ZEB2 was selected for further study. First, to examine the expression level of ZEB2 in AML cells, GEPIA was used to collect and analyze data on ZEB2 expression from The Cancer Genome Atlas (TCGA) and showed increased levels in 172 AML specimens relative to normal controls (n = 70) (a and b). Next, ZEB2 expression was quantified in 4 AML cell lines with PBMCs as negative control. RT-qPCR analysis showed overexpression of ZEB2 in AML cell lines relative to PBMCs (c). These findings demonstrate that ZEB2 may be associated with AML progression and with poor prognosis.
Figure 4. ZEB2 knockdown was associated with cell cycle arrest and cell proliferation in AML. Expression of ZEB2 in normal and AML samples from the database GEPIA (a and b). The expression of ZEB2 in KG1a, THP-1, NB4, U937 cells and PBMCs was assessed by RT-qPCR (c). Knockdown efficiency of sh-ZEB2-1 and sh-ZEB2-2 was verified by RT-qPCR and WB (d and e). Colony formation assays and CCK-8 assays were utilized to measure cell growth (f and g). WB and flow cytometry were conducted to assess cell cycle stage. (h and i). *p < 0.05, **p < 0.01, ***p < 0.001.
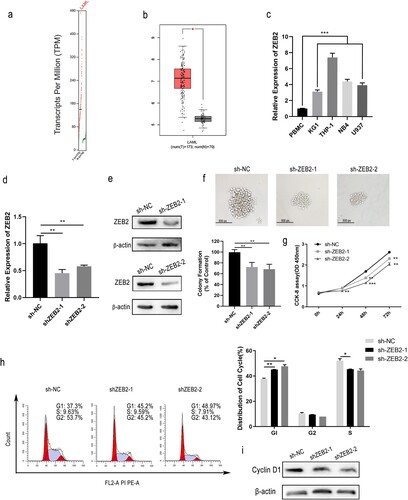
Given that THP-1 has the most pronounced overexpression of ZEB2 in the cell lines tested, we continued to use it as a representative cell line for AML. We succeeded in obtaining stable knockdown of ZEB2 in this line by lentiviral transfection. The knockdown efficiency of ZEB2 was assessed by WB and RT-qPCR (d and 4e). ZEB2 knockdown reduced THP-1 proliferation, as shown by colony formation and CCK-8 assays (f and 4g), and caused arrest in the G0/G1 phase, as shown by flow cytometry (h) due to downregulation of cell cycle-related protein, cyclin D1 (i). To our surprise, ZEB2 knockdown caused arrest at a different stage in the cell cycle from that caused by miR-454-3p overexpression. ZEB2 thus appears to have a beneficial function for AML cell growth.
3.5. ZEB2 knockdown promoted apoptosis induced by autophagy in AML cell lines
To validate the role of ZEB2 in regulating AML, autophagy and apoptosis were examined in THP-1 cells with stable ZEB2 knockdown. Flow cytometry of THP-1 cells with stable ZEB2 knockdown revealed increased apoptosis with elevated levels of cleaved PARP, cleaved caspase 9 and bax, and decreased BCL2 (a and 5b). ZEB2 knockdown also increased autophagy in THP-1 cells (c and 5d). The function of ZEB2 seems to be linked to the promotion of AML.
Figure 5. ZEB2 knockdown regulated apoptosis and autophagy in AML. WB and flow cytometry were carried out to detect the apoptosis of THP-1 cells (a and b). GFP-LC3 expression was detected by immunofluorescence (c). The autophagy-associated proteins, p62, ATG12 and LC3, in THP-1 were detected by WB (d). Levels of autophagy-related and apoptosis-related proteins were determined by WB with or without 5 mM 3-MA added to ZEB2 knockdown cells (e). Modulation of ZEB2 partially reversed miR-454-3p-regulated cellular processes in THP-1 cells. Rescue assays of apoptosis and autophagy after co-transfection with miR-454-3p-inhibitor and sh-ZEB2, at the protein level (f). Transfection of THP-1 cells with miR-454-3p-inhibitor or mimic was examined by WB for levels of autophagy signaling pathway proteins (g). *p < 0.05, **p < 0.01, ***p < 0.001. n.s., not significant.
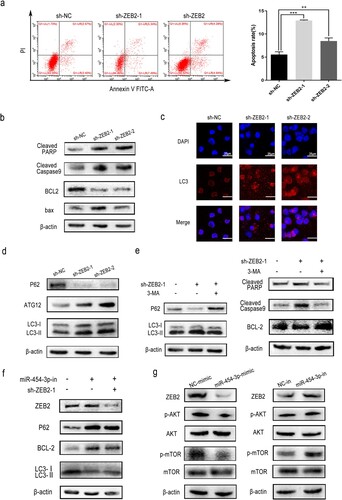
LC3 accumulation has been previously linked to the inhibition of ATG protein degradation or the initiation of autophagy flux [Citation20]. PtdIns3K activity is essential to autophagosome formation [Citation21] and the PtdIns3K inhibitor, 3-MA, was used in follow-up studies [Citation22]. 3-MA reversed the increase in LC3-II/LC3-I protein levels and the decrease in p62 protein caused by ZEB2 knockdown.
Apoptosis and autophagy are two types of programd cell death triggered by common upstream signals with complex functional interrelationships. Autophagy has been shown to suppress apoptosis and represents a stress adaptation to avoid cell death under certain conditions [Citation23]. Intrestingly, under different conditions, autophagy may stimulate apoptosis [Citation24,Citation25]. In our study, ZEB2 was found to participate in the suppression of apoptosis and autophagy. Simultaneously, we questioned if ZEB2 knockdown could trigger apoptosis in AML cells via enhancing autophagy in our research. Exposure of ZEB2-knockdown THP-1 cells to 3-MA produced decreased levels of apoptosis-associated proteins (e). Thus, autophagy appears to induce apoptosis in the system used during the current study. ZEB2 knockdown promoted apoptosis by enhancing autophagic activity in the AML cell-line.
3.6. miR-454-3p induced apoptosis and autophagy in AML via downregulation of ZEB2 expression and inhibition of the AKT/mTOR pathway
To further confirm that miR-454-3p targets ZEB2 to regulate AML development, rescue assays were performed and indicated that the impact of miR-454-3p downregulation on THP-1 cells could be reversed by ZEB2 inhibition at the protein level (f). In addition, AKT/mTOR is regarded as a classical autophagy pathway, and upregulation of AKT/mTOR, has been recently reported in AML and its inhibition suggested as a therapeutic approach [Citation26]. Inhibition of AKT/mTOR signaling by miR-454 has also been demonstrated in ovarian cancer cells [Citation27]. WB experiments showed that miR-454-3p inhibition resulted in elevated p-AKT/p-mTOR in THP-1 cells and miR-454-3p overexpression produced the reverse effect. Total AKT and mTOR levels remained unchanged in both cases (g). In summary, miR-454-3p targeted ZEB2 through inhibition of the AKT/mTOR pathway to increase apoptosis and autophagy in AML cells.
4. Discussion
MiRNAs have previously been indicated to be useful tools for cancer diagnosis and prognosis and may have oncogenic or tumor-suppressive roles [Citation28]. miR-454-3p has been implicated in the occurrence and progression of a variety of tumors [Citation29–31] but has an unclear relevance to AML. The current study found miR-454-3p to be downregulated in AML cells and to block AML tumor development. miR-454-3p suppressed the growth of AML cell-lines and promoted apoptosis and autophagy via targeting of ZEB2.
miRNAs usually interact with the 3'UTR of target genes but may also bind to 5’ UTR, gene promoter or coding sequences [Citation32]. Possible downstream targets of miR-454-3p were investigated through bioinformatics analysis and ZEB2 was finally filtered out as a target. ZEB2 is a transcription factor involved in AML carcinogenesis [Citation33]. Moreover, DLR assays indicated ZEB2 to be a potential target of miR-454-3p which merits further study.
According to our results, ZEB2 was upregulated in AML cells relative to PBMCs and its expression was inversely related to that of miR-454-3p, supporting the view that ZEB2 can be targeted by miR-454-3p to regulate AML progression. ZEB2 knockdown suppressed AML cell growth, by contrast with miR-454-3p knockdown. The opposite influences of ZEB2 and miR-454-3p on cell growth, apoptosis and autophagy confirmed the negative regulation hypothesis. However, ZEB2 knockdown induced a different stage of cell cycle arrest from that caused by miR-454-3p overexpression which was contrary to our expectations. This situation may arise due to the complexity of cell cycle progression, an area which needs to be further explored.
Autophagy is generally regarded as cellular self-digestion by regulating protein and organelle degradation [Citation34]. To verify the mechanisms underlying the effect of ZEB2 downregulation on AML cell autophagy, we applied the autophagy inhibitor 3-MA, which prevents the formation of autophagosomes. Pretreatment of ZEB2-knockdown THP-1 cells with 3-MA, which prevents autophagosome formation, reduced levels of LC3-II protein and increased those of p62. The finding that ZEB2 knockdown reduces AML cell autophagy by regulating the formation of autophagosomes is a novel one. High rates of autophagy were found to stimulate apoptosis indicating the intricate relationship between these two processes. Macrophage autophagy has previously been found to suppress apoptosis caused by lipid accretion during atherosclerosis [Citation35] but autophagy has also been reported to accelerate the apoptotic removal of damaged cells [Citation36]. The current work found that ZEB2 knockdown induced apoptosis via enhancing the autophagic activity of AML cells.
In conclusion, miR-454-3p regulated AML development via inhibition of the ZEB2/AKT/mTOR axis. AKT/mTOR signaling cascades are known to be essential for autophagy regulation [Citation37]. Mutational activation of the PI3K/AKT/mTOR axis has been found in many clinical samples of AML [Citation38]. MiR-454-3p overexpression was demonstrated to reduce p-AKT and p-mTOR protein levels in the AML cells of the current study and rescue assays confirmed the effects of ZEB2 knockdown reversing miR-454-3p at the protein level.
5. Conclusion
In summary, new insights into the pathophysiological role of miR-454-3p in AML are presented. MiR-454-3p inhibited AML cell growth and promoted apoptosis and autophagy through the ZEB2/AKT/mTOR axis. MiR-454-3p shows promise as a molecular target for AML treatment.
Compliance with ethical standards
Ethical approval
All procedures involving human participants were performed in accordance with the ethical standards of the institutional and/or national research committees and with the 1964 Helsinki declaration and its later amendments or comparable ethical standards.
Informed consent
Informed consent was obtained from all participants.
Acknowledgements
The authors would like to express their gratitude to EditSprings (https://www.editsprings.cn/) for the expert linguistic services provided.
Disclosure statement
No potential conflict of interest was reported by the author(s).
Additional information
Funding
References
- Thomas D, Majeti R. Biology and relevance of human acute myeloid leukemia stem cells. Blood. 2017;129:1577–1585. doi:10.1182/blood-2016-10-696054
- Chen X, Xie H, Wood BL, et al. Relation of clinical response and minimal residual disease and their prognostic impact on outcome in acute myeloid leukemia. J Clin Oncol. 2015;33:1258–1264. doi:10.1200/JCO.2014.58.3518
- Salvatore D, Labopin M, Ruggeri A, et al. Outcomes of hematopoietic stem cell transplantation from unmanipulated haploidentical versus matched sibling donor in patients with acute myeloid leukemia in first complete remission with intermediate or high-risk cytogenetics: a study from the acute leukemia working party of the European society for blood and marrow transplantation. Haematologica. 2018;103:1317–1328. doi:10.3324/haematol.2018.189258
- Volinia S, Calin GA, Liu CG, et al. A microRNA expression signature of human solid tumors defines cancer gene targets. P Natl Acad Sci. 2006;103:2257–2261. doi:10.1073/pnas.0510565103
- Calin GA, Croce CM. MicroRNA signatures in human cancers. Nat Reviews Cancer. 2006;6:857–866. doi:10.1038/nrc1997
- Shah NM, Zaitseva L, Bowles KM, et al. NRF2-driven miR-125B1 and miR-29B1 transcriptional regulation controls a novel anti-apoptotic miRNA regulatory network for AML survival. Cell Death Differ. 2015;22:654–664. doi:10.1038/cdd.2014.152
- Liu Y, Lei P, Qiao H, et al. miR-9 enhances the chemosensitivity of AML cells to daunorubicin by targeting the EIF5A2/MCL-1 axis. Int J Biol Sci. 2019;15:579–586. doi:10.7150/ijbs.29775
- Ren L, Chen H, Song J, et al. MiR-454-3p-Mediated Wnt/β-catenin signaling antagonists suppression promotes breast cancer metastasis. Theranostics. 2019;9:449–465. doi:10.7150/thno.29055
- Zhao X, Li X, Zhou L, et al. LncRNA HOXA11-AS drives cisplatin resistance of human LUAD cells via modulating miR-454-3p/Stat3. Cancer Sci. 2018;109:3068–3079. doi:10.1111/cas.13764
- Ghafouri-Fard S, Abak A, Hussen BM, et al. The emerging role of Non-coding RNAs in pituitary gland tumors and meningioma. Cancers. 2021;13; doi:10.3390/cancers13235987
- Li H, Mar BG, Zhang H, et al. The EMT regulator ZEB2 is a novel dependency of human and murine acute myeloid leukemia. Blood. 2017;129:497–508. doi:10.1182/blood-2016-05-714493
- Liao H, Liang Y, Kang L, et al. miR-454-3p inhibits non-small cell lung cancer cell proliferation and metastasis by targeting TGFB2. Oncol Rep. 2021;45; doi:10.3892/or.2021.8018
- Zuo J, Yu H, Xie P, et al. miR-454-3p exerts tumor-suppressive functions by down-regulation of NFATc2 in glioblastoma. Gene. 2019;710:233–239. doi:10.1016/j.gene.2019.06.008
- Hui X, Zhang S, Wang Y. miR-454-3p suppresses cell migration and invasion by targeting CPEB1 in human glioblastoma. Mol Med Rep. 2018;18:3965–3972. doi:10.3892/mmr.2018.9386
- Li X, He S, Ma B. Autophagy and autophagy-related proteins in cancer. Mol Cancer. 2020;19:12. doi:10.1186/s12943-020-1138-4
- Deng Z, Lim J, Wang Q, et al. ALS-FTLD-linked mutations of SQSTM1/p62 disrupt selective autophagy and NFE2L2/NRF2 anti-oxidative stress pathway. Autophagy. 2020;16:917–931. doi:10.1080/15548627.2019.1644076
- Klionsky DJ, Abdalla FC, Abeliovich H, et al. Guidelines for the use and interpretation of assays for monitoring autophagy. Autophagy. 2012;8:445–544. doi:10.4161/auto.19496
- Li Y, Fei H, Lin Q, et al. ZEB2 facilitates peritoneal metastasis by regulating the invasiveness and tumorigenesis of cancer stem-like cells in high-grade serous ovarian cancers. Oncogene. 2021;40:5131–5141. doi:10.1038/s41388-021-01913-3
- Kahlert C, Lahes S, Radhakrishnan P, et al. Overexpression of ZEB2 at the invasion front of colorectal cancer is an independent prognostic marker and regulates tumor invasion in vitro. Clin Cancer Res. 2011;17:7654–7663. doi:10.1158/1078-0432.CCR-10-2816
- Sun M, Wang S, Jiang L, et al. Patulin induces autophagy-dependent apoptosis through lysosomal-mitochondrial axis and impaired mitophagy in HepG2 cells. J Agric Food Chem. 2018;66:12376–12384. doi:10.1021/acs.jafc.8b03922
- Bernard M, Yang B, Migneault F, et al. Autophagy drives fibroblast senescence through MTORC2 regulation. Autophagy. 2020;16:2004–2016. doi:10.1080/15548627.2020.1713640
- Wu YT, Tan HL, Shui G, et al. Dual role of 3-methyladenine in modulation of autophagy via different temporal patterns of inhibition on class I and III phosphoinositide 3-kinase. J Biol Chem. 2010;285:10850–10861. doi:10.1074/jbc.M109.080796
- Maiuri MC, Zalckvar E, Kimchi A, et al. Self-eating and self-killing: crosstalk between autophagy and apoptosis. Nat Rev Mol Cell Biol. 2007;8(9):741–752. doi:10.1038/nrm2239
- Wang K. Autophagy and apoptosis in liver injury. Cell Cycle. 2015;14(11):1631–1642. doi:10.1080/15384101.2015.1038685
- Singla S, Iwamoto-Stohl LK, Zhu M, et al. Autophagy-mediated apoptosis eliminates aneuploid cells in a mouse model of chromosome mosaicism. Nat Commun. 2020;11:2958. doi:10.1038/s41467-020-16796-3
- Bertacchini J, Heidari N, Mediani L, et al. Targeting PI3K/AKT/mTOR network for treatment of leukemia. Cell Mol Life Sci. 2015;72:2337–2347. doi:10.1007/s00018-015-1867-5
- An Y, Zhang J, Cheng X, et al. miR-454 suppresses the proliferation and invasion of ovarian cancer by targeting E2F6. Cancer Cell Int. 2020;20:237. doi:10.1186/s12935-020-01300-0
- Lee YS, Dutta A. MicroRNAs in cancer. Annu Rev Pathol. 2009;4:199–227. doi:10.1146/annurev.pathol.4.110807.092222
- Qian XL, Zhou F, Xu S, et al. MiR-454-3p promotes oxaliplatin resistance by targeting PTEN in colorectal cancer. Front Oncol. 2021;11:638537. doi:10.3389/fonc.2021.638537
- Bao X, Ren T, Huang Y, et al. Knockdown of long non-coding RNA HOTAIR increases miR-454-3p by targeting Stat3 and Atg12 to inhibit chondrosarcoma growth. Cell Death Dis. 2017;8:e2605. doi:10.1038/cddis.2017.31
- Shao N, Xue L, Wang R, et al. miR-454-3p is an exosomal biomarker and functions as a tumor suppressor in glioma. Mol Cancer Ther. 2019;18:459–469. doi:10.1158/1535-7163.Mct-18-0725
- O'Brien J, Hayder H, Zayed Y, et al. Overview of MicroRNA biogenesis, mechanisms of actions, and circulation. Front Endocr. 2018;9:402. doi:10.3389/fendo.2018.00402
- Bolouri H, Farrar JE, Triche T, Jr. et al. The molecular landscape of pediatric acute myeloid leukemia reveals recurrent structural alterations and age-specific mutational interactions. Nat Med. 2018;24:103–112. doi:10.1038/nm.4439
- Gu HF, Li HZ, Tang YL, et al. Nicotinate-Curcumin impedes foam cell formation from THP-1 cells through restoring autophagy flux. PloS one. 2016;11:e0154820. doi:10.1371/journal.pone.0154820
- Zhou P, Xie W, Luo Y, et al. Inhibitory effects of ginsenoside Rb1 on early atherosclerosis in ApoE-/- Mice via inhibition of apoptosis and enhancing autophagy. Molecules-Basel, Switzerland. 2018;23; doi:10.3390/molecules23112912
- Tashiro SQ, Onodera S, Minami M, et al. Autophagy preceded apoptosis in oridonin-treated human breast cancer MCF-7 cells. Biol Pharm Bull. 2007;30:859–864. doi:10.1248/bpb.30.859
- Fang S, Wan X, Zou X, et al. Arsenic trioxide induces macrophage autophagy and atheroprotection by regulating ROS-dependent TFEB nuclear translocation and AKT/mTOR pathway. Cell Death Dis. 2021;12:88. doi:10.1038/s41419-020-03357-1
- Estruch M, Reckzeh K, Vittori C, et al. Targeted inhibition of cooperative mutation- and therapy-induced AKT activation in AML effectively enhances response to chemotherapy. Leukemia. 2021;35:2030–2042. doi:10.1038/s41375-020-01094-0